Detection Antibody ELISA: A Comprehensive Overview
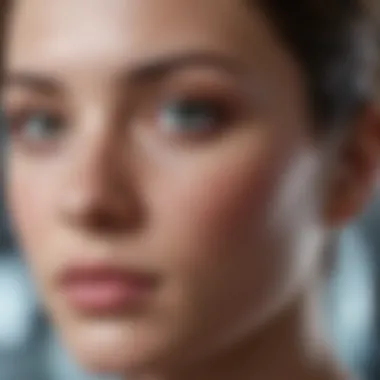
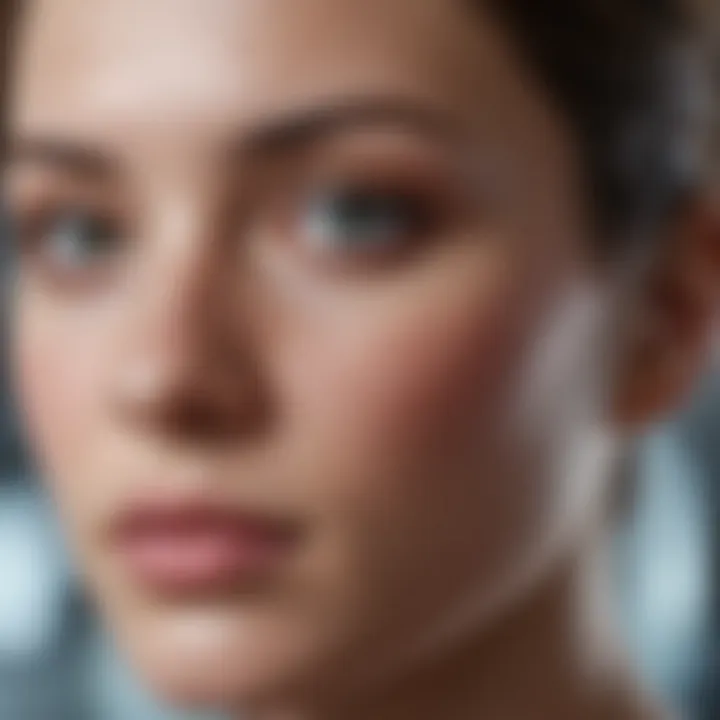
Intro
Detection Antibody Enzyme-Linked Immunosorbent Assay (ELISA) serves as a cornerstone in immunodiagnostics. It allows researchers and clinicians to identify specific antibodies in a sample with high sensitivity and specificity. ELISA's relevance spans multiple fields including biomedical research, pharmaceuticals, and clinical laboratories. Understanding its fundamental principles and applications is crucial for anyone working within these domains.
In this article, we will explore the essence of Detection Antibody ELISA, covering its theoretical framework, methodologies applied in practice, and advancements in the field. By delving deeper into these topics, we aim to provide insights that will help both newcomers and seasoned professionals grasp the intricacies of this assay.
Recent Advances
Latest Discoveries
Recent research has highlighted the enhanced capabilities of ELISA in detecting not just traditional antibodies, but also novel biomarkers. Scientists are continually discovering new antibodies related to diseases, improving the sensitivity of assays and broadening their application. Innovations in sample processing and detection methods have been instrumental in these developments.
In particular, the use of microfluidic technology has gained attention. This allows for smaller sample volumes and rapid analysis, which is critical in time-sensitive situations like infectious disease outbreaks. Additionally, multiplex ELISA platforms have emerged, enabling simultaneous detection of multiple antibodies from a single sample.
Technological Innovations
Technological progress has significantly changed the landscape of ELISA. One such innovation is the integration of nanotechnology. Nanoparticles can enhance the assay's sensitivity, providing clearer results even at low antibody concentrations. This has potential implications in early disease detection, where minute biomarker levels may signal underlying conditions.
Moreover, automated systems are transforming ELISA workflows. These platforms improve efficiency and minimize human error, contributing to more reliable and reproducible data.
"The evolution of ELISA through technological innovation is redefining its capabilities in diagnostics, making it an essential tool in modern science."
Methodology
Research Design
Understanding the research design behind ELISA assays is essential to achieve correct and meaningful results. Typically, ELISA setups are structured using a plate-based system, where samples are added to wells coated with specific antigens. This design enables the measurement of the target antibody response in a controlled environment. Researchers must consider their objectives to select appropriate antigens, controls, and assay formats.
Data Collection Techniques
Accurate data collection is fundamental in any ELISA. This involves the use of spectrophotometry to measure absorbance after adding a substrate. The intensity of the color produced is directly proportional to the concentration of antibodies present. Researchers also employ statistical analyses to validate the results, ensuring robust conclusions.
Prelude to ELISA
The Enzyme-Linked Immunosorbent Assay (ELISA) holds a prominent place in biological research and clinical diagnostics. This section aims to articulate its significance, offering readers an in-depth understanding of its fundamentals, applications, and the essential knowledge needed to utilize this powerful tool effectively.
The importance of ELISA stems from its ability to detect and quantify specific proteins, often antibodies, in various sample types. This capability has monumental implications across medical diagnostics, research studies, and vaccine development. By understanding the mechanisms of ELISA, professionals can accurately interpret results, which leads to better decision-making in clinical and laboratory settings.
Understanding ELISA
ELISA operates on a straightforward principle: the binding of an antigen to an antibody. This binding occurs within a controlled environment, often on a microplate, allowing for efficient analysis of samples. Typically, the methodology involves immobilizing antibodies onto a surface, adding the sample containing the target antigen, and then detecting the antigen-antibody complex using enzyme-linked antibodies that provide a quantifiable signal.
One significant advantage of ELISA is its capacity to test multiple samples simultaneously. This feature enhances throughput, making it a favored choice in high-volume diagnostic labs. Moreover, ELISA is relatively easy to perform, requiring standard laboratory equipment and procedures, which fosters accessibility even in less developed settings.
The sensitivity and specificity of ELISA can be customized based on the antibodies and substrates used, leading to varied applications across different fields. Research has shown that with proper optimization, ELISA can achieve unparalleled consistency and reliability in detecting specific antibodies.
Historical Context
The development of ELISA is rooted in decades of immunological research. The concept can be traced back to the 1970s, when two scientists, Dr. Eva Engvall and Dr. Peter Perlmann, introduced the assay. Their pioneering work on the enzyme-linked immunosorbent assay transformed the landscape of laboratory diagnostics.
Initially, ELISA was limited in scope, primarily employed in research settings. However, it gradually gained traction in clinical diagnostics due to advancements in antibodies and detection techniques.
Since then, ELISA has become an essential tool, widely used in various applications, from detecting infectious diseases to monitoring therapeutic antibodies in treatment regimens. The historical evolution of ELISA reflects ongoing improvements in technology and methodology, demonstrating its adaptability to emerging scientific challenges.
In summary, the introduction to ELISA offers a crucial foundation for understanding its principles, historical evolution, and its extensive applications. The ability to quantify specific immune responses in various contexts underscores the importance of this assay in contemporary science.
Fundamentals of Antibody Detection
Understanding the fundamentals of antibody detection is essential for effectively utilizing techniques like ELISA. These basics provide the foundation for a variety of applications in both clinical diagnostics and research. Knowledge about antibodies helps in comprehending how they function in different immunological responses. Furthermore, it allows for a better grasp of how ELISA can be employed to detect specific antibodies, thus making it invaluable for accurate testing.
What are Antibodies?
Antibodies, also known as immunoglobulins, are proteins produced by the immune system. They play a crucial role in identifying and neutralizing foreign objects such as bacteria and viruses. These Y-shaped molecules bind to antigens, leading to their destruction or inactivation. There are five main classes of antibodies: IgA, IgD, IgE, IgG, and IgM. Each class has distinct functions and properties, contributing to the immune system's complexity.
Antibodies are integral to diagnostic techniques. They enable the identification of diseases by detecting specific antigens that are present when an infection occurs. In ELISA, antibodies are key components that capture the target antigen, facilitating subsequent detection steps.
Role of Antibodies in Immunology
Antibodies serve multiple roles within the broader scope of immunology. They are not only pivotal in neutralizing pathogens but also in modulating immune responses. The binding of antibodies to antigens forms complexes that signal immune cells to engage with and clear the invaders.
Importantly, antibodies contribute to the specificity of immune responses. They can distinguish between similar structures, allowing the immune system to target specific threats. Additionally, the presence of memory B cells enables the body to respond more quickly upon re-exposure to the same antigen, underscoring the importance of antibodies in long-term immunity.
"The specificity and adaptability of antibodies mark them as fundamental players in immunology and highlight their significance in detection techniques like ELISA."
Principle of ELISA
The principle underlying the ELISA technology is of paramount significance. It elucidates how the detection antibody works and the various stages involved in an ELISA assay. Understanding this principle lays the groundwork for comprehending subsequent methodologies. The unique ability of ELISA to detect specific antibodies efficiently transforms how diseases and conditions are diagnosed in clinical and research settings. Consistency and precision are essential. Knowing how these factors function helps produce reliable results.
The ELISA Mechanism
The ELISA mechanism operates through a series of well-defined steps. First, the target antigen is immobilized onto a microplate. This is usually done by passive adsorption to the plate surface. After that, the specific antibodies directed against the antigen are introduced. If these antibodies bind to the antigen, the complex is formed. A secondary enzyme-linked antibody is then added. This antibody can bind to the primary antibody, facilitating the next stage.
Following these steps, a substrate for the enzyme is added. The enzyme linked to the secondary antibody catalyzes a reaction that produces a measurable signal, often a color change. The intensity of this signal correlates to the amount of antigen initially present in the sample. Therefore, quantifying this color change gives precise results regarding antibody concentration. This sensitivity is one of ELISA's main benefits.
Types of ELISA
ELISA can be categorized into several types based on how the assay is performed. Each type serves distinct purposes and presents unique characteristics. Here we discuss four main types:
Direct ELISA
Direct ELISA simplifies the process by minimizing the steps. The specific antigen is bound directly to the microplate, and a labeled primary antibody is added. This approach provides a rapid result due to fewer steps. The main advantage is the time saved in the assay procedure. However, one limitation can be reduced sensitivity compared to other methods. Still, for many applications, its straightforwardness is quite beneficial.
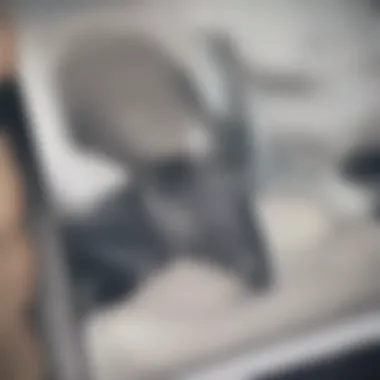
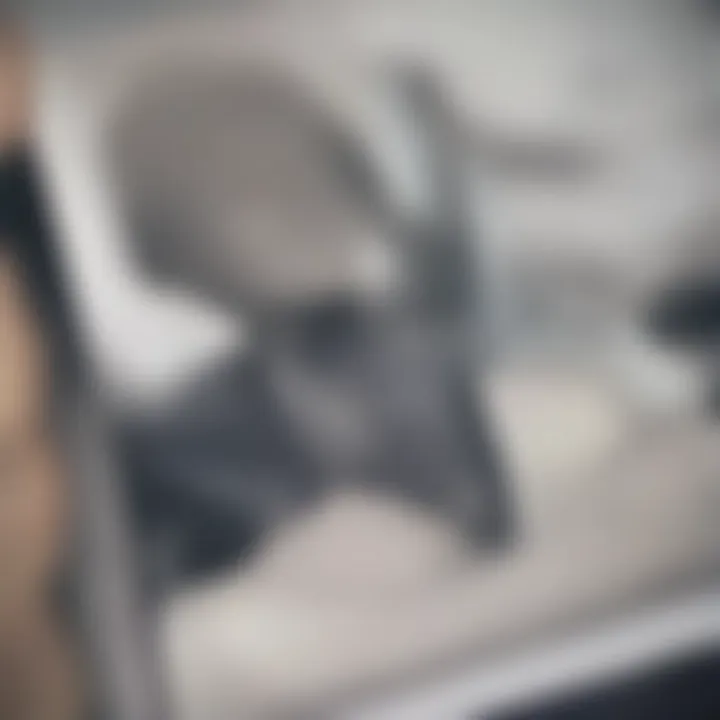
Indirect ELISA
Indirect ELISA incorporates an additional step, increasing the sensitivity of the test. After binding the antigen, a primary antibody attaches to it, followed by a labeled secondary antibody that recognizes the primary one. This layered approach means that even low antibody concentrations can produce a notable signal. It is popular for its sensitivity. However, there can be challenges related to non-specific binding, leading to potentially misleading results in some cases.
Sandwich ELISA
Sandwich ELISA is notable for its high specificity and sensitivity. In this method, the microplate is coated with a capture antibody that binds to the target antigen. After the antigen is introduced, a second detection antibody binds to the antigen, forming a "sandwich." This configuration allows detection of very low levels of the antigen. Its main advantage is this ability to accurately quantify antigens. However, good quality antibodies are essential for optimal results.
Competitive ELISA
Competitive ELISA works differently. Here, the antigen competes with a labeled version for binding sites on a specific antibody. This method is advantageous for detecting small amounts of antigen. A disadvantage is the complexity involved in interpreting results, as higher concentrations of the target will correlate to lower signal production. This can make quantification more challenging. Nevertheless, it is a valuable method in specific contexts.
Understanding these different ELISA types enhances comprehension of their application in various fields. Each has its unique benefits and challenges, driving their relevance depending on the intended use.
Components of ELISA
In the context of the Detection Antibody ELISA, understanding the components of the assay is fundamental. The quality and properties of each component directly influence the assay's reliability and sensitivity. This section will identify the core elements of ELISA, emphasizing microplate selection, antibody coating, and substrates and enzymes.
Microplate Selection
The selection of the microplate is critical in ELISA. Microplates come in different materials and surface treatments, which can affect protein binding capacity and the assay's final outcomes. Common materials include polystyrene and polypropylene. Polystyrene plates are typically used due to their ability to adsorb proteins effectively, making them suitable for many assays.
When choosing a microplate, factors such as binding capacity, background signal, and cost effectiveness should be considered. High-binding plates enhance the signal, while low-binding plates can lead to diminished sensitivity. It is also important to ensure compatibility with the detection methods and reagents utilized in the assay.
Antibody Coating
Antibody coating is a pivotal process in ELISA. The primary antibodies need to be attached to the microplate surface to allow for the specific capture of target antigens present in the sample. The efficiency of this step can heavily influence the assay's sensitivity.
Factors to consider for antibody coating include:
- Concentration: Optimal antibody concentration should be determined through preliminary experiments.
- Coating Buffer: The choice of buffer, such as phosphate-buffered saline (PBS), can affect antibody orientation and activity.
- Incubation Time and Temperature: Proper conditions enhance binding efficiency. Longer times and room temperature can often yield better results.
A well-executed coating process allows for consistent and reproducible results across assays.
Substrates and Enzymes
The last key component of ELISA is the substrates and enzymes. Enzymes conjugated to the detection antibodies catalyze a reaction with the substrate to produce a measurable signal, usually observable through colorimetric or fluorescent changes.
Commonly used enzymes include horseradish peroxidase and alkaline phosphatase. The choice of substrate must align with the enzyme used. For instance, tetramethylbenzidine (TMB) is often used with horseradish peroxidase, generating a chromogenic product that forms a colored solution.
Considerations for choosing substrates and enzymes include:
- Sensitivity: Some substrates result in higher sensitivity than others.
- Stability: The shelf-life and stabilization of reagents are also essential for consistent results.
- Detection Method: Ensure that the selected substrates produce signals compatible with the detection mechanisms employed.
Methodology of the Detection Antibody ELISA
The methodology of the Detection Antibody ELISA is crucial for ensuring reliable and reproducible results. This section provides a clear understanding of the sample preparation process and the assay procedure. Each step in the ELISA methodology must be carefully executed to minimize errors and ensure the integrity of the results. By focusing on these elements, professionals can efficiently employ ELISA in various applications, including research and diagnostics.
Sample Preparation
Sample preparation is a fundamental step in the ELISA process. It involves collecting and processing the samples to remove any substances that could interfere with the assay results. Proper sample collection and handling techniques are vital. Contaminants can lead to false positives or negatives, making accurate preparation essential.
The following points should be considered during sample preparation:
- Collection Method: Different source samples may require distinct collection methods. Blood serum, plasma, or tissue extracts have unique handling needs.
- Storage Conditions: Samples should be stored under appropriate conditions to maintain their stability. For example, serum samples are often stored at -20°C or lower for prolonged periods.
- Dilution: Samples may need to be diluted to ensure that they fall within the assay's detection range. This requires careful calculation based on the expected concentration of the antibodies being measured.
Overall, proper sample preparation enhances assay accuracy and boosts the reliability of the findings.
Assay Procedure
The assay procedure outlines the step-by-step process of conducting the ELISA. Each component of the procedure plays a vital role in determining the outcome of the test. Here’s a breakdown of the primary steps:
- Coating the Plate: The wells of a microplate are coated with the specific antibodies to capture the antigens. This step is crucial, as it establishes the basis of the assay.
- Blocking: After coating, a blocking solution is added to prevent nonspecific binding. This process reduces background noise, enhancing assay sensitivity.
- Sample Addition: Prepared samples are introduced into the wells. During this step, specific antibodies present in the samples bind to the coated antigens.
- Detection Antibody: A detection antibody, often linked to an enzyme, is added. This antibody binds to the antigen and allows for signal amplification upon substrate addition.
- Substrate Addition: Enzymatic reaction takes place when a substrate is introduced, producing a measurable signal, often in the form of color change. The intensity of the color correlates with the amount of antigen present in the sample.
- Measurement: Finally, the optical density is measured, usually using a spectrophotometer. This quantitative data will ultimately be analyzed to determine the presence or concentration of the antibodies.
The meticulous execution of the assay procedure ensures reliable results, critical for clinical diagnostics and research.
Attention to detail at each stage of the assay procedure is what separates successful ELISAs from less reliable ones. Small variations can lead to significant differences in outcomes, underscoring the necessity for precision.
Data Analysis in ELISA
Data analysis is crucial in the context of Detection Antibody ELISA. It serves as the backbone for interpreting results accurately and deriving meaningful conclusions from experiments. As ELISA is widely used in various fields, the reliability of the data obtained must be established. Effective data analysis ensures that the results reflect true biological or chemical interactions. Moreover, it allows researchers to distinguish significant findings from noise in the data, leading to trustworthy outcomes.
In this section, we will explore how to interpret results in ELISA and the common analytical techniques used. The focus will be on enhancing the understanding of key methodologies that improve the accuracy and validity of ELISA results.
Interpreting Results
When analyzing ELISA results, clear interpretation is essential. This process involves assessing the optical density (OD) values obtained from each well of the microplate. The OD values are proportional to the amount of antibody detected in the sample. In general, the higher the OD value, the greater the concentration of antibodies.
A critical step is determining whether these values fall within expected ranges. Results can be compared against known standards or controls included in the assay. This comparison reveals if the sample's antibody levels are above or below certain thresholds relevant to the study, thereby supporting diagnostics or research findings.
Quality control measures, such as including blank wells and positive controls, ensure that data is reliable. Documentation of all steps taken enhances reproducibility, a key aspect in scientific inquiry.
Common Analytical Techniques
Different analytical techniques can support the interpretation of ELISA results. Understanding these can significantly enhance the robustness of ELISA as a detection method.
Standard Curve
A standard curve provides a reference for quantifying the concentration of antibodies in unknown samples. It is created by plotting the OD values of a series of known concentrations against their respective values. This graphical representation aids in determining a linear relationship between concentration and OD values.
Key Characteristic: The key characteristic of a standard curve is its ability to allow precise quantification of sample concentrations based on the intensity of the signal measured.
Benefits: This method is relevant as it enables researchers to assess concentrations accurately. Using a standard curve can differentiate between variations due to experimental errors and actual biological differences.
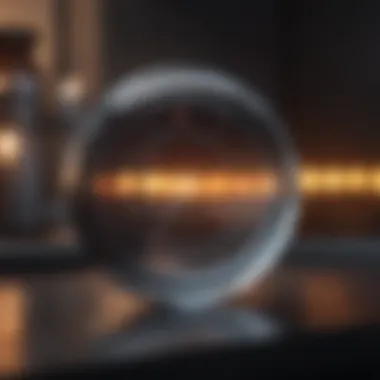
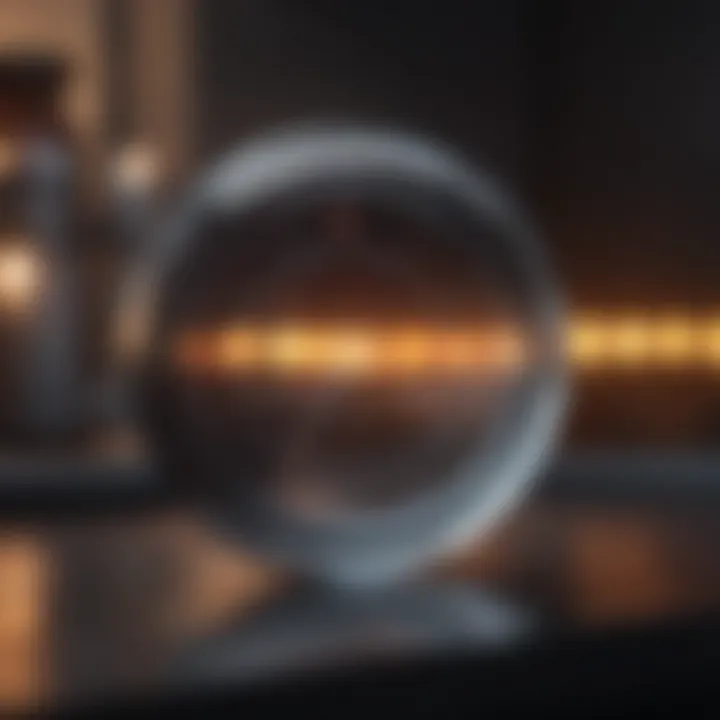
Advantages/Disadvantages: While a standard curve is beneficial for quantification, its accuracy depends heavily on the consistency of the conditions and controls used when establishing it. A poorly plotted standard curve can lead to misinterpretation of sample concentrations.
Limit of Detection
The limit of detection (LOD) is another important concept in ELISA data analysis. It indicates the lowest concentration of the target analyte that can be reliably detected but not necessarily quantified. Understanding LOD is vital for determining the assay’s sensitivity.
Key Characteristic: A defining characteristic of LOD is its focus on the detection capability of the assay rather than how much can be quantified.
Benefits: Knowing the LOD helps scientists understand the assay's limitations and efficiency in various contexts. It informs decisions like whether an assay can detect antibodies at concentrations relevant for clinical or research purposes.
Advantages/Disadvantages: While a low LOD is generally advantageous, extreme sensitivity can sometimes lead to false positives. Therefore, it is crucial to balance sensitivity with specificity when evaluating the ELISA performance.
Data analysis in ELISA ultimately forms the bridge between empirical results and actionable insights. Properly conducted analysis fosters reliability, ensuring that subsequent interpretations lead to scientific advancement.
Applications of ELISA
The versatility of the Enzyme-Linked Immunosorbent Assay (ELISA) extends its reach into various biological fields, making it an invaluable tool in both research and clinical diagnostics. This section explores the significant applications of ELISA, highlighting its use in clinical diagnostics, research applications, and veterinary medicine. Each application offers different benefits and highlights the assay's adaptability in different environments.
Clinical Diagnostics
In the clinical setting, ELISA plays a critical role in disease detection and monitoring. The method's ability to detect specific antibodies is essential for diagnosing infectious diseases, autoimmune disorders, and allergy testing. The high sensitivity and specificity of ELISA allow for accurate diagnosis, which can be crucial for timely treatment.
Key advantages of using ELISA in clinical diagnostics include:
- Rapid Results: ELISA tests can provide results in a relatively short time compared to other methods, facilitating faster clinical decisions.
- High Throughput: The ability to test many samples at once makes ELISA ideal for large-scale screening.
- Cost-Effective: Compared to other methodologies, ELISA is often more affordable, making it accessible for many laboratories.
Considerations must be made regarding reagent quality and assay optimization to minimize error rates and ensure reproducibility. Proper quality controls are essential to validate the results.
Research Applications
In research, ELISA serves as a powerful tool for understanding immune responses and disease mechanisms. Scientists use ELISA to quantify proteins, cytokines, and biomarkers, which helps to elucidate the relationship between immune responses and various conditions.
Some notable research focuses include:
- Vaccine Development: ELISA is instrumental in evaluating the efficacy of vaccine candidates by measuring antibody responses.
- Cancer Research: Investigators employ ELISA to discover biomarkers that could help in early cancer detection and therapeutic monitoring.
- Studies on Infectious Diseases: Researchers utilize ELISA to track immune responses to pathogens, assist in epidemiological studies, and vaccine trials.
The flexibility and adaptability of ELISA procedures mean that they can be tailored to meet specific research needs, thus providing accurate and reliable data.
Veterinary Medicine
ELISA is not limited to human health; it is equally significant in veterinary medicine. The assay is employed for diagnosing diseases in animals, monitoring vaccine responses, and conducting epidemiological studies on zoonotic infections.
Key applications in veterinary medicine include:
- Infectious Disease Diagnosis: ELISA aids in detecting diseases like heartworm or feline leukemia, enabling better management and treatment of affected animals.
- Serology: The assay is widely used in testing serum samples for various antibodies, supporting herd health assessments.
- Vaccine Efficacy: By measuring antibody levels post-vaccination, veterinarians can evaluate the effectiveness of vaccines in different species.
ELISA's reliable performance in veterinary applications strengthens its role in safeguarding animal health and enhancing preventive care strategies.
Challenges and Limitations
Understanding the challenges and limitations of ELISA is crucial for any researcher or clinician utilizing this method. While Detection Antibody ELISA is a powerful tool, it is not without issues. Various factors can impact the accuracy and reliability of results. Recognizing these challenges can help in refining approaches and achieving more consistent outcomes.
Cross-Reactivity Issues
Cross-reactivity poses a significant challenge in ELISA practices. This phenomenon occurs when an antibody intended for one specific antigen interacts with another, non-specific antigen. These unintended interactions can lead to false-positive results. Cross-reactivity can undermine the reliability of assays that detect specific antibodies.
To mitigate this issue, careful design of assays is essential. Here are some strategies to address cross-reactivity:
- Use highly purified antigens to minimize off-target interactions.
- Prioritize monoclonal antibodies over polyclonal whenever feasible, due to their specificity.
- Validate assays with negative control samples to assess potential cross-reactivity.
Understanding the nature of antibodies and their possible interactions is fundamental in avoiding misinterpretations that arise from cross-reactivity.
Variability in Results
Variability in results is another critical consideration in ELISA methodology. This variability can stem from multiple sources, including sample handling, reagent quality, equipment calibration, and even the operator's skill level. Such inconsistencies can lead to discrepancies in the data generated.
Some common factors affecting variability include:
- Inconsistent sample preparation protocols, which can alter the concentration of the analytes.
- Variations in the enzyme activity or substrate quality, leading to different signals.
- Differences in incubation times and temperatures that can influence the binding interactions.
To achieve reliable results, implementing stringent quality control measures is vital. Monitoring the performance of assays regularly helps identify trends in variability.
"Quality control is not just a good practice; it is essential for the integrity of the data obtained from ELISA assays."
Given the potential for variability, establishing standard operating procedures and training the personnel involved in conducting ELISA remains fundamental. By addressing these challenges, researchers can enhance the robustness and reliability of ELISA results.
Quality Control in ELISA
Quality control plays a vital role in the reliability and accuracy of ELISA results. It ensures that the assay operates within predefined performance limits. This becomes increasingly important in clinical and research settings, where the consequences of erroneous results can be significant. Establishing comprehensive quality control measures contributes to reproducibility and confidence in findings, thus bolstering the overall integrity of research studies and diagnostic applications.
The elements of quality control in ELISA typically encompass standardization procedures, validation techniques, and routine monitoring. These components work together to create a robust framework that minimizes variability and enhances the precision of the assay outcomes. Quality control not only facilitates effective troubleshooting when issues arise but also aids in maintaining compliance with regulatory standards.
Key considerations in implementing quality control in ELISA include:
- Defining acceptable performance criteria for assays
- Regular calibration of instruments
- Utilization of control samples in each assay run
- Monitoring of reagent stability and storage conditions
- Training and competency assessments for personnel involved in testing
By prioritizing these aspects, laboratories can significantly improve their assay reliability, reduce costs associated with re-testing, and ensure data integrity.
Standardization Procedures
Standardization procedures are crucial for achieving consistent and reliable ELISA results. These procedures involve the establishment of protocols that define how each assay should be performed. This includes aspects such as sample preparation, incubation times, and washing steps. Standardization helps reduce variability and allows for meaningful comparisons across different experiments or institutions.
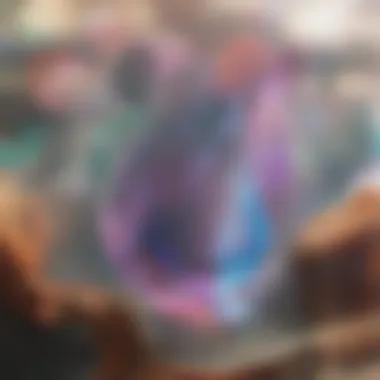
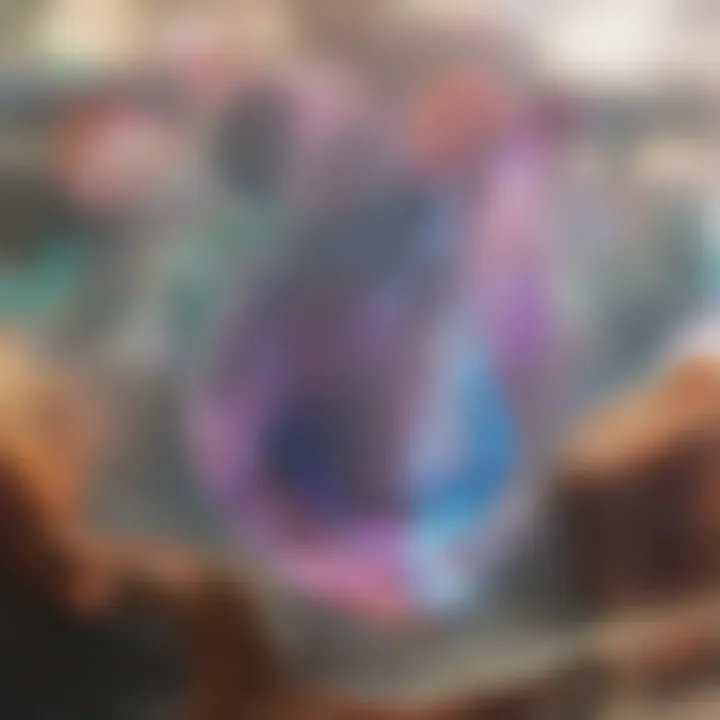
To achieve standardization, several strategies can be employed:
- Development of standardized reagents
- Implementation of optimized assay protocols
- Regular review of testing procedures
- Utilization of certified reference materials
- Maintenance of stringent environmental controls during assays
By adhering to these practices, researchers can enhance reproducibility, which is paramount for scientific advancements.
Validation Techniques
Validation techniques are essential for confirming that an ELISA assay performs as intended. Validation involves assessing various parameters such as sensitivity, specificity, accuracy, and precision. Through validation, laboratories can verify that their assays provide reliable and interpretable results, further fortifying the foundation of quality control.
Common validation techniques include:
- Assessing the limit of detection
- Performing recovery studies
- Evaluating cross-reactivity with closely related substances
- Conducting inter- and intra-assay variability assessments
Proper validation is not a one-time task; it requires regular reassessment as reagents or protocols change over time, ensuring ongoing reliability of the ELISA and fostering trust among the end-users of the data.
Recent Advances in ELISA Technology
The field of Detection Antibody ELISA has seen significant advances in technology that improve its efficiency and precision. These innovations enhance the assay's capability to detect specific antibodies while also broadening its application scope. As we dive into recent developments, it is essential to understand how they transform traditional approaches and contribute to more accurate, reliable outcomes.
Microfluidics in ELISA
Microfluidics is one of the most exciting advancements in ELISA technology. It involves the manipulation of fluids at a microliter scale, allowing for miniaturization of the ELISA process. This technology significantly reduces reagent consumption and samples needed for analysis.
Microfluidic devices can effectively perform multiple assays simultaneously. This capability accelerates the diagnostics process. Moreover, it drastically decreases the time to results. Using fewer reagents also lowers costs significantly.
Some benefits of incorporating microfluidics into ELISA include:
- Higher Sensitivity: The miniaturization of reactions enhances the signal-to-noise ratio, leading to more accurate results.
- Reduced Sample Volume: Small volumes of samples are easier to acquire from patients, which is particularly beneficial in clinical settings.
- Automation Potential: Microfluidics can be easily integrated with automated systems. This smoothens processes and increases throughput in laboratories.
In summary, microfluidics has the potential to revolutionize ELISA, making it a quicker, more cost-effective option for antibody detection.
Automation in ELISA
Automation in ELISA technology offers a transformative approach to laboratory procedures. Incorporating automated systems into the workflow significantly improves accuracy and reproducibility. Automation streamlines several repetitive and time-consuming steps, reducing human error and enhancing efficiency.
With automated platforms, laboratories can process a higher number of samples within a limited time frame, which is increasingly important in high-demand settings such as clinical diagnostics.
Key advancements in automation include:
- Robotic Pipetting Systems: These systems accurately dispense reagents and samples, ensuring consistency across various runs.
- Integrated Analyzers: These devices can perform multiple analyses concurrently, simplifying the workflow and speeding up the testing process.
- Data Management Systems: Automated systems often come equipped with advanced data tracking, which minimizes the risk of data loss and facilitates easier analysis.
Automation not only enhances the speed of results but also ensures data integrity, one of the critical factors in research and clinical applications.
Future Directions
In the realm of Detection Antibody ELISA, the future directions emphasize the need for continuous improvement and adaptation. Innovations in this field can vastly enhance the accuracy and efficiency of assays. With the growing demands of research and clinical diagnostics, techniques must evolve to keep pace. Future directions encompass a variety of elements including technological advancements, streamlined methodologies, and integrations that serve to refine the function and broaden the applicability of ELISA.
Innovations in Detection Methods
The journey towards improved detection methods in ELISA is marked by groundbreaking developments. One significant trend is the use of nano-materials and biosensors which make assays more sensitive. This could lead to earlier diagnosis and treatment of diseases. Recent advances in multiplexing capabilities allow for the testing of multiple antibodies simultaneously, enhancing throughput and reducing costs. Furthermore, the integration of digital platforms for real-time data analysis improves accuracy and reduces human error. Adoption of artificial intelligence in data interpretation is another noteworthy innovation, giving insights into complex datasets that would be challenging for researchers to analyze manually.
Some essential points to consider are:
- Increased sensitivity and specificity of tests
- The ability to conduct parallel assays for multiple targets
- Reduced time and resource expenditure in testing
"Future detection methods can be pivotal in transforming how we approach diagnostics, making them more effective and efficient."
Integration with Other Technologies
The future of Detection Antibody ELISA also lies in the seamless integration with other technologies. Combining ELISA with techniques like microfluidics and lab-on-a-chip technologies, for instance, can revolutionize sample handling and result accuracy. This integration allows for smaller sample volumes and faster processing times. Another promising direction is the incorporation of next-generation sequencing, enabling deeper insights into antibody diversity and response.
Considering the integration of technologies brings several benefits:
- Improved efficiency through automation
- Enhanced data quality and reliability
- Broader applications across various fields, especially in personalized medicine
In summary, forward-looking perspectives in Detection Antibody ELISA are vital. They offer clear paths toward enhancing the capabilities and applications of this critical assay. The attention to innovations and integrations is key to overcoming current limitations and adapting to future challenges.
The End
The conclusion encapsulates the essential takeaways regarding Detection Antibody ELISA. It is vital as it reflects on the comprehensive nature of the examination that has been provided throughout the article. Understanding the conclusion helps solidify the knowledge acquired from previous sections and serves as a point of reflection for its significance in various fields.
ELISA has proven to be a reliable technique in detecting specific antibodies, which is crucial for diagnostics and research. This section seeks to balance the theoretical insights with practical applications, enhancing the reader’s understanding of how ELISA can be utilized effectively.
Summary of Key Insights
The article has highlighted several core insights regarding Detection Antibody ELISA:
- Broad Applicability: ELISA is used in clinical diagnostics, veterinary medicine, and research applications.
- Methodological Framework: Understanding the steps from sample preparation to data analysis is crucial for optimizing the use of ELISA.
- Challenges and Solutions: Awareness of potential issues, such as cross-reactivity and variability, allows for the development of better validation techniques.
By synthesizing these points, readers gain a well-rounded understanding of both the capabilities and limitations of ELISA as a detection method.
The Significance of ELISA in Contemporary Science
ELISA holds a pivotal role in modern scientific investigations. Its ability to measure the presence of antibodies is invaluable in various domains, from medical diagnostics to pharmaceutical development.
The following elements underline its importance:
- Facilitating Disease Diagnosis: It allows for early detection of pathogens and monitoring immune responses, crucial for timely diagnosis of diseases.
- Research Validation: ELISA serves as a reliable standard in experimental research to validate findings, contributing to advancing scientific knowledge.
- Technological Advancements: Ongoing developments in ELISA technology, including microfluidics and automation, are enhancing accuracy, decreasing costs, and improving accessibility.
In light of these factors, Mobility of ELISA in contemporary science demonstrates how foundational it is as a tool, enabling breakthroughs that address various health and research-related challenges.
Benefits of including a well-structured reference section:
- Increased Credibility: Demonstrates thorough research and understanding of the topic, instilling confidence in the readers.
- Resource Accessibility: Allows readers to access foundational and advanced literature for further study.
- Intellectual Honesty: Acknowledges the contributions of other researchers and their work.
In addition, attention should be given to the format and presentation of citations. Consistent citation style not only enhances readability but also reflects professionalism. Whether using APA, MLA, or another format, uniformity facilitates the reader’s navigation of the references.
"Without proper references, any scientific work lacks the necessary foundation for credibility."