Atomic Clocks: Precision, Challenges, and Future Prospects
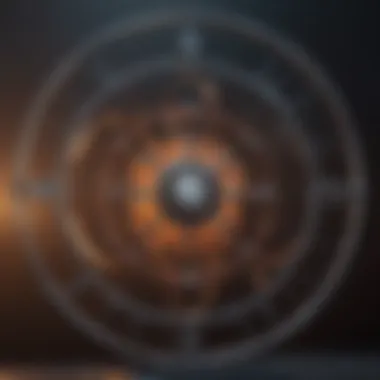
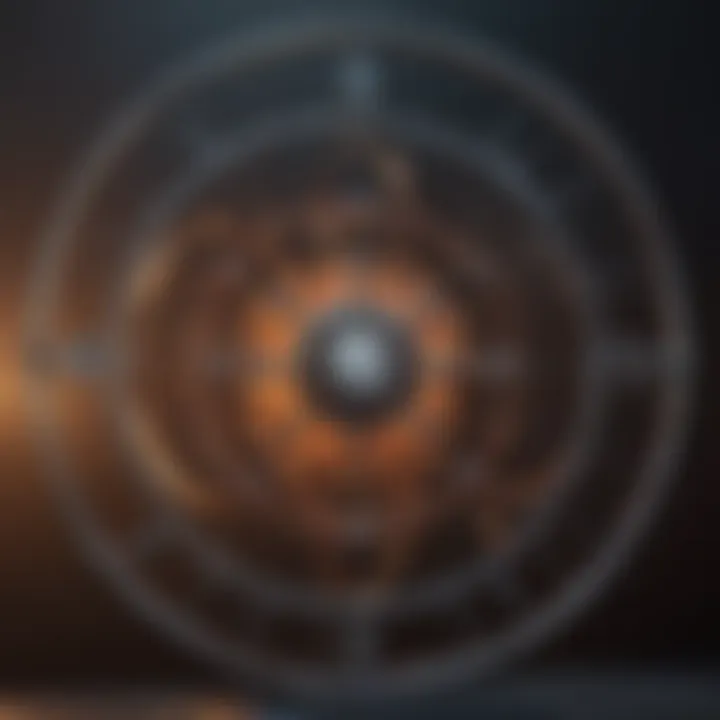
Intro
In a world that demands impeccable precision, atomic clocks have emerged as the unsung heroes of timekeeping. Their accuracy surpasses traditional methods by leaps and bounds, making them essential in a variety of fields, including navigation, telecommunications, and even fundamental research in physics. This article aims to unpack the technology behind atomic clocks, the significance of their accuracy, and the latest advancements in this revolutionary field.
Atomic clocks operate on the principle of vibrations of atoms; they essentially count the ticks of these atoms as they transition between energy levels under the influence of microwave radiation. Such workings may sound complex, but at their core, atomic clocks hold the potential to redefine our understanding of time itself.
Diving deeper into this topic reveals both the implications of atomic clock technology and its ongoing evolution. Let's explore the recent advances that are shaping this field.
Prologue to Atomic Clocks
Understanding atomic clocks is not just about grasping a complex technological marvel; it’s about realizing the bedrock of modern timekeeping. These precision instruments have fundamentally altered our grasp of time and its measurement. Take a moment to think about how critical accurate timekeeping is in our lives—from GPS navigation to telecommunications. The advancements in atomic clocks are not only a leap in scientific achievement but also a vital component that supports societal functions, technology, and scientific research.
When discussing atomic clocks, there are key elements to consider. First, the precision they offer is unmatched, with differences as minute as billionths of a second playing a role in syncing global networks and satellite systems. Second, the historical journey of these clocks speaks volumes about humanity's relentless pursuit of accuracy. Through phases of scientific exploration and technological enhancements, we have reached a point where the significance of atomic clocks gleams radiant in various fields—from astrophysics to quantum computing.
The beauty of atomic clocks lies also in their relationship with quantum mechanics, a relationship that illustrates the harmonious dance between theory and practical application. Without quantum mechanics, atomic clocks would be mere curiosities rather than the essential tools they are today. Therefore, the journey into the domain of atomic clocks is one of discovery and innovation, weaving together the multitude of scientific disciplines that make our understanding of time profound.
Dedicated research in this area continues to help us explore uncharted territories. This article aims to shed light on the depths of atomic clock technology, highlighting both its significance and advancements. From understanding the principles behind these clocks to assessing their future potential, the exploration is substantial, interesting, and highly relevant for students, researchers, educators, and professionals alike.
Principles Behind Atomic Clocks
Understanding the principles behind atomic clocks is essential to grasping their role in modern technology. Atomic clocks operate based on the precise frequencies of electromagnetic radiation absorbed or emitted by atoms, particularly during transitions between energy levels. This precision in measuring time hinges on the ability to accurately count cycles of this electromagnetic radiation. With timekeeping being fundamental to various sectors — from GPS navigation systems to telecommunications — the knowledge of atomic clock principles is indispensable.
Atomic Transitions
Atomic transitions are the phenomenon where electrons move between energy levels within an atom when they absorb or emit electromagnetic radiation. Specifically, in cesium atomic clocks, the transition between two hyperfine levels of the cesium-133 atom forms the basis for timekeeping.
It's interesting to note that these transitions are remarkably stable. The cesium standard is so precise that a second, defined as 9,192,631,770 cycles of microwave radiation corresponding to this transition, showcases the unique stability of atomic clocks. The implications of atomic transitions extend beyond mere timekeeping; they help to synchronize clocks globally, enabling a multitude of technologies to operate seamlessly.
Frequency Standards
Definition of Frequency Standards
Frequency standards serve as benchmarks for measuring time. In atomic clocks, these standards are defined by the consistent frequencies of atomic transitions. This definitional clarity is pivotal for maintaining uniformity in timekeeping.
The key characteristic of frequency standards lies in their stability and reproducibility, which renders them advantageous in creating accurate clocks. A notable attribute of these standards is that they incorporate quantum mechanics, leveraging principles that ensure a minimal margin of error. Their unique feature is that they allow scientists to achieve levels of precision — on the order of one second in millions of years — making them a critical element in enhancing the accuracy of atomic clocks.
Importance in Timekeeping
The importance of frequency standards cannot be overstated; they are indeed the backbone of modern timekeeping methods. These standards dictate how atomic clocks measure time, ensuring that they do so with unparalleled accuracy.
An essential aspect of their importance is their broad applicability. For instance, frequency standards not only stabilize the operations of atomic clocks but also underpin the synchronization necessary for devices like GPS satellites and mobile communication networks. A unique feature worth mentioning is that the choice of atomic species — like cesium or rubidium — influences the frequency standard's performance. This choice can have both advantages and disadvantages, as varying atomic properties lead to differences in precision and stability across clock technologies.
In summary, principles behind atomic clocks, especially through atomic transitions and frequency standards, highlight a sophisticated interplay of quantum mechanics and engineering precision. This combination supports not only the mechanisms of accurate timekeeping but also emerges as crucial in the broader context of our increasingly connected and fast-paced world.
The intricate dance of atoms and their transitions offers insight into the fundamental nature of time itself, demonstrating the convergence of science, technology, and our everyday lives.
Types of Atomic Clocks
Understanding the various types of atomic clocks provides insight into their capabilities and applications in our modern world. Each type is grounded in the same principles of atomic theory but diverges in its execution and technological demands. This variety allows for specific enhancements in timekeeping, catering to different needs and environments.
Cesium Atomic Clocks
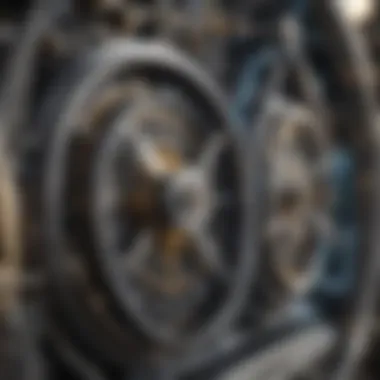
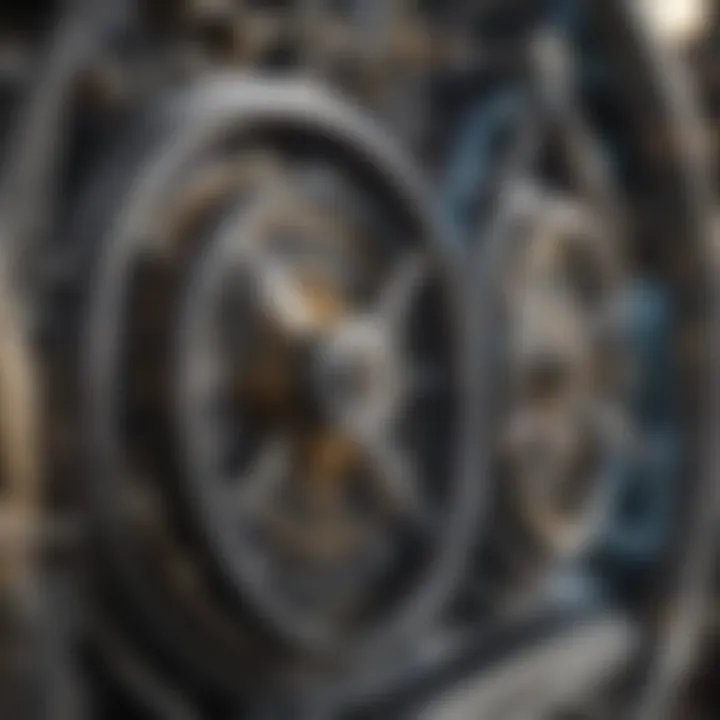
Cesium atomic clocks are the cornerstone of timekeeping precision since their acceptance as the standard by the International System of Units (SI) in the late 20th century. These clocks operate based on the transitions of cesium atoms between energy states, measured at a frequency of 9,192,631,770 hertz. This frequency defines the second, emphasizing the profound impact cesium clocks have on global timing standards.
The robustness of cesium clocks lies in their stability and reliability, making them essential for scientific research and telecommunications. Their widespread use in navigation systems and internet protocols showcases their importance in daily life. However, they do come with limitations, notably in their size and cost, which might not suit every application. Still, they remain a benchmark against which other atomic clock technologies are measured.
Rubidium Atomic Clocks
Rubidium atomic clocks offer a compelling alternative to cesium clocks, particularly due to their smaller size and relatively lower manufacturing costs. Utilizing the transition of rubidium-87 atoms, these clocks operate effectively within a broad range of environments, making them a popular choice for mobile devices and portable applications.
Although rubidium clocks generally provide slightly less precision compared to cesium models, they boast a quicker warm-up time and greater frequency stability, leading to their adoption in various satellite and naval projects. This versatility illustrates their benefits but also highlights their limitations; they aren't as accurate as cesium clocks for long-term timekeeping, which means the choice between the two often hinges on the specific requirements of the application.
Optical Lattice Clocks
Mechanisms of Optical Lattice Clocks
Optical lattice clocks represent a groundbreaking advancement in atomic clock technology, utilizing the properties of lasers to trap atoms in a grid-like formation. This mechanism allows the clock to operate at higher frequencies than traditional atomic clocks. By doing so, optical lattice clocks can outperform cesium and rubidium clocks, achieving remarkable accuracy levels that are becoming essential in cutting-edge scientific research.
KEY CHARACTERISTIC: The usage of lasers to confine atoms elevates their ability to minimize environmental disturbances, significantly enhancing their measurement precision. This incomparable accuracy makes optical lattice clocks indispensable in an era where scientific endeavors increasingly depend on precision timekeeping. However, their complexity and high cost can be barriers to widespread adoption, presenting a double-edged sword in clock technology.
Comparison with Traditional Clocks
When juxtaposed with traditional atomic clocks, optical lattice clocks shine due to their unique potential for unparalleled accuracy. Traditional clocks utilize microwave frequencies, while optical lattice clocks harness optical frequencies that are orders of magnitude higher, resulting in better stability and precision.
This monumental difference contributes to a faster sampling rate and a more stable output, which translates into greater timekeeping reliability. However, their cutting-edge technology may not align with the practical needs of every industry. While they pave the way for the future of precise timekeeping, the resources required for implementation can be significant, posing a challenge for users in sectors needing cost-efficiency without sacrificing performance.
Measuring Accuracy in Atomic Clocks
Measuring the accuracy in atomic clocks is not just a topic for physicists alone; it impacts numerous aspects of modern life. From communication methods to navigation systems, the precision of atomic clocks underpins the functionality of a multitude of technologies we often take for granted.
In this discussion, we will navigate the factors that contribute to this accuracy. Understanding them helps highlight the benefits these clocks bring, while also considering the challenges they face. It's essential to grasp how both the environment and technology shape atomic clock performance. As we delve deeper, we will uncover some significant aspects related to measuring accuracy that define the clock's reliability.
Factors Affecting Accuracy
Environmental Influences
Environmental influences play a vital role in determining the accuracy of atomic clocks. This characteristic is crucial because even the smallest external factor can lead to discrepancies, throwing off precise timekeeping. Atmospheric pressure, temperature variations, and electromagnetic radiation can all lead to alterations in the clock’s performance. For instance, if an atomic clock is calibrated in a lab with controlled conditions and then taken outdoors, fluctuations in temperature and humidity could affect its operation.
The unique feature of these influences is their variability – they can change depending on the time of day, location, or even seasonal shifts. Such characteristics make environmental influences both a challenge and an area for improvement in atomic clock technologies. On the upside, these external factors present an opportunity for researchers to develop more resilient designs that adapt to the surrounding environment, enhancing accuracy across diverse settings.
Technological Variances
Technological variances also hold immense significance when it comes to measuring atomic clock accuracy. Modern atomic clocks rely heavily on advancements in technology, which can introduce discrepancies based on the devices used and their configurations. For example, different laser systems used to probe atomic transitions can yield varied results on the clock’s precision. This variance extends to the materials and methodologies employed in construction, calibration, and maintenance of the clocks.
What sets technological variances apart is their continuous evolution. As scientific understanding deepens, new methodologies emerge that can potentially improve the precision of timekeeping. But with these advancements come challenges – it takes time and resources to shift from older systems to new ones. Embracing these technological changes can either enhance accuracy or introduce new potential pitfalls that must be carefully managed.
Standards of Measurement
The standards of measurement surround the accuracy of atomic clocks like an essential framework supporting the entire structure of precise timekeeping. Establishing consistent benchmarks allows scientists and professionals to evaluate and compare performance reliably. The accuracy of atomic clocks is typically measured in terms of second, which is the fundamental unit of time. However, it is the intricacies beyond this simple unit that reveal the true performance metrics.
Organizations such as the International System of Units (SI) and National Institute of Standards and Technology (NIST) play vital roles in setting these benchmarks. Their standards help ensure that the clocks used worldwide adhere to agreed-upon accuracy levels, which is essential as we become more dependent on synchronization in global technology applications.
Overall, measuring accuracy in atomic clocks encapsulates a complex interplay of various domains—environment, technology, and standardized protocols. By keeping a close eye on how these elements interact, we can ensure that these remarkable timekeeping devices continue to meet the ever-evolving demands placed on them.
Applications of Atomic Clock Technology
Atomic clocks, being the heralds of precision timekeeping, find their significance woven into a variety of applications that extend beyond mere time measurement. In a world that races ahead, the accuracy they provide shimmers like a beacon through the fog of uncertainty. Their applications — encompassing everything from GPS to scientific realms — demonstrate their necessity in ensuring our modern conveniences operate without a hitch. Let's delve into some of the most compelling areas where atomic clock technology plays a pivotal role.
Global Positioning System (GPS)
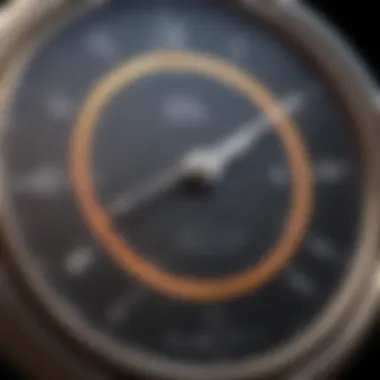
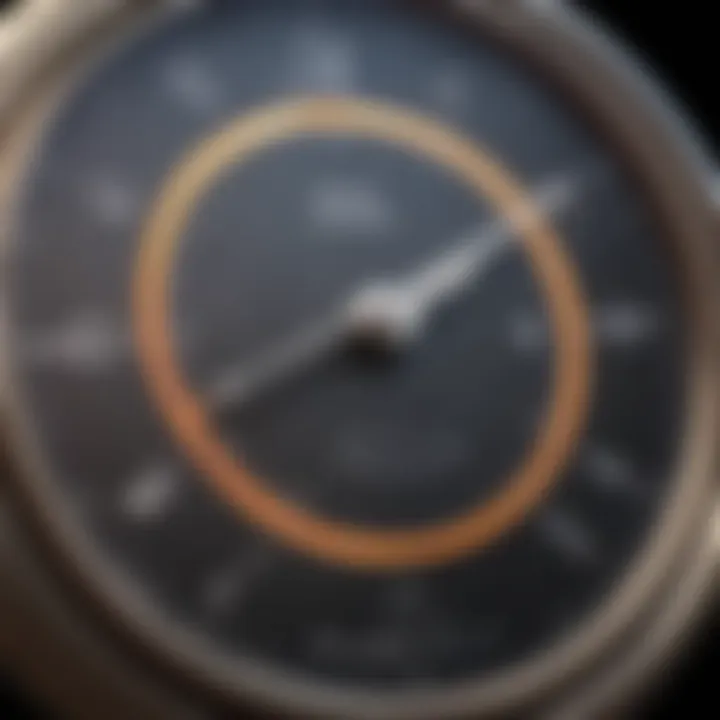
The Global Positioning System (GPS) relies heavily on atomic clocks, making them the backbone of this technology. Without atomic clocks, the pinpoint accuracy we rely on for navigation would be sorely lacking. As satellites orbit the Earth, they transmit signals. These signals include the time they were sent, determined by highly accurate atomic clocks onboard. When your device picks up these signals, it calculates your location by measuring the time delay in the signal's journey. The precision of atomic clocks ensures that this timing is incredibly accurate, leading to improved reliability in positioning.
Telecommunications
Synchronization of Networks
Telecommunications networks demand a level of synchronization that can’t falter. Atomic clocks serve as the gold standard for network time distribution. In essence, all of the different components of a telecommunications infrastructure need to operate in perfect tandem, and atomic clocks ensure this synchronization. By providing temporal alignment, they help in managing bandwidth efficiently and reducing latency during data transmission. The ability of atomic clocks to maintain stability over time makes them an indispensable asset for service providers.
Impact on Data Transmission
The impact of atomic clocks on data transmission is nothing short of revolutionary. They allow for precise timing, which is critical for asynchronous data transfer protocols. By ensuring that data packets are sent and received at designated intervals, atomic clocks minimize the chances of collision, thus optimizing throughput within networks. This characteristic turns atomic clocks into a favorable choice for any provider aiming for excellence in service delivery. The downside, however, can be the costs associated with high-precision timekeeping, particularly for non-tech-centric industries where budget constraints play a significant role.
Scientific Research and Experiments
Tests of Fundamental Physics
In terms of fundamental physics, atomic clocks are vital tools for experimentation and validation of theories. They allow scientists to explore the fabric of time and its relationship with various physical laws. For example, the most precise atomic clocks have been crucial in experiments testing the limits of relativity. They give researchers precise temporality to study interactions at the quantum level. By allowing researchers to measure phenomena that were once considered too nuanced, atomic clocks' utility becomes evident. Their incredible precision opens doors to previously unthinkable research possibilities.
Astrophysical Measurements
When it comes to astrophysics, accurate measurements are a must. Atomic clocks are instrumental in studying celestial bodies and phenomena. They enable astronomers to measure cosmic distances and analyze the motion of planets and stars with exceptional detail. The unique feature of using atomic clocks in astrophysics lies in their ability to reinforce theories related to the universe while measuring indicators like redshift. This advantage, in a sense, tightens the grip on fundamental truths about the cosmos.
"The universe is a symphony of time; atomic clocks may just be the conductors."
In summary, the applications of atomic clock technology emphasize its importance and relevance across various sectors. From GPS navigation that guides millions daily to the realms of telecommunications and scientific explorations, atomic clocks form an integral part of our technological ecosystem.
Recent Advancements in Atomic Clock Technology
The rapid pace of advancements in atomic clock technology is akin to the synchronous ticking of a finely tuned mechanism—each development propelling the field toward greater accuracy and functionality. These developments not only enhance the precision of timekeeping but also open up a range of applications across diverse sectors like telecommunications, global positioning, and fundamental science. By concentrating on the innovations ushering in this new era, we can grasp their significance and potential impact on future technologies.
Development of New Materials
Recent years have seen a significant shift in the materials used in atomic clocks. Traditionally, cesium and rubidium have dominated the field, with their inherent properties enabling reliable timekeeping. However, research is revealing that new materials can enhance atomic clock performance. For instance, silicon carbide is emerging as a frontrunner. Its attributes make it suitable for fabricating components that reduce noise and errors in frequency measurements, increasing clock accuracy.
Moreover, these new materials can improve environmental resilience. Clocks that utilize innovative substances can withstand extreme conditions where standard materials would falter. This shift not only extends the operational capabilities of atomic clocks but also their applicability in harsh environments. Such developments hint at a future where atomic clocks could become integral in a broader spectrum of fields, from aerospace to deep-sea exploration.
Improved Measurement Techniques
Innovations in Laser Technology
At the heart of recent advancements lies the enhancement in laser technology. Modern atomic clocks rely heavily on lasers to probe atomic transitions with remarkable precision. Newly developed laser systems, particularly those employing frequency combs, represent a noteworthy evolution in this area. These devices generate numerous closely spaced frequencies, essentially creating a 'ruler' for measuring time with unprecedented accuracy.
A key feature of these innovations is their ability to stabilize the frequency of lasers, minimizing fluctuations that could distort time measurement. As a result, atomic clocks can achieve stability levels that only dreamt of a decade ago.
However, these innovations are not without challenges. High costs and the complexity of maintaining these systems can be barriers during implementation. Nevertheless, the potential benefits often outweigh these concerns, making laser technology a popular choice in advancing timekeeping accuracy.
Quantum Computing Impacts
Quantum computing stands as another significant factor influencing atomic clock technology. This next-generation computing approach provides new paradigms for conducting calculations regarding atomic states and energy levels with unparalleled speed and accuracy. The nature of quantum computing allows for simulations of atomic behavior that were previously too complicated for traditional computers, paving the way for improvements in the characterization of atomic transitions.
The most noteworthy characteristic of quantum computing's influence on atomic clocks is its ability to process enormous datasets derived from atomic interactions almost instantaneously. The implications of this are broad, potentially revolutionizing how researchers develop and test new clock designs. However, challenges exist in terms of integrating quantum systems with existing atomic clock technology—these hurdles must be overcome for optimal synergy.
In summary, the pursuit of developing new materials and refining measurement techniques through innovations in laser technology and quantum computing signifies a robust future for atomic clocks. As these advancements unfold, their influence stretches far beyond mere timekeeping. Every tick of these atomic wonders encapsulates a blend of science and technology that holds infinite potential for our ever-evolving world.
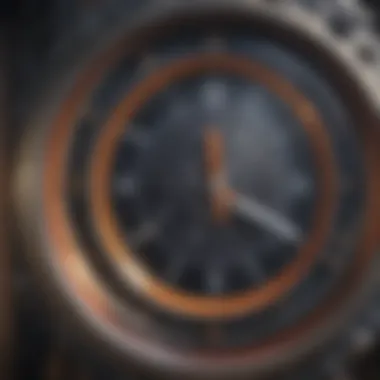
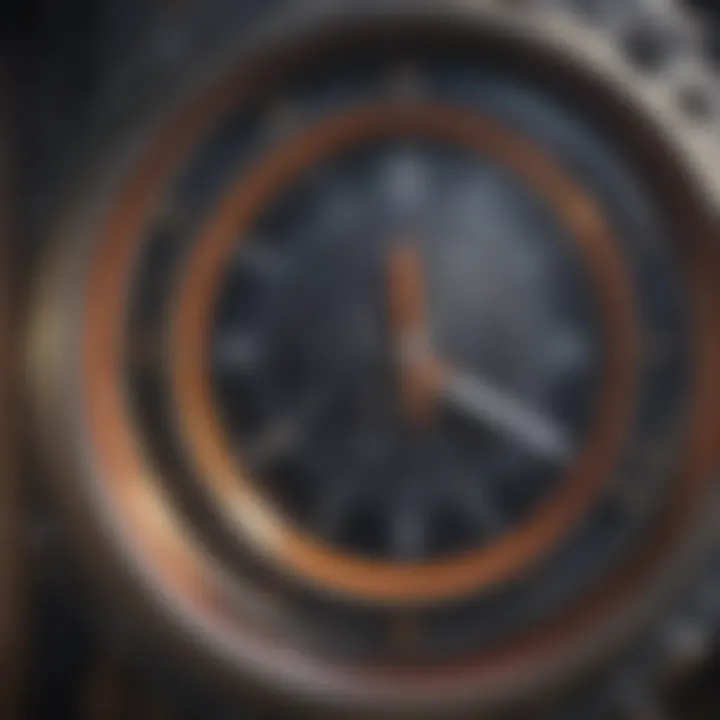
Challenges in Atomic Clock Development
The journey of atomic clock technology is fascinating. However, it is not without its hurdles. Understanding the challenges in atomic clock development is crucial, as they define the limits of our current capabilities and inspire future innovations. The importance of this topic lies in its direct impact on accuracy, reliability, and overall accessibility of atomic clocks in various applications.
Technical Limitations
One significant challenge lies in the technical limitations faced by researchers and engineers. Atomic clocks operate based on specific atomic transitions, and the precision of these transitions can be influenced by several factors. For example, environmental factors such as temperature fluctuations and magnetic fields can lead to frequency instabilities.
There’s a balance that must be struck between achieving unparalleled accuracy and managing these environmental influences. Additionally, the complexity involved in the design and manufacturing of atomic clocks adds another layer of difficulty. High-precision equipment often requires specialized laboratories and instruments, making trial-and-error methods both time-consuming and costly.
Some specific technical limitations include:
- Precision Materials: Not all materials behave ideally at atomic levels. Finding materials that minimize noise and contribute to stability is an ongoing challenge.
- Quantum Decoherence: As the conditions around the clock change, the quantum state of atoms may interact with their environment, affecting the clock’s performance.
- Complexity of Calibration: Regular maintenance and recalibration are vital; however, these processes can introduce errors if not done meticulously.
"The pursuit of accuracy in atomic clocks is like trying to catch smoke with your bare hands—every effort reveals more intricacies that challenge our understanding."
Cost and Accessibility
Cost is another considerable barrier in the field of atomic clocks, heavily impacting their accessibility. High-end atomic clocks, particularly optical lattice clocks, involve not just expensive components but also sophisticated operational expertise. The expenses related to such technology can limit its use to governments, large corporations, or dedicated research institutions, thereby sidelining smaller players or emerging markets.
Here are a few aspects regarding costs and accessibility:
- R&D Investments: The initial investments for research and development are substantial, often requiring significant funding from government bodies or large organizations.
- Operational Costs: Maintaining high precision equipment incurs ongoing costs, including power consumption and regular components replacement, affecting operational budgets of institutions.
- Training Requirement: Operating atomic clocks requires specialized knowledge, so training personnel in these advanced technologies adds another layer of cost.
Ultimately, these challenges can hinder the widespread adoption and benefits of atomic clock technology. Bridging these gaps might involve innovative approaches to engineering and a reevaluation of what is deemed essential for accuracy in timekeeping.
Future Perspectives on Atomic Clock Accuracy
The exploration of atomic clock accuracy offers a glimpse into the future of timekeeping. In a world that increasingly relies on precision, understanding where atomic clock technology is headed is crucial. Advancements in this field can significantly affect various industries, from telecommunications to scientific research. The reliability of time measurement is paramount, and predicting how these developments will unfold is not just speculative but has tangible implications.
Predicted Trends in Technology
Looking forward, several trends are expected to shape atomic clock technology:
- Miniaturization of Technology: As with many tech advances, the trend towards smaller, more efficient devices is likely to continue. Expect atomic clocks to shrink in size without sacrificing accuracy, making them more accessible for both consumer devices and broader applications.
- Integration with Quantum Technologies: With quantum computing gaining traction, its integration with atomic clocks might lead to revolutionary improvements. Quantum-enhanced atomic clocks could provide unprecedented levels of precision, challenging our current understanding of time.
- Flexibility in Frequency Standards: The future might bring flexible frequency standards that cater to specific needs across industries. This flexibility could lead to adjustments in design that optimize performance based on environmental conditions or operational demands.
- Networking and Connectivity: Enhanced connectivity features could emerge, where atomic clocks sync seamlessly with other devices or networks. This means that accuracy does not just come from standalone operations but also from collaborative systems that ensure synchronized timekeeping globally.
Potential Impacts on Global Standards
The implications of advancements in atomic clock accuracy are extensive:
"The elegance of atomic clock precision might redefine what we consider standard time, impacting everything from GPS accuracy to fundamental measurements in physics."
- GPS and Navigation Systems: Enhanced atomic clock precision will boost GPS technology stability. As navigation systems become increasingly reliant on accurate timing, global positioning accuracy can dramatically improve, optimizing travel routes and logistics.
- Scientific Research: The scientific community stands to gain significantly from more accurate atomic clocks. Areas such as astronomy, where time measurements of phenomena across vast distances are vital, could see a paradigm shift in data collection and analysis methodologies.
- Standards and Regulations: New, higher standards may emerge from the advancements in atomic clock technologies. Governments and organizations may need to adapt regulations regarding timekeeping in various industries, aligning with these new benchmarks to ensure consistency and reliability.
- Economical and Social Impact: The ripple effects of improved accuracy will extend into economic practices and daily life. Industries like finance that depend heavily on time-stamped transactions may streamline operations, leading to indirect benefits in productivity and accountability.
In summary, future perspectives on atomic clock accuracy promise fascinating developments. Understanding these trends not only prepares various sectors for change but also highlights the necessity of continual research and adaptation in the ever-evolving landscape of timekeeping.
Closure
The exploration of atomic clocks delivers profound insights not only into their operational principles but also into their broader significance in the ever-evolving field of timekeeping. The accuracy inherent in atomic clocks—often on the scale of billionths of a second—reshapes how we view precision in time measurement. This accuracy plays a crucial role in navigation, telecommunications, and scientific research, making it imperative for industries that depend on synchronization.
Summation of Key Findings
In reviewing the landscape of atomic clock technology, several pivotal points emerge:
- Precision Powerhouse: Atomic clocks stand at the forefront of timekeeping accuracy, vastly outperforming traditional clocks. This capacity for precision not only helps maintain the integrity of GPS systems but also aids in scientific discoveries where timing can be critical.
- Diversity of Technologies: From Cesium to optical lattice clocks, each type offers unique advantages tailored to varied applications. For instance, while Cesium clocks serve well for global positioning, optical lattice clocks promise even greater precision for future research endeavors.
- The Evolution Ahead: Recent advancements in materials and measurement techniques highlight a promising trajectory, indicating that future atomic clocks will likely push the boundaries of timekeeping accuracy further than we’ve ever known.
The Importance of Ongoing Research
As technology advances, the quest for greater accuracy in atomic clocks remains vital. Ongoing research addresses challenges such as improving resilience to environmental factors and minimizing costs to make the technology more accessible. This continuous exploration ensures that atomic clocks not only maintain relevancy but also evolve to meet the demands of burgeoning fields such as quantum computing and relativity studies.