Understanding the Biological Immune System: Mechanisms and Implications
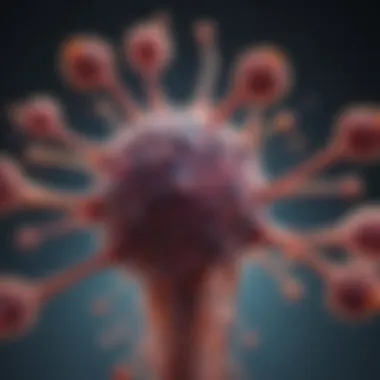
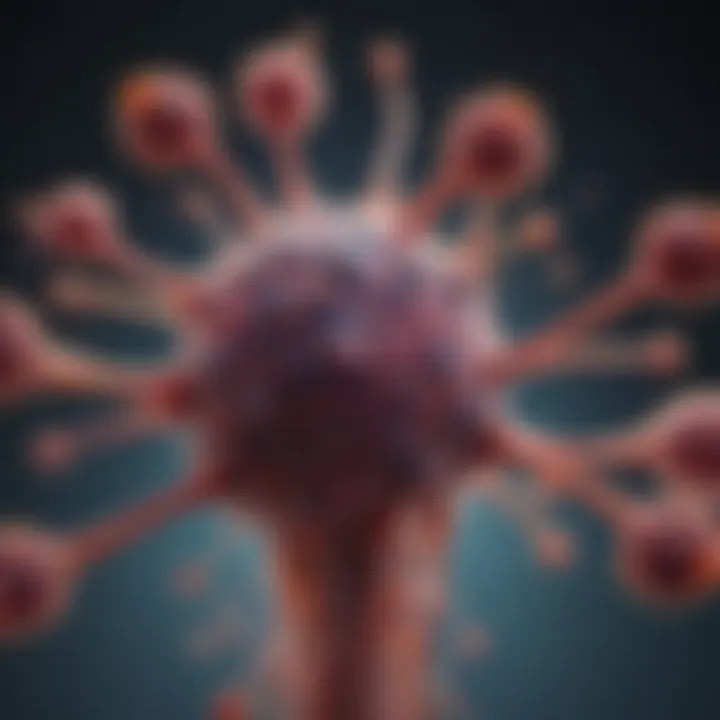
Intro
The biological immune system serves as a critical guardian of human health, operating through intricate mechanisms. With its complex interactions and functions, the immune system ensures that the body remains resilient against various pathogens. Understanding these processes is essential for both researchers and healthcare professionals alike.
This exploration begins by examining the two primary components of immunity: innate and adaptive immunity. Each plays a distinctive role in defending the body from infections and diseases. The immune system's ability to adapt becomes particularly relevant when discussing its implications for health, vaccines, and emerging therapies.
From the fundamentals of immune cells to the latest advances in immunology, this article intends to provide a thorough analysis. Consideration will be given to how immune responses relate to disease processes. Additionally, the narrative will explore the influence of environmental factors and genetics.
By delving into this subject, we aim to offer a cohesive understanding of how the immune system operates. The insights gathered might be valuable in the ongoing efforts to combat infectious diseases, improve vaccination strategies, and develop personalized treatments for various health concerns.
Prologue to the Biological Immune System
The biological immune system plays a critical role in maintaining human health by providing a defense against pathogens such as bacteria, viruses, and fungi. Understanding the mechanisms and functions of this system is essential for students, researchers, educators, and professionals who seek to comprehend the complexities of human biology. The immune system is not just a collection of cells; it is a highly sophisticated and adaptive network that continuously learns from and responds to various threats.
In this section, we will define what the immune system is and discuss its importance. By recognizing its functions and components, we lay the groundwork for further exploration of the immune system's intricacies.
Definition and Importance
The immune system can be simply defined as the body's defense mechanism, which identifies and eliminates harmful entities. This includes a variety of cells, tissues, and organs that work in concert to protect the body from disease. Importantly, the immune system exhibits two main types of immunity: innate and adaptive. Innate immunity is the first line of defense and provides immediate reactions, whereas adaptive immunity develops over time and targets specific pathogens more effectively.
The importance of the immune system cannot be overstated. A well-functioning immune system can distinguish between self and non-self, allowing it to target and eliminate invaders while preserving the body's own cells. Furthermore, the immune response has implications beyond just pathogen defense. It also plays a role in wound healing, cancer surveillance, and the regulation of inflammation.
"The immune system's ability to remember past infections enables it to respond more robustly upon re-exposure, effectively contributing to long-term health."
Moreover, disruptions to the immune system can lead to a range of health issues, including autoimmune diseases, immunodeficiency disorders, and increased susceptibility to infections. Understanding these mechanisms is crucial for developing effective treatments and preventive measures.
Components of the Immune System
The components of the immune system play a crucial role in its overall functionality. Understanding these elements provides insights into how the immune system protects the body from pathogens and maintains health. Key components include specialized immune cells and various organs and tissues that work in coordination. Each component has unique roles and characteristics that contribute to the effective functioning of immune responses.
Immune Cells
Immune cells are the frontline defenders of the body. They are crucial for recognizing and eliminating pathogens, as well as for regulating immune responses. Different types of immune cells work together to create a robust defense mechanism.
B Cells
B Cells are essential players in the immune response. Their primary role is to produce antibodies, which are proteins that neutralize pathogens. A key characteristic of B Cells is their ability to undergo somatic hypermutation, enhancing their affinity for specific antigens. This makes them particularly effective in long-term immunity.
Unique to B Cells is their memory function; once activated, they can remain in the body for years, providing rapid responses upon re-exposure to pathogens. Although they are highly effective against circulating pathogens, their dependency on T Cells for activation can be seen as a limitation.
T Cells
T Cells are another vital component of the immune system. They come in various subtypes, including helper T Cells and cytotoxic T Cells. The key characteristic of T Cells is their ability to recognize infected cells through their specific T cell receptors (TCR). This recognition is essential for targeting intracellular pathogens such as viruses.
What distinguishes T Cells is their role in orchestrating the overall immune response. Cytotoxic T Cells can directly kill infected cells, while helper T Cells enhance the activity of other immune cells. However, they require antigen presentation by dendritic cells or macrophages for activation, which can be a limiting factor under certain conditions.
Macrophages
Macrophages serve as versatile immune cells. They are responsible for phagocytosis, where they engulf and destroy pathogens. A key characteristic of macrophages is their ability to present antigens to T Cells, bridging innate and adaptive immunity. This makes them crucial for initiating the immune response when a pathogen is detected.
One unique feature of macrophages is their plasticity. They can adopt different functional states based on the cytokine environment, allowing them to respond dynamically to infection and inflammation. Nonetheless, their efficacy can be compromised in chronic inflammatory conditions.
Dendritic Cells
Dendritic Cells are critical for the activation of T Cells. They act as professional antigen-presenting cells, capturing and processing antigens from pathogens. The key characteristic of dendritic cells is their ability to migrate to lymph nodes, where they present antigens to T Cells. This is pivotal for initiating adaptive immune responses.
What sets dendritic cells apart is their role in determining the type of immune response generated—whether it will be humoral or cell-mediated. However, their relatively low abundance compared to other immune cells can limit their ability to activate a rapid response.
Organs and Tissues
The immune system is not solely dependent on immune cells; specific organs and tissues are also vital for its functionality. These components provide the necessary environment for immune cell maturation, activation, and coordination. This section examines key organs and their roles in maintaining immunity.
Thymus
The Thymus is essential for T Cell development. It is where immature T Cells undergo maturation and selection. A key characteristic of the thymus is the process of positive and negative selection, which ensures that mature T Cells can recognize foreign antigens while remaining tolerant to self-antigens.
The unique feature of the thymus is its role in generating a diverse T Cell repertoire. As individuals age, the thymus shrinks, potentially limiting the body’s ability to produce new T Cells.
Bone Marrow
Bone Marrow is the primary site for hematopoiesis, where all blood cell types, including immune cells, originate. It is essential for the production of B Cells and the precursors of T Cells. A key characteristic of bone marrow is its supportive microenvironment, which nurtures developing immune cells.
One unique aspect of bone marrow is its ability to continue producing immune cells throughout life. However, certain conditions, like leukemia, can disrupt its function, leading to immune deficiencies.
Spleen
The Spleen functions as a blood filter and is crucial in mounting an immune response to blood-borne pathogens. A key characteristic of the spleen is its ability to store immune cells, like B and T Cells, while also filtering out old or damaged blood cells.
A unique feature of the spleen is its two main components: the red pulp and white pulp, each serving distinct functions. However, removal or dysfunction of the spleen may increase susceptibility to infections.
Lymph Nodes
Lymph Nodes act as filters in the lymphatic system, playing a critical role in immune surveillance. Their primary purpose is to facilitate interactions between different immune cells. A key characteristic of lymph nodes is their structure, which provides distinct areas for the activation of B and T cells.
What makes lymph nodes unique is their ability to trap antigens and present them to lymphocytes. This function can also be a disadvantage, as swollen lymph nodes can indicate infection or immune response.
Types of Immunity
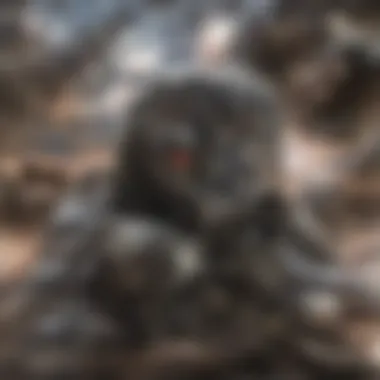
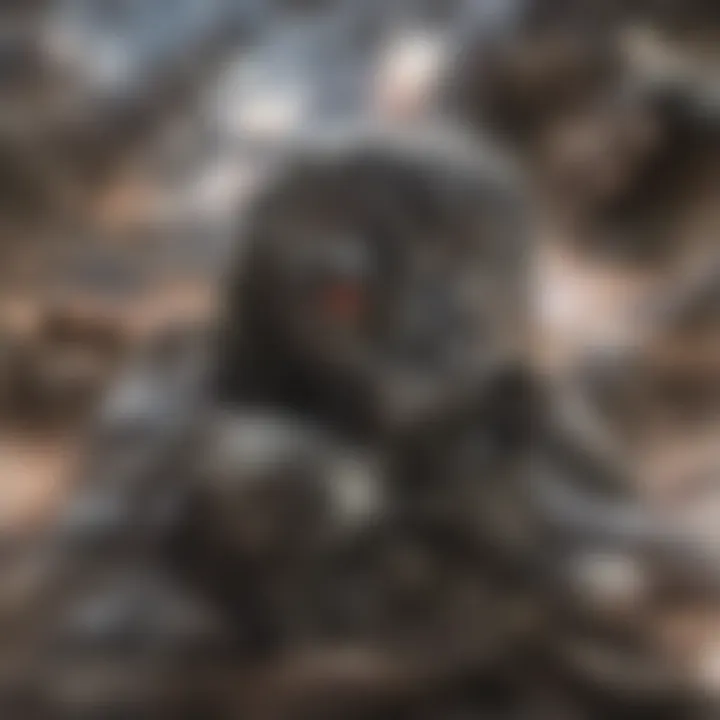
Understanding the two main types of immunity is critical for grasping how the biological immune system functions. Immunity can broadly be categorized into innate and adaptive immunity. This distinction is not only significant but also useful in explaining different immune mechanisms and responses. Recognizing these types allows researchers and health practitioners to appreciate how the body defends itself against pathogens through various strategies.
Innate Immunity
The innate immune system serves as the first line of defense against pathogens. It is characterized by its immediate response to invading organisms and does not require prior exposure.
Physical Barriers
Physical barriers are among the most fundamental aspects of innate immunity. Skin and mucous membranes act as shields against pathogens. This attribute—being the body's primary defense—makes it very effective. The unique feature is that these barriers are always present and functioning, creating a continuous protective shield. This constant readiness allows the body to thwart many infections before they take hold. However, if these barriers are breached, the body must rely on other immunity components, which might not be as immediate.
Cellular Responses
Cellular responses encompass various immune cells that react quickly to infections. Phagocytes, such as macrophages, engulf pathogens. This immediate action is vital for preventing infection spread. The key characteristic of cellular responses is its ability to respond swiftly. These innate immune cells act first and can adapt slightly based on previous encounters. While beneficial, it is not as specific as adaptive responses.
Chemical Mediators
Chemical mediators play a role in signaling and regulating the immune response. Cytokines, for instance, are secreted by immune cells to communicate and attract other cells to infection sites. This feature allows for coordinated attacking strategies, enhancing the innate immune response. However, the challenge is that excessive signaling can lead to chronic inflammation and tissue damage.
Adaptive Immunity
Adaptive immunity develops after exposure to specific pathogens. This type of immunity is characterized by specificity and memory, enabling the immune system to recognize and respond more effectively upon subsequent encounters.
Humoral Response
Humoral response involves B cells that produce antibodies against specific antigens. These antibodies neutralize or eliminate antigens effectively. The main attribute of this response type is its high specificity for pathogens. This feature is beneficial since it enhances targeted protection and can prevent future infections. Yet, it takes time to develop following initial exposure.
Cell-Mediated Response
The cell-mediated response relies on T cells to fight infected or cancerous cells. T cells can directly kill infected cells or help boost other immune responses. The key characteristic here is versatility, as these T cells adapt based on the type of invasion. This adaptability makes them invaluable in immune functionality. Nonetheless, like the humoral response, it demands time for effective action.
Memory Cells
Memory cells are crucial for long-term immunity. After initial exposure to a pathogen, some B and T cells become memory cells that can respond rapidly to future infections by the same pathogen. Their defining characteristic is their longevity and speed of response. They ensure that the body retains the capability to defend against previously encountered threats. However, over time, some memory cells can diminish in number, potentially leaving a gap in the immune response.
"The adaptive immune system's memory provides an efficient means of protection, enabling quicker responses upon re-exposure to pathogens."
With a thorough understanding of the types of immunity, it is easier to appreciate the complexity and interrelation of these immune responses. Each plays a vital role in maintaining health, providing layers of defense against myriad threats.
Immune System Functions
The functionality of the immune system serves as the core of human resilience against diseases. Understanding immune system functions is crucial because it informs how our body reacts to foreign invaders, enhancing both preventive and therapeutic strategies. The immune system is not merely a passive shield; it is an intricate, responsive mechanism that actively identifies, combats, and ultimately helps eliminate pathogens. The effective function of the immune system contributes to overall health, reduces susceptibility to infections, and supports recovery from injuries and illnesses. Evaluating these functions allows for a greater insight into disease mechanisms, treatment options, and preventive care strategies.
Pathogen Recognition
Pathogen recognition is the foundational step in the immune response. This process involves the detection of foreign substances known as antigens. Immune cells, particularly dendritic cells and macrophages, play a significant role in recognizing these antigens. These cells express pattern recognition receptors (PRRs) that identify common features of pathogens, helping to discriminate between self and non-self. Once a pathogen is identified, these immune cells process and present the antigen to T cells, thus alerting the immune system to initiate a response.
The ability to recognize a wide variety of pathogens is critical. For instance, viruses, bacteria, and parasites exhibit diverse molecular structures, necessitating a highly sensitive recognition system. This adaptability prevents pathogens from overwhelming the immune defenses. Furthermore, correct pathogen recognition sets off a cascade of immune activation. If the sensors of the immune system fail or misidentify antigens, it can lead to inadequate immune responses or autoimmune diseases, where the body attacks its own cells.
Activation of Immune Responses
Activation of immune responses follows the recognition of pathogens. Upon successful identification, B and T cells become activated. B cells are responsible for producing antibodies, which bind to specific antigens, neutralizing pathogens or marking them for destruction. T cells take on roles in cell-mediated immunity, directly killing infected cells or orchestrating the immune response by releasing signaling molecules called cytokines.
This dual activation leads to clonal expansion, where specific T and B cells multiply to effectively target the identified pathogen. Importantly, activated immune cells undergo changes that enhance their functionality, increasing the efficacy of the immune response. This activation phase is crucial for eliminating pathogens but also has implications for immunological memory. The immune system's efficiency in activating the appropriate cellular responses can dictate the outcome of infections and influence vaccine development strategies.
Regulation and Resolution of Inflammation
The immune system not only fights infections but also must regulate and resolve inflammation efficiently. Inflammation is a natural response to infection or injury, characterized by redness, heat, swelling, and pain. While necessary for healing, prolonged or excessive inflammation can lead to tissue damage and chronic diseases. Therefore, the control of inflammation is essential in preventing collateral damage to host tissues.
Key immune cells, such as regulatory T cells, play an important role in balancing the immune response. They help suppress overactive immune functions and promote resolution. Additionally, various cytokines serve to manage this inflammatory response. For example, interleukin-10 is known for its anti-inflammatory properties.
The processes of regulation and resolution ensure that the immune system returns to a resting state after the threat has been eliminated. Failure in this aspect can lead to conditions like allergies or autoimmune disorders. Maintaining this homeostasis is vital for long-term health and recovery.
"An effective immune system is marked by its ability to recognize threats, mount the appropriate responses, and return to equilibrium promptly."
Through understanding these functions, one gains insight into the complexities of the immune system. This comprehension can pilot advancements in immunotherapies, vaccines, and approaches to tackle autoimmune diseases. The fine-tuning of immune functions heralds significant potential in the fight against diverse health challenges.
The Immune Response Process
The immune response process is a central theme within the study of the biological immune system. It underscores how the body detects, responds to, and eradicates potential threats such as pathogens. Understanding this process is critical for grasping the functionality of the immune system and its significance in maintaining health. The immune response can be organized into a series of phases that ensure proper identification of harmful agents and appropriate subsequent actions.
Initial Immune Activation
Initial immune activation occurs when antigen-presenting cells, such as dendritic cells, encounter pathogens and ingest them. These cells then process the antigens and present them on their surface using molecules called Major Histocompatibility Complex (MHC). When naive T cells encounter their specific antigen presented in this form, they become activated. This stage involves the signaling from co-stimulatory molecules, which also plays a crucial role in T cell activation.
The importance of initial activation cannot be overstated, as it sets the stage for a cascade of immune responses. Appropriate activation leads to further proliferation and differentiation of T cells and B cells, which is essential for both immediate defense and long-term immunity.
Clonal Expansion
Once activated, T cells undergo clonal expansion. This means they rapidly divide and produce clones of themselves, each capable of recognizing the same specific antigen. The recruitment of antigen-specific T cells is vital for an effective immune response. Some of these cells will differentiate into effector T cells that target infected cells, while others will become memory T cells that persist after an infection has been cleared.
This phase ensures a robust response. The efficiency of clonal expansion determines how quickly the immune system can mount an effective defense. As more antigen-specific cells are generated, the immune system can respond more vigorously to the threat.
Effector Function
Effector function is the next key phase in the immune response. During this stage, the activated T cells and B cells exert their effects against the pathogens. CD8+ T cells, also known as cytotoxic T lymphocytes, directly kill infected cells by recognizing antigen on their surface. On the other hand, B cells produce antibodies that neutralize pathogens or mark them for destruction by other immune cells.
The effectiveness of this response is crucial for eliminating the infection. The coordinated efforts of various immune cells directly impact the pathophysiology of the disease and determine clinical outcomes. A well-timed and efficient effector function can lead to the resolution of the infection and restore homeostasis in the body.
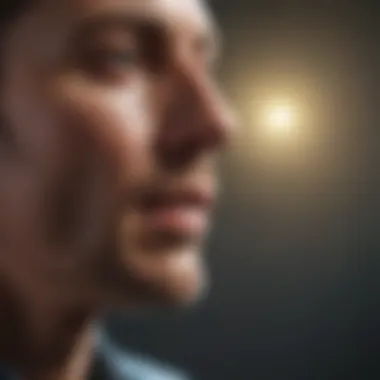
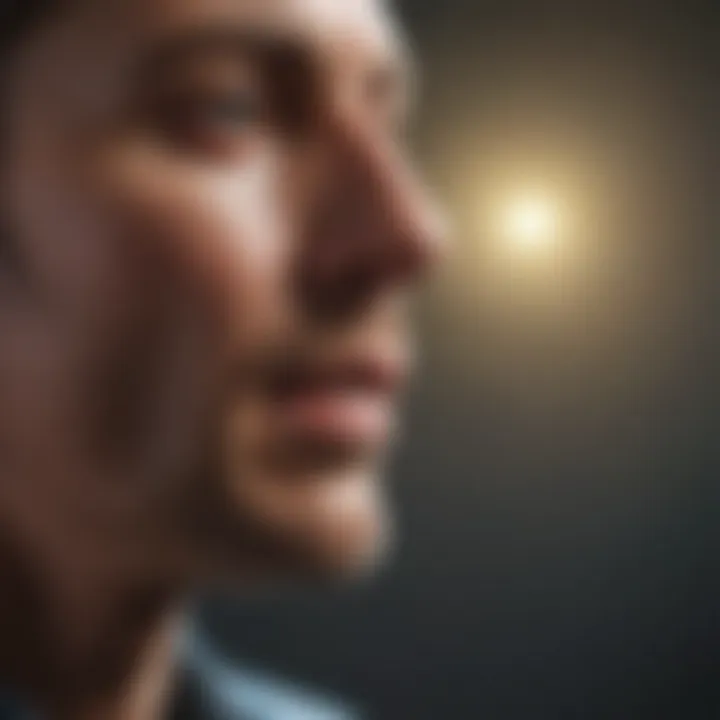
Memory Formation
Following the clearance of a pathogen, the immune system develops memory cells, a process known as memory formation. These memory T and B cells persist long after the initial immune response has resolved. They remain vigilant, ready to respond more rapidly and effectively upon re-exposure to the same pathogen in the future.
Memory formation is vital for long-term immunity. It decreases the likelihood of reinfection and modifies the immune response during subsequent encounters with the same pathogen. This process is also fundamental to the principle behind vaccinations, as it allows the immune system to remember and tackle pathogens without causing disease.
"The ability to generate a memory response is what makes the immune system so effective against repeated infections."
In summary, the immune response process involves precise coordination and execution of several key phases. Each phase, from initial activation to memory formation, plays a specific role in ensuring that the body can effectively defend itself against pathogens. Understanding these processes is essential for advancements in immunology, vaccine development, and therapeutic interventions.
The Role of Antigens
Antigens are essential players in the function of the immune system, directing immune responses against foreign invaders. These molecules are typically found on the surface of pathogens like bacteria and viruses. Understanding the significance of antigens is crucial for anyone studying immunology, as they are the key to how immune cells recognize threats. This section will delve into the definition of antigens and explore how immune cells are trained to recognize them, forming a foundation for broader discussions on immune mechanisms.
Definition of Antigens
Antigens are substances that induce an immune response in the body. They can be proteins, polysaccharides, lipids, or nucleic acids. When the body detects antigens, it triggers the production of antibodies or stimulates specific immune cells to react.
Some key features of antigens include:
- Specificity: Each antigen is unique and can stimulate a specific immune response.
- Immunogenicity: This property determines how well an antigen can provoke an immune response. Some antigens are highly immunogenic, while others might not elicit a strong response.
Antigens can be categorized into two main types:
- Exogenous Antigens: These come from outside the body, like pathogens. They are recognized by the immune system and can provoke responses.
- Endogenous Antigens: These arise from within the body, often from infected or cancerous cells. The immune system still needs to identify and address these threats.
Antigen Recognition by Immune Cells
The recognition of antigens is a complex process involving specialized immune cells. Both B cells and T cells play critical roles in this process.
- B Cells: These lymphocytes can recognize free-floating antigens in the body. Upon detection, B cells can produce antibodies that specifically target the identified antigens. This interaction is vital for neutralizing pathogens.
- T Cells: Unlike B cells, T cells require antigens to be presented by other cells. This presentation often occurs through major histocompatibility complex (MHC) molecules on the surface of antigen-presenting cells. T cells can then trigger various immune responses.
The process of antigen recognition is essential for:
- Mounting effective immune responses: The ability of immune cells to identify antigens directly correlates with the effectiveness of the immune response.
- Memory formation: Upon encountering an antigen, some immune cells become memory cells, allowing for a quicker and stronger response during future exposures
This mechanism showcases the adaptability of the immune system and highlights why a precise understanding of antigens is vital in fields such as vaccine development and immunotherapy.
Immune System and Disease
The intricate relationship between the immune system and disease is vital for understanding human health and disease prevention. The immune system works tirelessly to protect the body from pathogens, such as bacteria and viruses. However, when it malfunctions, it can contribute to various diseases. Understanding these interactions is crucial for developing effective therapies and preventive measures.
Autoimmune Diseases
Autoimmune diseases reflect a failure of the immune system to distinguish between self and non-self. In these conditions, the immune system attacks the body’s own tissues, leading to inflammation and damage. Common examples include rheumatoid arthritis, lupus, and multiple sclerosis. The emergence of these diseases can be linked to several factors, such as genetic predisposition and environmental triggers.
The consequences of autoimmune diseases are significant. They lead to chronic pain, fatigue, and reduced quality of life. Understanding the underlying mechanisms can inform treatment strategies. Moreover, ongoing research aims to uncover new methods to modulate the immune response, offering hope for affected individuals.
Immunodeficiency Disorders
Immunodeficiency disorders occur when the immune system’s ability to respond is compromised. This can be congenital, as seen in conditions like severe combined immunodeficiency (SCID), or acquired, as seen in HIV/AIDS. Patients with immunodeficiency are at higher risk for infections, some of which can be life-threatening.
The importance of addressing these disorders cannot be understated. Effective treatments, including antiretroviral therapy for HIV, have transformed the prognosis for many patients. Additionally, understanding immunodeficiency can lead to better preventive strategies and educational efforts about infection control.
Cancer and the Immune System
Cancer represents a unique challenge for the immune system. Tumors can evade immune detection through various mechanisms, leading to disease progression. However, the immune system can also be harnessed against cancer. Immunotherapy has emerged as a promising treatment approach, utilizing the body's own immune cells to target and destroy cancer cells.
The relationship between cancer and the immune system is complex. Research is ongoing to determine how best to enhance immune responses to fight cancer. Studying this interplay offers insights that may pave the way for novel therapies and improved patient outcomes.
The immune system's role in disease highlights the delicate balance between protection and pathology. Understanding these mechanisms provides a pathway to innovative treatments and preventive measures in immunology.
Vaccinations and Immunity
Vaccinations play a crucial role in public health by preparing the immune system to combat various infectious diseases. The significance of vaccines lies in their ability to stimulate an immune response without causing the disease itself. This has broad implications for individual health and community wellness. Immunity gained through vaccination reduces the incidence of illness, leading to lower healthcare costs and improved quality of life.
As society faces emerging infectious diseases, the importance of effective vaccination programs cannot be overstated. Vaccines also contribute towards herd immunity, which protects those who cannot be vaccinated, including certain vulnerable populations. This collective defense strengthens community health and guards against outbreaks.
Mechanism of Vaccines
Vaccines operate by mimicking an infection, prompting the immune system to respond. Once administered, a vaccine introduces antigens, either as weakened or inactivated versions of pathogens. The immune system recognizes these antigens as foreign, activating immune cells to fight the perceived threat.
This activation initiates the production of antibodies specific to the introduced antigens. Notably, memory T and B cells form during this process. These memory cells remain in the body, ensuring the immune system can mount a swift response if it encounters the real pathogen in the future. Thus, vaccines provide long-term protection, demonstrating their effectiveness over time.
Types of Vaccines
Vaccines are categorized based on their components and how they induce an immune response. Each type has unique characteristics that determine its usage and effectiveness. Understanding these types aids in better grasping their importance in medical science.
Live Attenuated Vaccines
Live attenuated vaccines contain living pathogens that have been weakened so they cannot cause disease in healthy individuals. A key characteristic of these vaccines is their ability to closely mimic a natural infection, leading to a robust immune response. This contributes to the generation of strong and lasting immunity. For example, the measles, mumps, and rubella (MMR) vaccine is a notable live attenuated vaccine.
The unique feature of live attenuated vaccines is the presence of live microbes, which prompts both cellular and humoral immunity. However, these vaccines may not be suitable for immunocompromised individuals due to the risk of disease from the attenuated pathogen. Generally, they are regarded as beneficial choices for healthy populations.
Inactivated Vaccines
Inactivated vaccines are composed of pathogens that have been killed or inactivated. A key attribute is their safety, as they carry no risk of causing disease, making them a suitable option for those with weaker immune systems. An example includes the polio vaccine, which is an inactivated form.
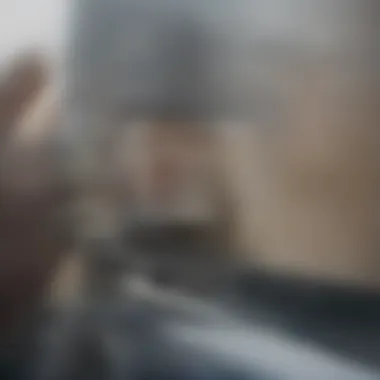
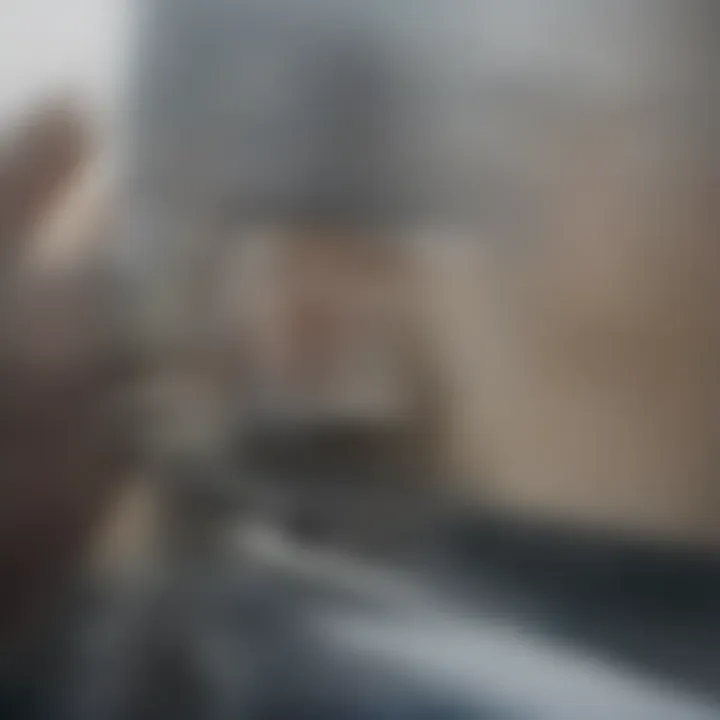
Their advantage lies in the reduced risk of infection since they cannot replicate. However, a limitation of inactivated vaccines is the need for boosters to maintain immunity because the immune response might not be as strong or long-lasting compared to that of live attenuated vaccines.
mRNA Vaccines
mRNA vaccines represent a newer technology that uses messenger RNA to instruct cells to produce a protein that is part of the pathogen. This prompts an immune response without using live virus. A key characteristic is the speed of development and production, exemplified by the Pfizer-BioNTech and Moderna COVID-19 vaccines.
The unique feature of mRNA vaccines is their ability to adapt quickly to mutations of pathogens since changing the mRNA sequence requires minimal time. However, challenges remain, such as storage conditions that require freezing and possible side effects. They are recognized as an important advancement in vaccine technology, providing essential tools for managing infections.
"Vaccination not only protects the individual but also strengthens community health and resilience against outbreaks."
Advances in Immunotherapy
Immunotherapy has emerged as a revolutionizing approach in treating various diseases, particularly cancer. Its significance lies in its ability to use the body's immune system to identify and destroy abnormal cells. Unlike traditional therapies such as chemotherapy and radiation, which can damage healthy tissues, immunotherapy aims for precision by enhancing the body's natural defenses. This targeted approach promises not only better efficacy but also potentially reduced side effects.
With advancements in technology and scientific understanding, immunotherapy has broadened the horizons of patient care. This section will discuss two critical dimensions of immunotherapy: cell-based therapies and monoclonal antibodies.
Cell-Based Therapies
Cell-based therapies represent a novel and dynamic avenue within immunotherapy. They utilize living cells to combat disease. One well-known form is CAR T-cell therapy. This therapy involves collecting a patient's T cells, modifying them in the laboratory to better recognize cancer cells, and infusing them back into the patient.
The advantages of cell-based therapies are noticeable:
- Personalized Treatment: By using the patient's cells, the therapy can be tailored to their specific immune profile, improving its effectiveness.
- Durable Responses: These therapies can create a long-term immune memory against cancer, allowing the body to recognize and attack cancer cells that may reappear.
- Broad Application: Situtations like hematologic malignancies respond well to cell-based therapies, expanding the scope of treatable conditions.
Nevertheless, challenges exist. Potential side effects include cytokine release syndrome and neurological complications. Therefore, patient monitoring and management during and after treatment are crucial.
Monoclonal Antibodies
Monoclonal antibodies are another pillar of immunotherapy. These are laboratory-engineered molecules that can bind to specific targets on cells, often cancer cells. By doing this, they can obstruct growth signals or mark cancer cells for destruction by the immune system.
Some key features of monoclonal antibodies include:
- Targeted Therapy: They can be engineered to target over-expressed proteins in specific cancers, minimizing harm to normal cells.
- Combination Potential: Monoclonal antibodies may be combined with other treatment modalities to enhance overall effectiveness. This synergy can lead to better patient outcomes.
- Research and Development: Ongoing research continues to develop and refine these antibodies, broadening their application in various autoimmune disorders and infectious diseases as well.
Using monoclonal antibodies comes with considerations such as high costs and varying individual responses. Ongoing studies are essential to understand better their role in different therapeutic areas.
"Immunotherapy, including cell-based therapies and monoclonal antibodies, represents a significant shift in the landscape of treatment options, promising to harness and enhance the body’s natural defenses in adaptable and targeted ways."
In summary, advances in immunotherapy demonstrate a growing understanding of the biological immune system. This evolution enhances the precision and efficacy of treatments while emphasizing personalized approaches to individual patient care.
Future Challenges in Immunology
The landscape of immunology is rapidly evolving, influenced by advancements in technology and an increasing understanding of the immune system. Identifying and overcoming future challenges in this field is essential for various reasons. For one, the rise of new infectious diseases has highlighted vulnerabilities in current immune responses and medical interventions. Additionally, the growing interest in personalized medicine necessitates a closer examination of how individual immune profiles can be utilized for tailored treatments. Finally, understanding the microbiome's role in immune function could open new pathways for intervention and prevention strategies.
Emerging Infectious Diseases
Emerging infectious diseases represent a significant challenge for public health and the field of immunology. These diseases often arise unexpectedly, fueled by factors such as climate change, urbanization, and globalization. For example, viruses and bacteria can adapt and evolve, leading to new strains that may evade existing immune defenses. This dynamic nature of pathogens complicates the creation of effective vaccines and treatments.
Researchers must prioritize surveillance and rapid response systems to identify outbreaks and respond effectively. Developing vaccines that can adapt quickly to new strains or novel pathogens is equally critical, as demonstrated during the COVID-19 pandemic.
The Role of the Microbiome
The microbiome refers to the community of microorganisms residing in the human body, particularly in the gut. It plays a crucial role in shaping the immune system. A balanced microbiome supports immune health, while dysbiosis, or microbial imbalance, can lead to several health issues, including autoimmune diseases and allergies.
Understanding how the microbiome interacts with immune cells is fundamental for developing targeted therapies. Probiotics, prebiotics, and dietary interventions are areas of interest in this research. They may enhance immune resilience and reduce the incidence of immune-related diseases.
Personalized Medicine Approaches
Personalized medicine represents a paradigm shift in how medical treatments are developed and delivered. In immunology, this approach focuses on tailoring therapies to individual patients based on their specific genetic makeup and immune profiles.
Techniques such as genomic sequencing allow for a deeper understanding of an individual's immune system and its responses to various pathogens. This personalized approach holds the potential to revolutionize treatments for diseases such as cancer, autoimmune disorders, and allergies.
Investing in biomarkers and tools for individualized assessment of immune responses can lead to more effective treatments and better patient outcomes.
Optimizing vaccines for different population segments also falls under personalized medicine, ensuring that various demographic groups receive the most suitable interventions.
"As we advance into the future, the integration of genetic information, environmental factors, and microbial health into immunology will define the next frontier in medical science."
Closure
The biological immune system is a complex and vital component of our health, serving as the body's primary defense against pathogens. Understanding the intricacies of this system is crucial. In this article, we explored various aspects of the immune system, from its components and types of immunity to its functions and implications for disease and treatment. Each section provided insight into how immune cells and organs work together to identify and combat threats.
Moreover, the discussion on vaccines highlighted their importance in disease prevention and control. Immunotherapy represents an exciting frontier in medical science, offering new possibilities for treating diseases, including cancer.
Addressing future challenges in immunology is essential. As we face emerging infectious diseases and increasingly complex health situations, personalized medicine may offer tailored solutions. This journey into the immune system's mechanisms not only informs researchers and students but also shapes the public understanding of our health.
Understanding the immune system's function is not just for academics. It influences public health policies and individual health choices.
Hence, the collective knowledge of the immune system enhances our ability to fight diseases and promotes better health outcomes.
Summary of Key Points
In summary, several key points emerged from our exploration of the biological immune system:
- Components: The immune system comprises various cells, such as B cells and T cells, and organs, including the thymus and spleen.
- Types of Immunity: Both innate and adaptive immunity play significant roles in defending against pathogens.
- Functions: The primary functions include pathogen recognition, immune response activation, and the regulation of inflammation.
- Role of Vaccines: Vaccination is an effective method for enhancing immunity against specific diseases.
- Advances in Immunotherapy: Innovative therapies, such as cell-based treatments and monoclonal antibodies, are changing disease management.
- Future Directions: Ongoing research into microbial interactions and personalized strategies holds promise for improving health outcomes.
Future Directions in Immunology Research
The field of immunology is rapidly evolving, and future research will focus on several critical areas:
- Emerging Infectious Diseases: As new pathogens arise, understanding immune responses to these threats will be vital.
- Role of the Microbiome: The relationship between our gut bacteria and the immune system is an exciting area of study, potentially influencing how we approach health and disease.
- Personalized Medicine: Customizing immune therapies based on individual genetic makeup and environmental factors could enhance treatment efficacy.
These avenues promise to deepen our understanding of the immune system's function and expand therapeutic options, offering hope for innovative approaches to health challenges. By staying informed and engaged in this research, we position ourselves to better address the complex health issues of tomorrow.