CO2 Capture and Storage Technology: An In-Depth Analysis
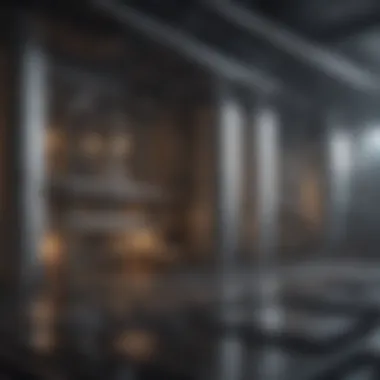
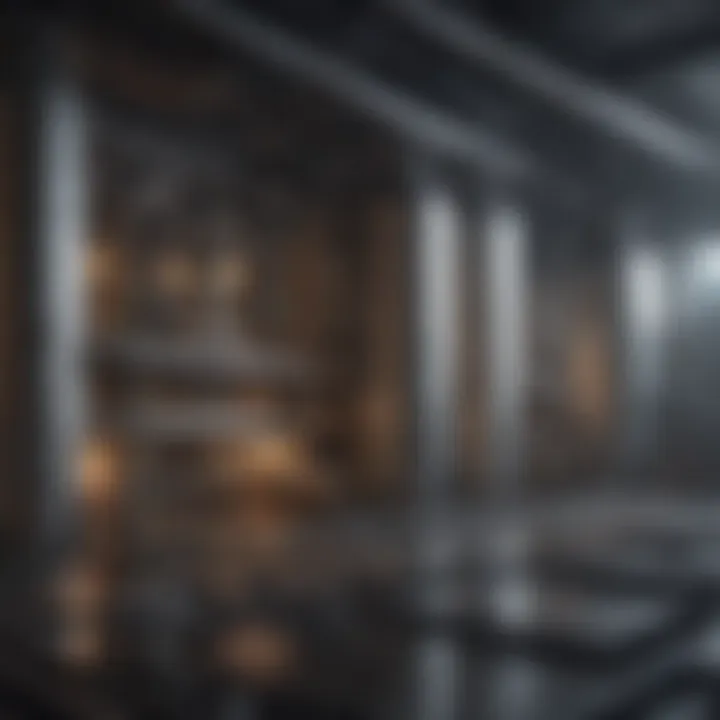
Intro
Carbon dioxide (CO2) capture and storage technology plays a crucial role in addressing the pressing issue of climate change. The increasing levels of atmospheric CO2 are directly linked to global warming, making it imperative to explore methods that can effectively sequester this greenhouse gas. This article aims to provide a comprehensive look into the processes associated with CO2 capture and storage, highlighting recent advances, methodologies, and the implications for environmental sustainability. By doing so, we aim to equip students, researchers, and professionals with meaningful insights into this evolving field.
The importance of CO2 capture and storage technology can not be overstated. As nations strive to meet their greenhouse gas emission targets, many find that direct methods of reducing emissions are often insufficient. As a result, CO2 capture and storage have emerged as viable strategies. This technology allows for the removal of CO2 from various sources, including power plants and industrial processes, before it is released into the atmosphere.
The journey into the realm of CO2 capture and storage begins with an exploration of recent advances in the field. Innovations continue to emerge, propelling the efficiency and effectiveness of capture methods, while also addressing the challenges that accompany large-scale deployment.
Recent Advances
Latest Discoveries
In recent years, researchers have made significant strides in optimizing capture processes. These discoveries span various domains, including materials engineering and process design. For example, advancements in sorbent materials, such as metal-organic frameworks, have shown promise in enhancing the efficiency of CO2 capture. This technology leverages highly porous structures that can selectively adsorb CO2, thus improving overall capture rates.
Another notable advancement is the integration of CO2 capture systems with renewable energy sources. The coupling of these systems not only aids in minimizing emissions but also promotes the utilization of green energy for power generation.
"The integration of CO2 capture with renewable energy sources represents a symbiotic relationship crucial for sustainable development."
Technological Innovations
Among the various capture methods, post-combustion capture stands out. Innovative designs in this area continue to enhance the viability of capturing CO2 from existing power plants. Techniques such as amine scrubbing have evolved, with newer formulations showing reduced energy consumption during the regeneration process.
Moreover, the development of direct air capture (DAC) technology is paving the way for more flexible solutions. DAC systems capture CO2 directly from the ambient air—creating potential for negative emissions. Their scalability is being evaluated, making them a topic of significant interest within academic and industry circles.
Methodology
Research Design
The examination of CO2 capture and storage technology employs a mixed-methods research design. Combining qualitative and quantitative approaches allows for a richer analysis of the challenges and advancements within the sector. Surveys distributed to industry professionals provide valuable insights into real-world applications, while case studies offer a deeper understanding of operational technologies in diverse contexts.
Data Collection Techniques
Data collection methods include academic literature reviews and interviews with experts in the field. By gathering information from various sources, a comprehensive overview of the current state of CO2 capture and storage technology is achievable. Furthermore, performance data from existing projects is analyzed to identify trends and patterns within implementation practices.
As we navigate through the challenges and prospects in this field, we aim to present a thorough analysis, contributing to informed discussions among students, researchers, and industry professionals.
Foreword to CO2 Capture and Storage Technology
The urgency of climate change mitigation has intensified discussions surrounding CO2 capture and storage technology. This topic is critical as it presents a pragmatic approach to reducing greenhouse gas emissions, particularly carbon dioxide, from industrial processes and energy production. As society grapples with climate impacts, understanding CO2 capture and storage becomes essential for developing comprehensive environmental strategies.
Definition and Importance
CO2 capture and storage technology refers to the processes that capture carbon dioxide emissions from sources like power plants and industrial facilities, preventing the gas from entering the atmosphere. The captured CO2 can then be stored underground in geological formations or utilized in various applications. The importance of this technology lies in its potential to significantly lower the carbon footprint of major emitters, thus contributing to global efforts against climate change.
- Significantly reduces greenhouse gas emissions.
- Supports compliance with regulatory frameworks aimed at climate goals.
- Provides a path for industries dependent on fossil fuels to transition towards sustainability.
Employing these technologies can offer industries an advantage by aligning with emerging environmental standards and consumer preferences, highlighting the importance of investing in CO2 capture and storage.
Historical Context
The roots of CO2 capture and storage technology can be traced back several decades. Its development was primarily driven by the need to address environmental concerns associated with rising CO2 levels. Early research focused on enhancing the energy efficiency of fossil fuel use while simultaneously seeking methods to mitigate CO2 emissions. The first major project was the Sleipner CO2 storage project in Norway, initiated in 1996, which successfully demonstrated the feasibility of storing CO2 in deep saline aquifers.
Since then, various advancements have occurred:
- 2000s: Interest grew as nations recognized the potential for carbon capture to play a role in energy transition strategies.
- 2010s: More projects emerged globally, showcasing innovations in capture technologies, including post-combustion methods.
- Present: Current efforts focus on integrating CO2 capture in large-scale industrial applications and the development of policies to support these initiatives.
Understanding the historical context provides insight into the evolution of CO2 capture and storage technology, laying the groundwork for its significance in today's climate strategies. As countries adopt measures to achieve net-zero emissions, the technology's relevance continues to grow.
Mechanisms of CO2 Capture
Understanding the mechanisms of CO2 capture is essential in the effort to combat climate change. These mechanisms provide pathways to efficiently separate carbon dioxide from other gases. This article discusses three main methods: post-combustion capture, pre-combustion capture, and oxy-fuel combustion. Each has unique characteristics and potential advantages that can be leveraged in various applications.
Post-Combustion Capture
Post-combustion capture is a technique used to remove CO2 from flue gases after fossil fuels have been burned. It operates on the principle of treating exhaust gases to reduce their carbon footprint.
This method is particularly significant as it can be retrofitted into existing power plants, allowing older facilities to adopt carbon capture technology without needing to undergo extensive redesigns. In many cases, this makes it a more financially viable option for reducing emissions in the short term.
The process generally utilizes amine solvents, which selectively absorb CO2 from other gases. Once absorbed, the CO2 is heated to release it from the solvent, allowing for potential reuse or storage. The technology is promising, but it also presents challenges such as:
- Energy consumption during regeneration of the absorbent.
- The need to manage solvent degradation effectively.
- Potential impacts on the profitability of energy generation.
By addressing these challenges, post-combustion capture can gain traction as a pivotal method in the broader scope of carbon reduction initiatives.
Pre-Combustion Capture
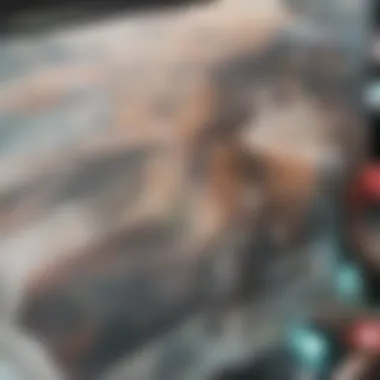
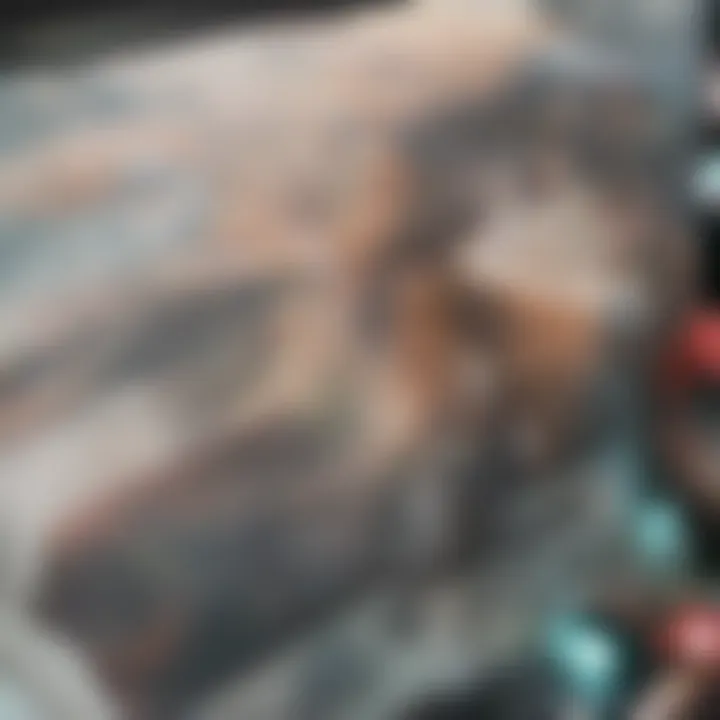
Pre-combustion capture differs fundamentally from post-combustion capture. Instead of treating exhaust gases, this method captures CO2 before combustion occurs. This is typically achieved by converting fossil fuels into a mixture of hydrogen and carbon dioxide.
The separation occurs before burning fuels, optimizing the carbon capture process. This produces a high-purity hydrogen fuel, which can serve as an energy source with lower greenhouse gas emissions.
Some benefits include:
- Greater efficiency in capturing CO2 compared to post-combustion methods.
- Production of clean hydrogen, which is increasingly important in the transition to renewable energy systems.
However, this method requires substantial infrastructure investments, such as capturing facilities integrated with gasification units. This may limit its implementation in specific contexts or regions where such technology is not yet established.
Oxy-Fuel Combustion
Oxy-fuel combustion is an advanced process that involves burning fuel in an oxygen-rich environment instead of air. This results in a flue gas that is almost entirely composed of water vapor and CO2, simplifying the separation process.
The primary advantage of oxy-fuel combustion is that it reduces nitrogen content in the exhaust, facilitating easier CO2 capture and making the cooling and separation processes more efficient. Some design elements of oxy-fuel systems include:
- Use of cryogenic air separation technologies.
- Adoption of high-performance combustion turbines.
Despite its advantages, oxy-fuel combustion faces numerous challenges, including increased costs for oxygen production and technical complications in boiler design. Addressing these issues will be crucial for optimizing the use of this capture method in a sustainable energy framework.
Understanding these mechanisms is not merely academic; it is fundamentally linked to the success of global initiatives aimed at reducing atmospheric CO2 levels and mitigating climate change effects.
Technologies for CO2 Storage
Understanding the technologies for CO2 storage is crucial in the context of combatting climate change. These technologies focus on safely storing carbon dioxide emissions captured from various sources. The overall goal is to minimize the amount of CO2 that enters the atmosphere. This section explores three main storage methods: geological storage, ocean storage, and mineralization. Each method has its own set of advantages and challenges, impacting their applicability and effectiveness.
Geological Storage
Geological storage involves isolating CO2 in deep underground rock formations, which has gained attention due to its potential for large-scale storage of carbon dioxide. The capability to store large amounts makes it an attractive choice. Geologic formations can provide a long-term solution to CO2 emissions.
Types of Geological Formations
Geological formations suitable for CO2 storage include saline aquifers, depleted oil and gas fields, and unmineable coal seams. Saline aquifers are often the most prominent choice due to their widespread availability and capacity to store large volumes of CO2. These formations are typically located deep beneath the earth's surface.
The key characteristic of saline aquifers is their porosity and permeability. This means they can hold fluids like CO2. The unique feature of using saline aquifers is their ability to contain CO2 over long periods, which is essential for effective climate strategies. However, while the potential is high, monitoring leakage is important to ensure environmental safety.
Potential Risks and Mitigation Strategies
Storing CO2 underground comes with risks, which include leakage and induced seismicity. Leakage can allow CO2 to escape back into the atmosphere, negating the benefits of capture. The unique risk of induced seismicity involves generating small earthquakes due to reservoir pressure changes.
To mitigate these risks, rigorous monitoring systems must be established. Strategies include utilizing seismic imaging and pressure monitoring within storage sites to detect any abnormalities early. This case emphasizes the importance of ongoing research in risk assessment and the development of advanced technology to ensure the safety of geological storage methods.
Ocean Storage
Ocean storage refers to the concept of directly injecting captured CO2 into oceans. As a large carbon sink, oceans theoretically can absorb substantial amounts of carbon dioxide. However, the implications of altering ocean chemistry are complex and raise environmental concerns that require further research.
Mineralization
Mineralization involves converting CO2 into stable minerals through chemical reactions with naturally occurring minerals. This process offers a permanent solution to CO2 storage. One beneficial aspect is the potential to utilize local materials, thus cutting costs related to transportation. The main disadvantage, however, lies in the slower reaction times compared to other storage methods.
Mineralization can provide a dual benefit by creating valuable materials while reducing CO2 levels, supporting long-term climate goals.
Overall, the technologies discussed here present both opportunities and challenges in the effort to mitigate climate change effectively. Each method plays a unique role, and understanding their characteristics is vital in choosing appropriate strategies in environmental action.
Economic Considerations
Economic considerations play a crucial role in the development and deployment of CO2 capture and storage technology. The financial viability of these technologies not only influences investment decisions but also affects the broader acceptance and implementation in various industries. Understanding the costs associated with capturing and storing carbon dioxide is essential for policymakers, researchers, and industry professionals. It also contributes greatly to the discussions surrounding sustainability and climate change mitigation efforts.
Cost of Implementation
The cost of implementing CO2 capture and storage systems varies significantly. Several factors influence these costs, including the specific technology employed, the scale of the operation, and the geographic location of facilities. On average, the costs can be categorized into capital expenses, operational expenses, and maintenance.
- Capital Expenses: These are the upfront investments needed to establish the capture and storage infrastructure. Technologies like post-combustion capture or geological storage requires significant initial funding.
- Operational Expenses: Ongoing costs after the infrastructure is established include energy requirements, personnel wages, and other operational factors.
- Maintenance: Regular upkeep ensures the system functions efficiently and safely, avoiding costly repairs and downtime.
Understanding these financial aspects is vital for stakeholders seeking to promote CO2 capture and storage as a sustainable practice.
Economic Incentives
Carbon Pricing
Carbon pricing serves as a market-based approach to reducing greenhouse gas emissions. It assigns a cost to carbon emissions, encouraging industries to adopt more efficient systems and invest in CO2 capture technologies.
- Key Characteristic: The foundational aspect of carbon pricing is to create a direct financial incentive for emitters to reduce their carbon footprint. By making emissions costly, it motivates businesses to integrate cleaner technologies, such as CO2 capture.
- Benefits: Carbon pricing can significantly influence corporate investment strategies. Companies seeking to minimize expenses may shift toward adopting carbon capture solutions. This creates a financial case for investing in sustainable technologies.
- Unique Feature: A notable feature of carbon pricing is its adaptability. It can be designed with varying levels of stringency, allowing governments to adjust the pricing based on emissions reduction targets and economic contexts.
Government Subsidies
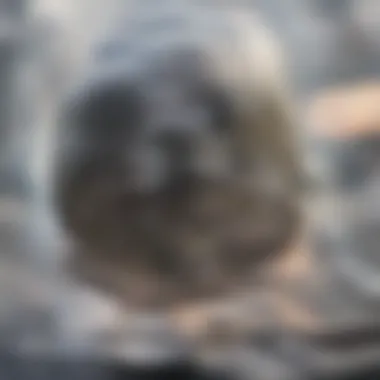
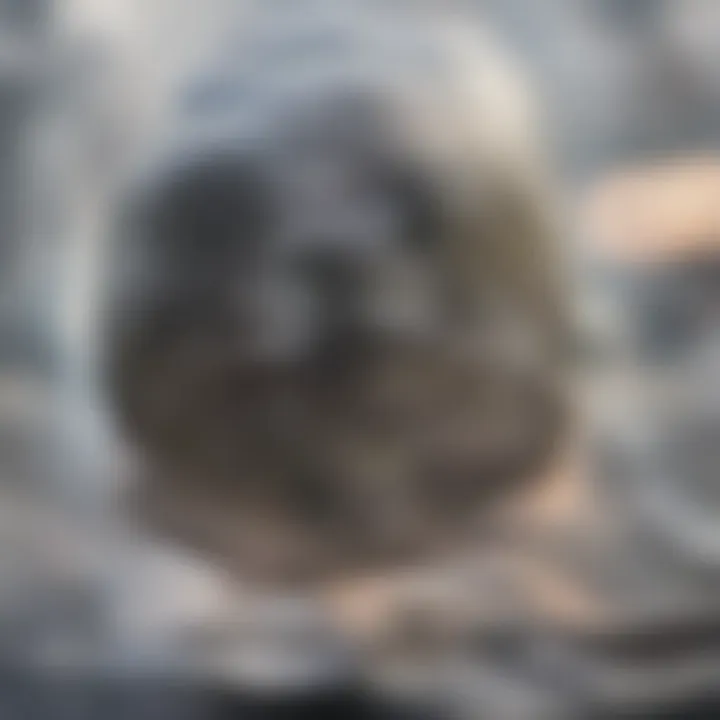
Government subsidies represent another crucial economic incentive for CO2 capture and storage. These financial aids lower the burden on companies developing and implementing advanced capture technologies.
- Key Characteristic: Subsidies can take various forms, including direct funding, tax breaks, or research grants. These approaches help alleviate the costs associated with CO2 capture initiatives, making them more feasible for companies, especially startups.
- Benefits: By reducing financial risks, subsidies can accelerate the adoption of CO2 capture technologies. This approach attracts more investments and drives innovation within the sector.
- Unique Feature: Government subsidies often target specific projects or technologies, encouraging the development of solutions that align with broader environmental goals.
Both carbon pricing and government subsidies present opportunities and challenges, highlighting the money factor in CO2 capture and storage technologies. Understanding these economic considerations can facilitate informed decision making regarding climate strategies moving forward.
Regulatory Frameworks
Regulatory frameworks play a vital role in shaping the development and implementation of CO2 capture and storage technologies. They create the guidelines and standards necessary for ensuring safety, environmental protection, and economic viability. These frameworks can influence the pace at which technologies are adopted and deployed, as they determine the regulations that industries must follow in order to comply with national and international environmental standards. A well-structured regulatory framework not only ensures that the technology is deployed safely but also addresses public concerns regarding its impacts on health and ecosystems.
Key Benefits of Regulatory Frameworks:
- Safety Assurance: Regulations help in establishing safety protocols for CO2 capture and storage processes.
- Environmental Protection: These frameworks set limits and requirements aimed at minimizing environmental impacts.
- Market Confidence: Clear regulations can enhance investor confidence by providing legal certainty and stability in the market.
- Public Trust: By demonstrating commitment to safety and environmental integrity, regulatory frameworks can help build public acceptance for these technologies.
National Policies
National policies are the backbone of regulatory frameworks at a country-level. Each nation has unique energy, economic, and environmental contexts that influence its approach to carbon management. For CO2 capture and storage technologies, national policies can include incentives for research and development, funding for pilot projects, and support for commercial-scale applications. These policies are essential for fostering innovation and attracting investments in this emerging field.
Most countries recognize the need to reduce greenhouse gas emissions. National policies can reflect this recognition by establishing specific targets and regulations related to CO2 emissions. For example, countries may impose strict emissions limits for industrial activities, providing a strong incentive for these entities to invest in carbon capture technology.
International Agreements
International agreements create a broader framework for addressing climate change on a global scale. They bring together countries to align their efforts toward reducing carbon emissions and fostering sustainable practices. Such agreements can significantly impact CO2 capture and storage technologies by encouraging cooperative research initiatives, sharing best practices, and mobilizing funding for climate mitigation projects.
One notable example is the Paris Agreement, which commits countries to limit global warming. This agreement emphasizes the importance of reducing emissions and encourages nations to invest in innovative technologies, including carbon capture and storage. Through these international frameworks, countries are pressured to adopt cleaner technologies, thereby fostering a collaborative approach toward tackling climate change.
"Regulatory frameworks are essential for establishing a systematic approach to CO2 capture and storage."
Environmental Impacts
Understanding the environmental impacts of CO2 capture and storage technology is crucial for assessing its overall effectiveness and sustainability. This section explores two significant facets: ecosystem effects and long-term sustainability. As society shifts towards minimizing greenhouse gas emissions, the implications on various ecosystems and the sustainability of these technologies warrant careful consideration.
Ecosystem Effects
The deployment of CO2 capture and storage technologies can have varied effects on ecosystems. It is essential to analyze how carbon dioxide sequestration might influence local flora and fauna. The choice of geological formations or ocean locations for storage can affect both terrestrial and aquatic ecosystems.
- Geological Storage: Storing CO2 underground will alter the geological pressure within formations. This can lead to changes in local ecosystems, especially if the stored CO2 leaks, affecting groundwater quality and plant life in the area. If CO2 seeps into aquifers, the adjacent ecosystems may suffer from increased acidity, fundamentally altering species compositions.
- Ocean Storage: Ocean-based storage methods can impact marine life. Larger volumes of CO2 could lead to local changes in water chemistry, potentially disrupting food chains. For instance, increased acidity from dissolved CO2 can impact shellfish and coral reefs, which are sensitive to pH changes. The broader implications for marine ecosystems depend on the concentration and geographical spread of stored CO2.
In summary, while CO2 capture aims to mitigate climate change, potential adverse effects on ecosystems must be carefully monitored and managed.
Long-Term Sustainability
The concept of long-term sustainability in CO2 capture and storage centers on the effectiveness and safety of these systems over extended periods. It evaluates whether these technologies can contribute effectively to climate goals without introducing new environmental risks.
- Monitoring and Regulations: Sustainable CO2 storage requires a robust framework for monitoring to ensure no leakages occur. Continuous evaluation helps maintain public trust and ensures compliance with regulatory standards.
- Lifecycle Analysis: Assessing the full lifecycle of CO2 capture and storage can inform its sustainability. This includes understanding the energy expenditures during CO2 capture, transport, and storage processes. If the process consumes more energy than it mitigates than it creates a net negative effect.
- Integration with Other Solutions: For CO2 capture and storage technology to be truly sustainable, it may need to be integrated with other climate strategies, such as renewable energy production and emissions reduction. Collaboration among these approaches can enhance the overall effectiveness, making it a cornerstone in climate mitigation efforts.
"The success of CO2 capture and storage technology lies in its ability to operate securely and sustainably over generations, promising a healthier planet for future populations."
Through careful consideration of ecosystem impacts and long-term viability, we can ensure that CO2 capture and storage strategies serve not only immediate climate goals but also contribute positively to the environment.
Technological Innovations
Technological innovations play a crucial role in the advancement of CO2 capture and storage technology. They enable improvements in efficacy and cost-effectiveness while leveraging new scientific insights for better outcomes. This section highlights emerging technologies and trends in research and development that are reshaping the landscape of carbon management.
Emerging Technologies
Emerging technologies in CO2 capture and storage comprise a variety of methods and tools that enhance the efficiency of carbon dioxide management. These innovations stem from significant research initiatives, focusing on integrating new materials and methodologies.
- Nanomaterials have shown promise in improving the efficiency of CO2 absorption. For instance, metal-organic frameworks (MOFs) can selectively capture carbon dioxide from industrial emissions, offering high surface areas and tunable properties.
- Biological approaches, such as algae cultivation and other bioengineered micro-organisms, contribute to effective carbon capture. These systems use photosynthesis to absorb CO2 while producing biomass that can be converted into fuel or other products.
- Direct Air Capture (DAC) technologies allow for the extraction of CO2 from the atmosphere. The development of scalable units that employ chemical pathways to isolate carbon dioxide enhances prospects for global carbon neutrality.
These technologies not only aim to capture more CO2 but also attempt to make the process economically viable.
Research and Development Trends
Research and development trends point to a growing focus on improving existing technologies and understanding the broader implications of CO2 capture and storage. Key areas of interest include:
- Integration with renewable energy sources: The coupling of carbon capture with renewable energy sources, like wind and solar, aims to optimize energy use while reducing carbon emissions. The goal is to develop systems that utilize renewable energy for powering capture processes.
- Innovative modeling and simulation techniques: Enhanced computational models are emerging to predict the behavior of CO2 in various storage environments. These models can inform decisions on site selection and long-term monitoring, which are crucial for successful storage strategies.
- Life Cycle Assessments (LCAs): Evaluating the environmental impacts of CO2 capture and storage technologies promotes understanding of their full implications. Researchers are increasingly conducting LCAs to provide insights into life-cycle greenhouse gas emissions and resource use.
- Collaborative initiatives: Partnerships among academia, industry, and government are becoming more common. These collaborations push the boundaries of research and accelerate the development of novel solutions.
Investing in R&D for CO2 capture technologies has the potential to unlock significant benefits for environmental sustainability and economic growth.
Overall, the trends in research and emerging technologies signal a positive trajectory toward effective CO2 capture and storage solutions, crucial for combating climate change.
Challenges and Barriers
The journey towards the efficient implementation of CO2 capture and storage technology is marred with various challenges and barriers. Understanding these obstacles is not just an academic exercise; it is critical for policymakers and industry leaders aiming to develop sustainable climate strategies. Gaining insight into these factors helps build a clearer path for technological development and public acceptance.
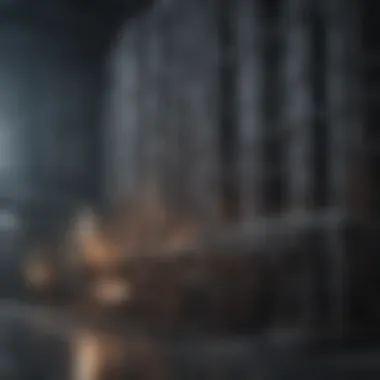
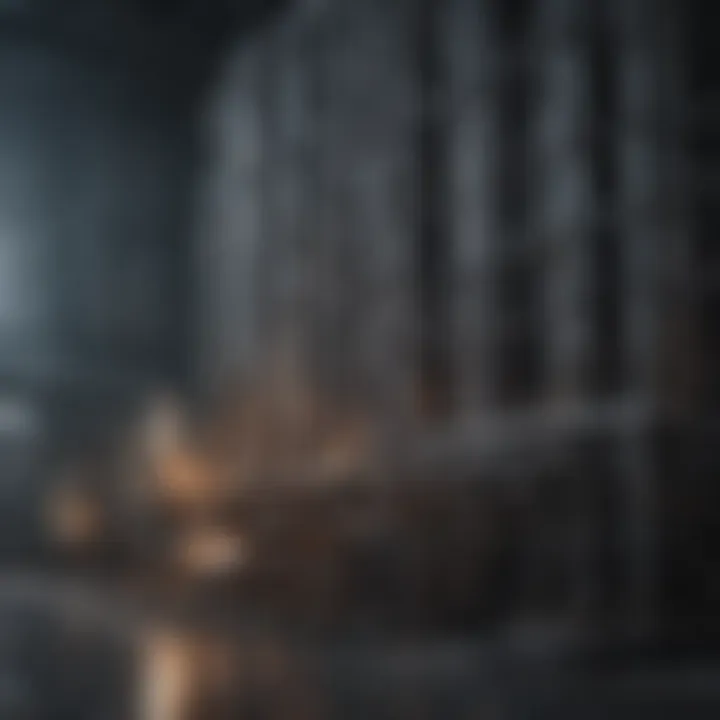
Technological Challenges
Advancements in CO2 capture and storage technology face numerous technological hurdles. These challenges can impede the adoption of this technology at a broader scale.
- Efficiency Issues: Many current capture methods do not achieve high efficiency, meaning that significant amounts of CO2 can remain uncaptured. Innovations like solvent selection and process optimization are crucial, yet they remain in various stages of development.
- Scale of Infrastructure: Transitioning from pilot projects to large-scale implementations requires substantial infrastructure investment. Building this capacity is both costly and logistically challenging, especially in regions that lack robust energy frameworks.
- Durability and Safety: The long-term storage of CO2 demands monitoring for potential leaks. Ensuring the integrity of geological formations and the development of effective monitoring systems is essential. Failure in these areas poses significant risks to both the environment and public health.
Emerging technologies, thus far, have demonstrated promise, but challenges in scalability, efficiency, and safety must be systematically addressed. Continued research in these areas is essential to enhance the technological foundation of CO2 capture and storage.
Public Acceptance
Public acceptance is another significant barrier to the widespread adoption of CO2 capture and storage technology. Engaging the public effectively can determine the success of various initiatives.
- Awareness and Understanding: There is a general lack of awareness surrounding CO2 capture and storage. Educational campaigns highlighting the technology's benefits and safety measures can help alleviate misconceptions.
- Perception of Risk: Many people fear potential risks associated with underground storage sites. Addressing concerns about leaks or environmental impacts is paramount. Transparency in processes and dedicated risk communication strategies can foster trust.
- Community Involvement: Local communities must feel included in decision-making processes regarding projects in their vicinity. Engaging stakeholders in discussions about community benefits and safety can enhance acceptance.
Public skepticism can stall progress, emphasizing the need for clear communication and community engagement strategies.
Thus, bridging the gap between technology and public perception is key. Public acceptance is often influenced by both trust and knowledge, making these factors essential for fostering understanding and support.
In summary, the challenges and barriers to CO2 capture and storage technology are multi-faceted. Tackling technological difficulties while simultaneously gaining public trust is crucial for generating momentum in climate change mitigation efforts.
Case Studies
Case studies in CO2 capture and storage technology play a critical role in understanding its practical application and efficacy. They offer real-world insights into how various methods are being implemented across different regions and industries. Analyzed projects can highlight both the successes and the challenges faced, providing a necessary framework for evaluating the technology's potential. Case studies also foster knowledge sharing among peers in academia, industries, and policy-making circles, ultimately promoting innovation and improving best practices in deploying these technologies.
Successful Projects
Many successful projects around the world have showcased how CO2 capture and storage can be effective in addressing emissions. One notable example is the Boundary Dam project in Canada. This initiative uses a post-combustion carbon capture system, and it has successfully captured over a million tons of CO2 annually since its launch. The collected CO2 is then utilized for enhanced oil recovery, demonstrating economic viability alongside environmental responsibility.
Another significant project is Sleipner, located in Norway. It was the first commercial project to store CO2 in a saline aquifer. Since 1996, Sleipner has safely stored approximately 1 million tons of CO2 each year beneath the North Sea. This project serves as a valuable example of geological storage technology's reliability.
These projects provide a blueprint for future initiatives. They illustrate that CO2 capture and storage is not only feasible but can operate within various frameworks, such as enhanced oil recovery and long-term geological storage.
Lessons Learned
Lessons from these projects are invaluable for advancing CO2 capture and storage technologies. Firstly, the importance of stakeholder engagement cannot be overstated. Projects that involve local communities and understand public perceptions tend to gain wider acceptance and support. The Sleipner project, for instance, has consistently engaged local stakeholders, ensuring transparency and fostering trust.
Secondly, economic aspects like cost management and financing strategies are crucial. Successful projects often employ innovative financing models that lessen the financial burden on initial investments. The Boundary Dam project, for example, benefited from a combination of public funding and support from private sectors, creating a more robust financial structure.
Finally, the necessity of continuous monitoring is evident in these case studies. Effective CO2 storage requires ongoing assessment to ensure safety and for verifying that the stored CO2 remains contained. Lessons learned emphasize that a proactive monitoring strategy leads to greater public confidence and enhanced regulatory compliance.
In summary, case studies of successful projects in CO2 capture and storage technology offer a wealth of knowledge. They not only demonstrate the viability of these methods but also highlight essential strategies needed to overcome obstacles in this critical field.
Future Directions
The future of CO2 capture and storage technology is critical for several reasons. First, the increasing need for effective climate change mitigation strategies highlights the urgent requirement for innovations in this field. As global temperatures rise, reducing atmospheric carbon dioxide is vital. CO2 capture and storage can play a significant role by offering a way to lower emissions from various industries.
Moreover, improving the technology itself can lead to efficiencies that make it more cost-effective. Cost reductions in capturing CO2 could enhance its attractiveness to industries aiming to comply with emissions regulations. Furthermore, new advancements can potentially broaden the scope of suitable applications, allowing for a wider array of facilities to adopt these methods.
Future Research Needs
Research is essential to advance CO2 capture and storage technologies. Areas requiring focus include:
- Material Development: Research should explore new materials for CO2 adsorption that could enhance capture efficiency.
- Process Optimization: Improving existing processes can significantly increase yield while reducing energy consumption.
- Monitoring and Verification: For successful geological storage, better monitoring technologies must be developed to ensure that stored CO2 remains contained and does not leak.
"Investing in research offers not only the potential for technological advancements but also economic benefits through job creation in emerging sectors."
- Field Studies: Practical field studies are necessary to evaluate the long-term viability of different storage sites and the effectiveness of capture technologies.
Role in Climate Strategies
CO2 capture and storage has a pivotal role in global climate strategies. It aligns with various national and international emissions targets aimed at limiting global warming. By capturing CO2 emissions from point sources, we can create a balance against the emissions produced through fossil fuel use.
- Complementing Renewables: As the world increasingly relies on renewable energy, CO2 capture can complement these technologies by addressing emissions from harder-to-decarbonize sectors such as heavy industry and aviation.
- Negative Emissions Technologies: Advancements in CO2 capture and storage can also lead to negative emissions technologies. These methods can capture more CO2 than is emitted, contributing positively to reversing current atmospheric carbon levels.
Epilogue
The exploration of CO2 capture and storage technology reveals its critical role in addressing climate change. This conclusion synthesizes the insights gathered throughout the article, emphasizing the mechanism's importance and the broader implications for society.
Summary of Findings
Throughout this article, several key aspects of CO2 capture and storage have been discussed. The various capture methods, including post-combustion, pre-combustion, and oxy-fuel combustion, showcase the technological diversity available. Each method offers unique advantages and challenges, which must be carefully considered in practical applications. Moreover, understanding the different storage options, such as geological formations, ocean storage, and mineralization, is vital in assessing their long-term viability and safety.
Economic considerations, including implementation costs, incentives like carbon pricing, and government subsidies, play a large role in shaping the feasibility of these technologies. Regulatory frameworks help ensure safety and efficacy, which links back to public acceptance—a recurring challenge faced by initiatives in this field.
Research and development are paramount in improving existing technologies and uncovering new methods. Successful projects provide valuable case studies, helping to identify best practices and lessons learned that inform future efforts in this area.
Final Thoughts
CO2 capture and storage technology stands at the forefront of efforts to mitigate climate change. Its successful deployment could significantly decrease atmospheric carbon levels and help nations meet their emissions targets. However, this potential is contingent upon solving existing challenges, including technological hurdles, economic feasibility, and societal acceptance.
The future of CO2 capture and storage requires concerted efforts from policymakers, researchers, and industry leaders. Only through collaboration and innovation can the ambitions surrounding these technologies be realized. Adequate investment in research and development will be essential in advancing the technology further.