Coherent Optics Principles and Applications Explained
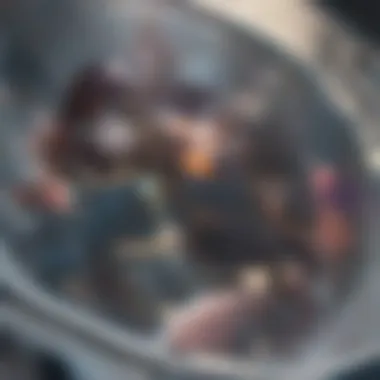
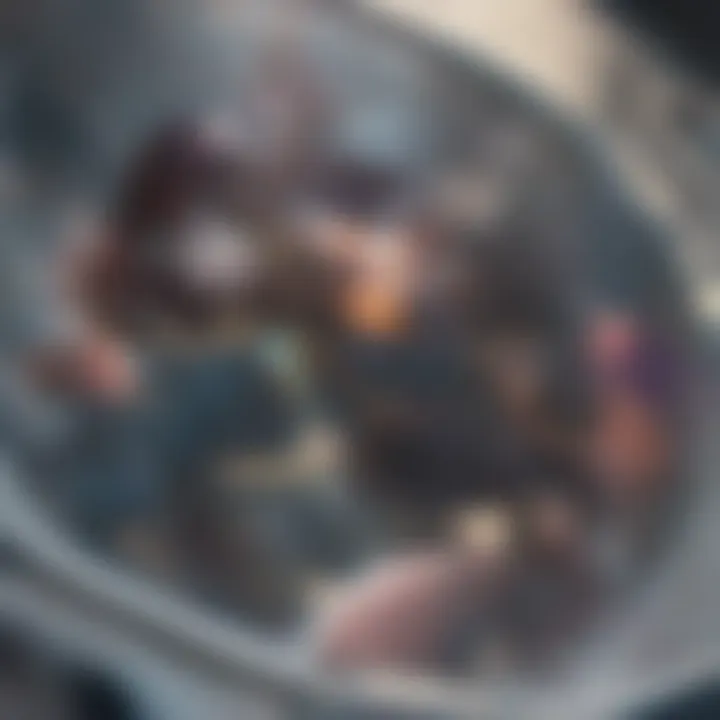
Intro
Coherent optics is a critical field in modern science and technology. It employs coherent light sources, such as lasers, to manipulate and analyze light waves. This technique allows for precise control over the light's properties, making it essential in several applications. Its principles extend beyond traditional optics, ushering in significant advancements across various industries.
In this article, we will explore the fundamental aspects of coherent optics, examining its core principles and diverse applications. This includes its impact on optical imaging, telecommunications, and metrology. Understanding the relationship between coherence and these applications provides insight into how coherent optics fosters technological innovation and scientific exploration.
Recent Advances
Recent developments in coherent optics have propelled the field forward, revealing new potential applications and improving existing technologies.
Latest Discoveries
Research in coherent optics has led to significant discoveries. For instance, scientists have uncovered new methods of improving the stability of laser beams, which is crucial in applications requiring high precision. Another area of advancement involves quantum optics, where researchers are exploring entangled light particles to enhance communication systems. This research opens the door to quantum-based telecommunications, promising faster and more secure data transmission.
Technological Innovations
Technological advancements have played a crucial role in coherent optics. One such innovation is the development of optical coherence tomography (OCT). OCT is widely used in medical imaging, particularly for eye examinations. It allows for non-invasive imaging of the retina with high resolution. Additionally, the emergence of photonic computing utilizes principles of coherent optics, aiming to perform calculations faster than electrical systems by leveraging light.
Methodology
An understanding of the methodology employed in coherent optics research reveals how discoveries are made and technologies developed.
Research Design
Most experimental designs in coherent optics are based on controlled laboratory settings. Researchers often focus on parameters like light coherence length, wavelength, and interference patterns. These are essential in testing hypotheses about light behavior and establishing coherence properties in various materials.
Data Collection Techniques
Data collection techniques range from basic measurements to advanced imaging technologies. Standard methods often include interferometry and spectrometry. Interferometry provides information about light wave phase shifts, critical for understanding coherence.
In summary, coherent optics stands as a vital field, intersecting with numerous technological domains. The continuous advances and research methodologies not only deepen our theoretical knowledge but also enhance practical applications, impacting various industries.
Prelude to Coherent Optics
Coherent optics represents a vital field in modern science and technology, focusing on the manipulation and application of light waves that exhibit a consistent phase relationship. This introduction provides context to its significance. Coherent light sources, primarily lasers, are essential in numerous applications. These include imaging techniques, telecommunications, and precision measurement systems, making coherent optics a cornerstone for various industries.
The importance of coherent optics lies in its ability to produce high-quality images and signals. Through the use of coherent light, professionals can achieve enhanced resolution and clarity in optical systems. This capability not only improves the effectiveness of imaging devices but also reduces noise, allowing for more precise data interpretation.
Definition of Coherent Light
Coherent light is characterized by waves that maintain a fixed phase relationship over time. This coherence can be temporal or spatial, each playing a critical role in how light behaves. For instance, laser light is a prime example of coherent light, as it emits waves that are both monochromatic and in phase. Unlike incoherent light sources, such as incandescent bulbs, coherent light offers significant advantages in applications requiring precision.
In many cases, coherent light enhances the performance of optical instruments. For imaging devices, coherent light can lead to better focus and depth of field. In telecommunications, it allows for higher data transmission rates by reducing dispersion and increasing signal fidelity, which is crucial for modern internet infrastructure.
Historical Context
Understanding the journey of coherent optics provides insight into its present applications and future potential. The concept of coherent light dates back to the early 20th century with the development of quantum theory. However, it wasn't until the invention of the laser in the 1960s that coherent optics gained significant traction. The first working laser was created by Theodore Maiman in 1960, which opened doors for numerous scientific and technological advancements.
The rise of coherent optics was marked by several key developments:
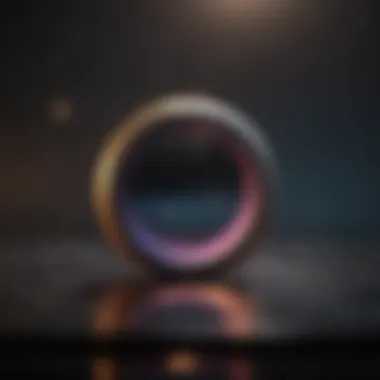
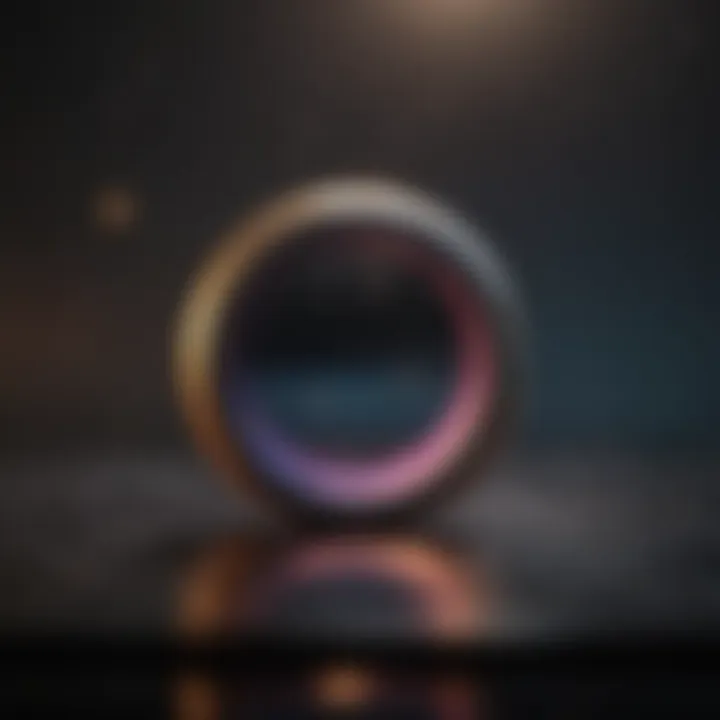
- The introduction of holography, allowing for the recording and reconstruction of light fields.
- Innovations in laser technology leading to various applications in medicine, communication, and manufacturing.
- The integration of coherent optics in research fields like astrophysics and metrology, enhancing the capability to measure distances with great precision.
These historic advancements demonstrate the profound impact coherent optics has had on technology and science, paving the way for current and future innovations.
Fundamental Principles of Coherence
Understanding the fundamental principles of coherence is critical for grasping the intricacies of coherent optics. Coherence refers to the correlation between light waves in terms of phase and frequency. It plays a significant role in developing technologies and applications that rely on the behavior of light. By grasping these principles, one can appreciate how coherent light enhances imaging techniques, improves communication systems, and facilitates precise measurements.
Temporal Coherence
Temporal coherence describes the correlation of light waves over time. It defines how consistent the phase of a light wave remains as it travels and can be quantified by the coherence time. This time provides insight into how long light waves maintain their phase relationship. In practical terms, sources with high temporal coherence, like lasers, generate light that exhibits consistent frequencies and predictable behavior over longer durations. This is essential in applications such as interferometry, where precise phase measurement is crucial.
Spatial Coherence
Spatial coherence refers to the degree of phase correlation between different points in a beam of light at a specific instant. It is measured by considering the coherence length, which signifies how far the light travels before losing its wavefront uniformity. High spatial coherence is characteristic of laser light. This property allows coherent light sources to produce sharp, distinct images in optical systems. Photonic applications like holography leverage this concept to create realistic three-dimensional images. Understanding spatial coherence is thus essential for developing technologies in imaging and laser applications.
Measurement of Coherence Length
Coherence length is a vital parameter that quantifies how far the waves can travel while remaining coherent. It is directly related to both spatial and temporal coherence. The longer the coherence length, the more suitable the light source is for applications requiring extensive phase relationships over long distances. Measuring coherence length involves techniques such as using Michelson interferometers. This measurement provides key insights for researchers working on advanced optical systems. Understanding and accurately determining coherence length can significantly impact the design and functionality of optical devices.
Light Sources and Coherence
The role of light sources in coherent optics cannot be overstated. Coherent light is essential for various applications, influencing precision and performance in multiple fields. Understanding light sources enables us to appreciate their significance in applications like optical imaging, telecommunications, and metrology. This section will delve into the specifics of laser technology and compare it to non-coherent light sources, establishing clear distinctions and implications for their use.
Laser Technology Overview
Lasers, or Light Amplification by Stimulated Emission of Radiation, serve as the cornerstone of coherent optics. These devices emit light that is characterized by high coherence, monochromaticity, and directionality. The hearts of lasers are stimulated emission processes, where excited atoms or molecules release photons in phase with one another. The critical aspects of laser technology include:
- High Coherence: Lasers produce light where the phases of the wavefronts are locked together, ensuring uniformity over a distance.
- Monochromatic Light: The light emitted arises from a narrow range of wavelengths, which enhances its precision in applications.
- Directional Emission: Unlike most light sources, lasers emit light in a focused beam, enabling effective transmission and application in various scenarios.
These properties make lasers invaluable for many industries. Common usages include telecommunications, where light signals travel through fiber-optic cables, and in imaging techniques, including holography.
Comparison to Non-Coherent Light Sources
In contrast, non-coherent light sources, like incandescent bulbs and fluorescent lamps, exhibit significant differences from lasers. Understanding these differences helps elucidate the unique advantages lasers provide.
- Phase Relationship: Non-coherent sources emit light without a fixed phase relationship. The emitted light comprises multiple wavelengths and is uncorrelated, leading to a lack of coherence over distance.
- Broad Spectrum: These light sources typically produce a wide range of wavelengths, making them more suitable for general illumination but less effective for applications needing precision.
- Diffusion: Non-coherent light spreads out over broader areas, thus losing intensity. This characteristic limits their feasibility in applications requiring focused and coherent light.
In summary, while non-coherent light sources have their applications mainly in general lighting and display technologies, lasers effectively meet the stringent demands of coherent optics.
"The unique properties of laser light permit innovation in imaging, communications, and metrology that traditional light sources cannot achieve."
Understanding these differences between coherent and non-coherent light sources allows for informed choices in selecting appropriate technologies for specific applications. The transition towards more sophisticated laser-based solutions continues to drive advancements across diverse fields.
Applications of Coherent Optics
Coherent optics has become an essential field due to its wide range of applications across various industries. Its significance lies not only in theoretical frameworks but also in practical implementations. The manipulation of coherent light opens up new possibilities in imaging, telecommunications, and metrology, leading to innovative solutions for complex challenges. In this section, we will delve into the specific applications that characterize coherent optics, emphasizing the benefits and considerations inherent in each area.
Optical Imaging Techniques
Interferometry
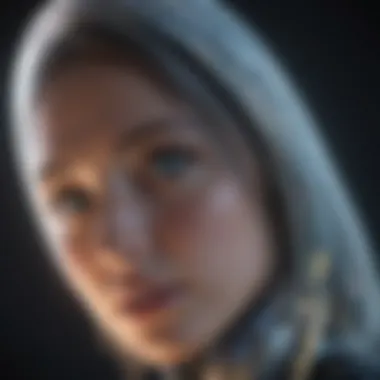
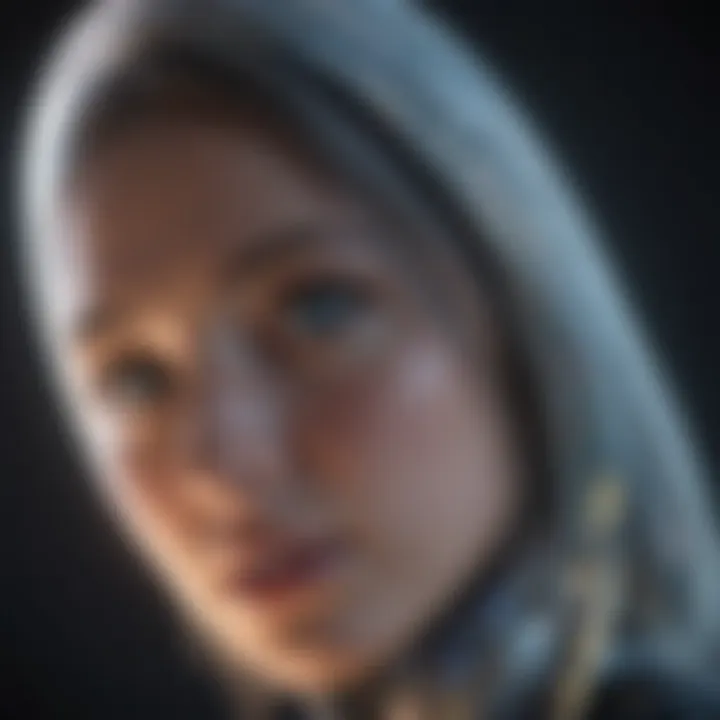
Interferometry is a technique that utilizes the principle of interference between coherent light waves. This method is crucial for measuring the precise dimensions and shape of objects. One key characteristic of interferometry is its high sensitivity, which allows it to detect changes in distance on the order of a fraction of a wavelength of light. Its popularity arises from the ability to achieve detailed imaging without direct contact with the sample, making it a favored choice in fields ranging from astronomy to materials science. The unique feature of interferometry is the ability to create interference patterns, which can reveal a wealth of information about the object under study. However, it can also be sensitive to environmental disturbances, which may impact measurement accuracy.
Holography
Holography represents another significant application within coherent optics. This technique captures the light field from an object and reconstructs it in three dimensions. The key characteristic of holography is its ability to produce a complete three-dimensional image using coherent light. This property makes holography a beneficial choice for many applications, including data storage, security features in documents, and even art. A distinct advantage of holography is that it can provide depth information without the need for complex optical systems; however, its complexity can present challenges in terms of equipment and technique required for effective implementation.
Tomography
Tomography, which utilizes coherent light for imaging internal structures, is another vital application. This technique contributes significantly to medical imaging through methods like optical coherence tomography (OCT). A notable characteristic of tomography is its ability to provide cross-sectional images of biological tissues. This makes it a beneficial method for medical diagnostics and research. The unique aspect of tomography is its reliance on depth resolution, which allows for detailed interior visualization. However, the disadvantages may include the limited penetration depth in certain materials, impacting its broader utility in other contexts.
Telecommunications
Coherent Detection
Coherent detection is a technique used in telecommunications that significantly enhances data transmission efficiency. This method improves signal quality and extends the range of communication systems. The key characteristic of coherent detection is its ability to recover the phase information of the incoming optical signal, enabling better performance compared to incoherent detection. This makes it a popular choice for modern optical networks. The unique feature of coherent detection lies in its implementation of advanced signal processing techniques, which can manage high data rates effectively. Nonetheless, the complexity involved in the required technology may pose challenges for some network providers.
Optical Fiber Systems
Optical fiber systems utilize coherent optics to transmit data over long distances with minimal loss. These systems have revolutionized telecommunications by allowing high bandwidth and low attenuation. The key characteristic of optical fiber systems is their capacity to transmit large amounts of data efficiently. This makes them an essential choice for global communication networks. The unique aspect of these systems is their use of light waves in a fiber medium, significantly mitigating signal degradation over large distances. However, fiber systems can be costly to install and maintain, which may be a consideration for some operators.
Metrology
Precision Measurement
In the realm of metrology, precision measurement techniques utilize coherent optics for highly accurate readings of various physical properties. These methods are pivotal in fields such as engineering and manufacturing. A key characteristic of precision measurement is its remarkable accuracy, often achieving measurements down to nanometers. This accuracy makes it a beneficial application within this field. The unique feature of precision measurement is the use of laser technology and interferometry to ensure high levels of detail and reliability. However, the required calibration and environmental control can limit flexibility during measurements.
Surface Profiling
Surface profiling utilizes coherent optics to analyze the topography of a surface. This application gains importance in quality control processes in manufacturing. The key characteristic of surface profiling is its ability to map surface features and irregularities with high fidelity. It is a beneficial tool for ensuring product quality across various industries. The unique aspect of surface profiling is that it can provide real-time feedback on surface conditions, allowing for immediate adjustments. Nevertheless, the equipment can be expensive, which may restrict its accessibility to smaller firms.
The applications of coherent optics cover a broad spectrum, demonstrating its vital role in technological advancements across multiple fields. Understanding these applications helps to appreciate the broader implications of coherent light manipulation.
Challenges in Coherent Optics
Coherent optics is a field full of promise and innovation. However, it is not without its challenges. Understanding these challenges is crucial for advancing technologies and applications based on this discipline. This section examines two main challenges: environmental sensitivities and technical limitations.
Environmental Sensitivities
Environmental factors play a significant role in coherent optics. Factors such as temperature fluctuations, humidity, and vibrations can greatly affect the performance of optical systems. For example, variations in temperature can change the refractive index of materials used in optical setups. This, in turn, impacts the coherence length of light waves, which is essential for ensuring accurate measurement and imaging.
Moreover, fluctuations in humidity can lead to condensation on optical components. This can cause scattering and loss of signal, interfering with the precision needed in applications like interferometry. Vibrations, whether from machinery or external disturbances, can introduce noise into optical signals, affecting coherence and measurement accuracy.
To mitigate these environmental sensitivities, several strategies can be implemented, including:
- Utilizing temperature-controlled environments for sensitive optical equipment.
- Implementing vibration isolation tables to minimize external impacts.
- Employing protective coatings on optical surfaces to reduce moisture absorption.
Adopting these methods can enhance the reliability of coherent optical systems, ensuring their effectiveness in critical applications like telecommunications and metrology.
Technical Limitations
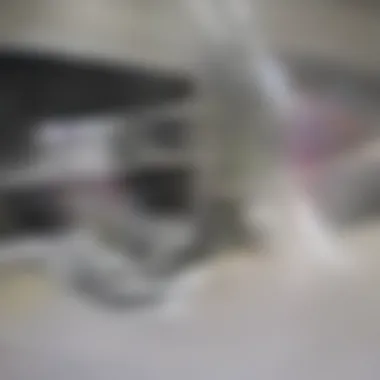
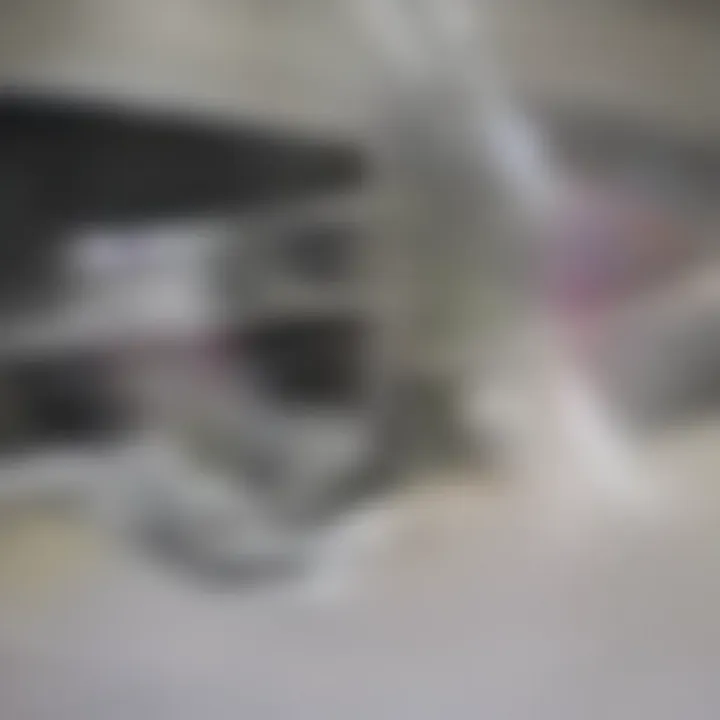
In addition to environmental challenges, coherent optics faces various technical limitations. The design and implementation of coherent optical systems can be constrained by factors such as material properties, system complexity, and cost.
Material limitations are important. Not all materials exhibit the desired optical characteristics needed for coherent light manipulation. For instance, certain crystals used for lasers may have limitations in their efficiency and bandwidth. This can restrict the types of coherent light sources available for specific applications.
Moreover, the complexity of the optical setups can lead to higher chances of error during the design phase. The alignment of optical elements must be precise to maintain coherence; even slight misalignments can result in significant losses in performance.
Cost is also a considerable factor. Advanced coherent optical systems often require expensive components, which can drive up costs and limit accessibility. This is particularly relevant for researchers and institutions operating under budget restrictions.
Future Directions in Coherent Optics
Coherent optics is at an interesting juncture, exploring multiple frontiers in technology. The future directions in this field not only influence current practices but also lay groundwork for breakthroughs in various applications. It is essential to understand these directions to appreciate the potential impact on industries like telecommunications, imaging, and sensing.
Emerging Technologies
Quantum Optics
Quantum optics represents a significant frontier in coherent optics. This discipline explores the quantum nature of light and its interactions with matter. One key characteristic of quantum optics is the ability to manipulate light at the quantum level. Such manipulation allows for powerful new technologies, such as quantum communication and quantum cryptography, making it a popular choice in discussions about future advancements.
The unique feature of quantum optics lies in its exploitation of quantum states, such as entangled photons. This leads to protocols that offer unparalleled security in data transmission. However, there are challenges that accompany these advantages, including the need for sophisticated technology and environments that are often delicate and sensitive.
Integrated Photonics
Integrated photonics is also reshaping the landscape of coherent optics. This area involves integrating photonic devices on a single chip, enabling compact, efficient, and high-performance optical systems. Its key characteristic is miniaturization, which significantly benefits telecommunications and signal processing.
The unique feature of integrated photonics is the scalability and low power consumption it offers. By allowing for smaller devices without compromising performance, integrated photonics pushes the boundaries of what coherent optics can achieve. However, the technology also faces scalability challenges, particularly in manufacturing and design, which must be addressed to realize its full potential.
Improving Coherence in New Applications
As coherent optics advances, improving coherence in new applications becomes paramount. Techniques to enhance coherence could unlock greater precision in measurement and imaging. Research focuses on novel materials and methods to produce coherent light more efficiently. Innovations may include new laser designs or alternative light sources that support coherence over varying distances and conditions.
The future of coherent optics is rife with opportunities. It continues to inspire research, guiding both theoretical and practical endeavors, ultimately pushing forward the boundaries of technology and science.
End and Implications
The conclusion section of the article serves as a critical reflection on the comprehensive exploration of coherent optics. This subject not only encompasses theoretical principles but also has impactful applications in various sectors. The relevance of coherent optics lies in its ability to manipulate and control light with precision, which is essential in advancing technology and scientific inquiry.
Through the detailed analysis provided, it becomes evident that coherent optics plays a pivotal role in fields such as optical imaging, telecommunications, and metrology. By utilizing coherent light sources like lasers, practitioners can achieve unparalleled accuracy and efficiency. The implications of this technology extend beyond basic applications; they shape the future of industries reliant on optical systems.
Moreover, the anticipated advancements in coherent optics promise to influence new technologies such as quantum optics and integrated photonics. This forward-looking perspective emphasizes the continual evolution of this field and its importance for future innovators. Considering these elements, it is clear that coherent optics will remain at the forefront of scientific and engineering disciplines.
"Coherent optics has redefined the possibilities in imaging and detection, driving innovation in multiple sectors and laying a foundation for future discoveries."
Summary of Key Points
The primary discussion points in the article highlight significant aspects of coherent optics:
- Definition and Importance: Coherent optics is based on coherent light sources, enabling precise manipulation of light waves.
- Fundamental Principles: The principles of temporal and spatial coherence are central to understanding the behavior of coherent light.
- Technological Advancements: The development of lasers has transformed fields such as telecommunications, metrology, and optical imaging techniques.
- Applications: Coherent optics finds application in various areas, including interferometry, optical fiber systems, and precision measurement technologies.
- Future Directions: Emerging technologies in quantum optics and integrated photonics signify ongoing innovation in this realm.
These points construct a multifaceted understanding of coherent optics, illustrating its relevance to modern science and technology.
The Role of Coherent Optics in Modern Science
Coherent optics has carved its niche in modern science, primarily due to the unique properties of coherent light. In imaging, it enhances resolution and contrast, allowing scientists to make detailed observations at the microscopic level. In telecommunications, coherent detection improves the efficiency of data transmission over optical fibers, thus supporting the ever-growing demand for bandwidth.
In metrology, coherent optics provides high precision in measurements and surface profiling. The use of coherent light sources enables researchers to develop advanced techniques that were previously unattainable.
Overall, the role of coherent optics in pushing the boundaries of technology and facilitating scientific exploration is undeniable. Its continuous evolution indicates that coherent optics will remain integral to innovation and research across various scientific domains.