Exploring Direct DNA Sequencing: Techniques and Implications
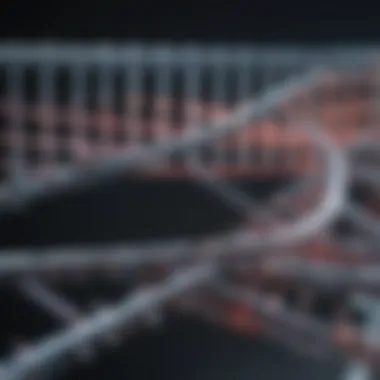
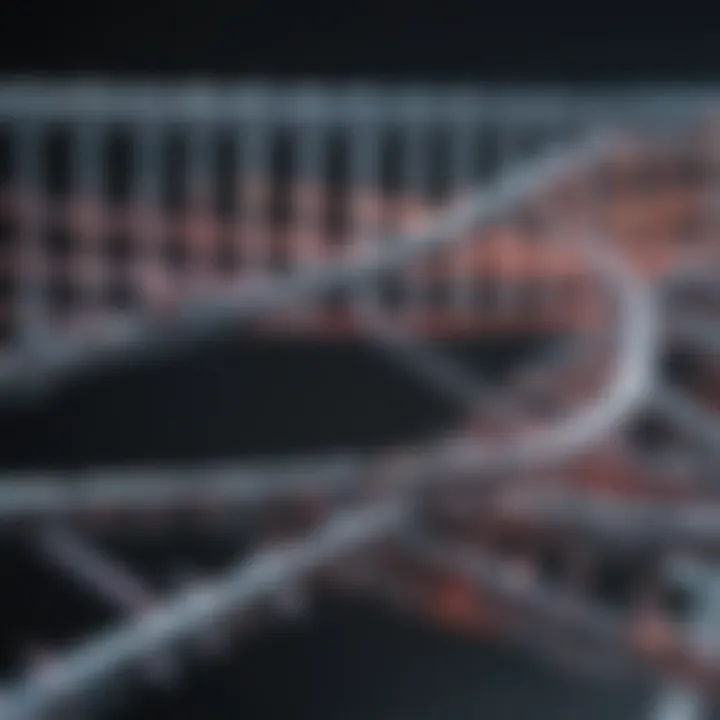
Intro
Direct DNA sequencing has reshaped the field of genetics. It enables scientists to determine the precise order of nucleotides in deoxyribonucleic acid (DNA). Understanding this sequence is essential, as it lays the foundation for many aspects of biology and medicine. This article offers an in-depth look at the various techniques employed in direct DNA sequencing, as well as their implications across scientific and clinical practices.
Recent Advances
Recent developments in direct DNA sequencing technology have been remarkable, initiating significant changes in the field and broadening its applications.
Latest Discoveries
Several recent discoveries highlight the utility of direct DNA sequencing. Notable among these is the ability to sequence long reads, which allows for the comprehensive mapping of complex genomic regions. Studies involving PacBio and Oxford Nanopore Technologies have yielded promising results that shed light on difficult-to-sequence areas of the genome. Researchers can now explore structural variations and epigenetic modifications with more precision. Such advancements augment our understanding and provide critical insights into genetic disorders and evolutionary biology.
Technological Innovations
The term 'direct DNA sequencing' refers not only to methodologies but also to the instruments driving these practices. Innovations such as improved sequencing throughput and reduced costs have proliferated. The emergence of portable sequencing devices has made it possible to conduct analyses in diverse environments, thus bridging the gap between laboratory and fieldwork. Lastly, developments in bioinformatics have enhanced the capability to analyze and interpret vast amounts of sequencing data efficiently.
Methodology
Understanding the methodology behind direct DNA sequencing is crucial for appreciating its implications. This section details the research design employed and the data collection techniques utilized in current studies.
Research Design
Research in this domain often employs both experimental and observational designs. Sequencing projects may focus on specific populations or conditions, targeting particular genetic regions associated with diseases. Collaborative efforts across institutions also contribute to large-scale genomic studies that yield significant insights.
Data Collection Techniques
Data collection for direct DNA sequencing typically involves:
- Sample acquisition from biological sources, such as blood or tissue.
- DNA extraction using methods like phenol-chloroform or column-based protocols.
- Amplification through polymerase chain reaction (PCR) to ensure sufficient substrate for sequencing.
- Sequencing reactions that utilize various platforms to achieve accurate reads.
- Post-sequencing analysis employing software tools to align sequences and identify variations.
The integration of high-throughput sequencing technologies has revolutionized genetics, enabling researchers to explore genomes at an unprecedented scale.
Through careful methodology and innovative technologies, direct DNA sequencing continues to advance our capacity for genetic analysis, pushing the frontiers of research and clinical applications. By understanding these frameworks, one can appreciate the vital role direct DNA sequencing plays in modern science.
Foreword to Direct DNA Sequencing
Direct DNA sequencing serves as a cornerstone in molecular biology and genetics. This technique allows researchers to determine the precise nucleotide sequence of a given DNA strand. As scientific inquiry increasingly demands higher accuracy in genetic information, direct DNA sequencing emerges as an essential tool. The implications are vast, affecting fields from personalized medicine to genetic engineering. This article will investigate the methodologies that underpin direct DNA sequencing, noting their efficiencies and potential areas for improvement.
Understanding the history and evolution of these techniques provides essential context for their current applications.
Defining Direct DNA Sequencing
Direct DNA sequencing can be defined as the process of determining the order of nucleotides in a DNA molecule. This involves converting the chemical information contained in the DNA into a readable format, often through the use of specialized machines known as sequencers. Unlike other sequencing methods, direct DNA sequencing offers higher precision and accuracy. Sequencers read the DNA directly without the need for extensive prior manipulation. The output is usually represented in a series of base pairs, which researchers can analyze further for various applications, including detecting mutations, understanding gene functions, and evolving therapeutic strategies.
Historical Context and Evolution
The journey of DNA sequencing began in the early 1970s with the development of Sanger sequencing. Freddrick Sanger introduced a method that utilized dideoxynucleotides, allowing scientists to read sequences of few hundred nucleotides. This was revolutionary at that time. As research advanced, so did the need for faster and more efficient sequencing techniques. The late 2000s saw the advent of next-generation sequencing, which allowed for the sequencing of millions of fragments in parallel. This technology changed the landscape of genomic research, providing vast amounts of data in a fraction of the time previously required.
More recently, third-generation sequencing technologies, such as those developed by Pacific Biosciences and Oxford Nanopore Technologies, have enabled the sequencing of longer DNA fragments. This development allows researchers to gain more comprehensive genomic insights. The evolution from manual methods to high-throughput, automated procedures exemplifies the pace of innovation in this field. Each breakthrough not only enhances our ability to sequence DNA but also vastly broadens its applications in various scientific domains.
Methodologies in DNA Sequencing
Understanding the methodologies in DNA sequencing is crucial for grasping how sequences are determined and analyzed. These methodologies offer insights into genetic structures and functions, which are essential in fields such as medicine, agriculture, and environmental science. Each method has unique strengths and limitations, impacting accuracy, speed, and cost. Researchers must choose methods that align with their specific objectives.
Sanger Sequencing: The First Generation
Sanger sequencing, developed by Frederick Sanger in 1977, is often referred to as first-generation sequencing. It was one of the earliest methods to reliably determine DNA sequences and has been widely used for many years. The process involves the selective incorporation of chain-terminating dideoxynucleotides during DNA replication. When a dideoxynucleotide is added, the elongation of the DNA strand stops, resulting in fragments of varying lengths.
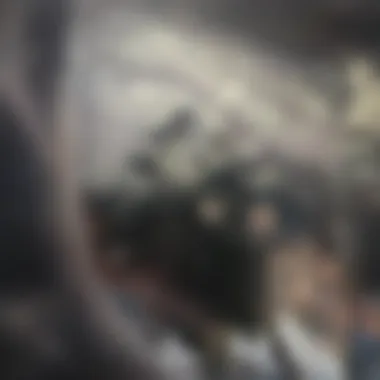
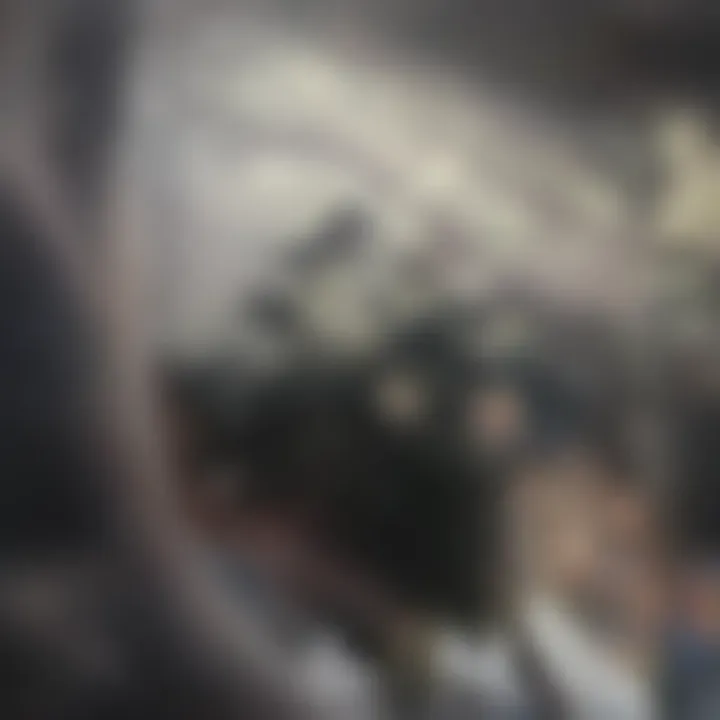
This technique is particularly notable for its high accuracy, which is beneficial for sequencing smaller genomes or targeted areas. It is often used in clinical diagnostics, for instance, when identifying mutations in specific genes. However, Sanger sequencing can be time-consuming and less suited for large-scale sequencing projects due to its relatively low throughput.
Next-Generation Sequencing (NGS)
Next-Generation Sequencing (NGS) represents a significant advancement over Sanger sequencing, allowing for the sequencing of entire genomes more rapidly and cost-effectively. NGS encompasses various techniques, including Illumina sequencing, Ion Torrent, and others, each utilizing different methods for fragmenting and processing DNA.
NGS can produce millions of sequences in a single run, drastically increasing output. This methodology allows researchers to conduct large-scale genomics studies, identify rare variants, and understand complex biological processes with unprecedented depth. However, NGS also introduces challenges related to data management and analysis, given the volume of information generated.
NGS has revolutionized genomic studies, paving the way for personalized medicine and comprehensive biological research.
Third-Generation Sequencing Techniques
Third-generation sequencing (TGS) incorporates various technologies that offer even greater improvements in sequencing speed and accuracy. Techniques like PacBio and Oxford Nanopore technologies exemplify TGS, enabling the direct sequencing of single DNA molecules. This approach eliminates the need for PCR amplification, which can introduce bias and errors.
TGS provides longer read lengths compared to NGS, which is advantageous for resolving complex genomic regions and structural variants. This capability is particularly useful in understanding repetitive regions in the genome or phasing variants accurately. However, the error rates in some TGS methods can be higher, which necessitates careful validation of sequences generated.
Principles of Direct DNA Sequencing
Understanding the principles of direct DNA sequencing is essential for appreciating the broader implications and applications of this technology. The underpinning mechanisms not only define how sequences are obtained but also frame the context in which results are interpreted. Knowledge in this area bridges the gap between theory and practical application, enabling researchers to make informed decisions on methodology and analysis.
DNA Fragmentation and Amplification
DNA fragmentation is a crucial step in preparing DNA for sequencing. It involves breaking down long strands of DNA into smaller, more manageable pieces. By doing this, the sequencing process becomes more efficient and accurate. Fragments typically range from hundreds to thousands of base pairs, depending on the sequencing method chosen. Several techniques can facilitate fragmentation, including enzymatic digestion and mechanical shearing.
Amplification, often following fragmentation, enhances the quantity of DNA available for sequencing. Techniques such as Polymerase Chain Reaction (PCR) are employed to create numerous copies of a specific DNA segment. This process ensures that there is enough material to generate robust sequencing data. Amplification requires careful optimization, as excess amplification can lead to errors in subsequent analysis. The balance between fragmentation and amplification is vital to achieve high fidelity in sequencing results.
Base Calling and Data Interpretation
Base calling is the process of determining the specific nucleotide sequence from raw sequencing data. This step translates the signals detected during sequencing into actual DNA bases - adenine, cytosine, guanine, and thymine. Accuracy in base calling directly impacts the quality of the results. Various algorithms and software tools aid in this task, making it a sophisticated and sometimes complex process.
After base calling, data interpretation follows, where researchers must analyze the sequence data to derive meaningful biological insights. This interpretation often involves comparing results to reference genomes or databases. It may also include identifying genetic variants that have implications in various fields, such as medicine and evolutionary biology. The reliability of the data interpretation hinges on the quality of both the fragmentation/amplification techniques and the base calling methods utilized, highlighting the interconnectedness within the sequencing workflow.
"The accuracy of the sequencing process is paramount, as errors in base calling can lead to misinterpretation of genetic data."
Overall, understanding these principles is essential for students, researchers, and professionals engaged in genetic research or related fields. With the rapid advancements in DNA sequencing technologies, a solid grasp of these foundational aspects enhances the ability to navigate the complexities of genomic analysis.
Technological Innovations
The landscape of DNA sequencing is undergoing rapid transformation, thanks largely to recent technological innovations. These advancements are pivotal, as they enhance the capabilities of researchers and clinicians in genomic analysis. This section delves into crucial elements driving these changes: advancements in sequencer technology and the growing role of bioinformatics in data analysis.
Advancements in Sequencer Technology
New sequencer technologies have significantly improved the efficiency and accuracy of DNA sequencing. For instance, newer models like the Illumina NovaSeq series or Oxford Nanopore's MinION utilize innovative methodologies to handle larger datasets swiftly. These machines can sequence whole genomes in days instead of months, a crucial factor for research and clinical diagnostics.
Some of the key features of current sequencer technologies include:
- Increased throughput: Modern sequencers can process hundreds of samples simultaneously, which is essential for large-scale genomic studies.
- Reduction in costs: The price of sequencing continues to decline, making it more accessible for various applications from clinical settings to academic research.
- Real-time sequencing: Certain technologies allow researchers to obtain data in real-time, which enables immediate analyses and quicker decision-making.
The combination of these advancements enables scientists to tackle complex genetic questions with increased precision and speed. The advancements not only facilitate a deeper understanding of genetics but also promote personalized medicine, where treatments can be tailored based on an individual’s unique genetic profile.
Bioinformatics: The Role of Software in Analysis
With the surge in data generated from sequencing technologies, bioinformatics has become vital. This discipline employs software and algorithms to analyze and interpret massive amounts of genetic data. The complexity of data derived from sequencing requires sophisticated methods for its interpretation, leading to more insightful conclusions. In addition to organizing raw data, bioinformatics helps in tasks such as:
- Alignment of sequences: Matching DNA sequences to reference genomes to identify variations.
- Variant calling: Detecting genomic variations that may have implications for health or disease.
- Statistical analysis: Applying methodologies to validate findings and ensure their reliability.
- Visualization tools: Presenting data in an understandable format that assists in interpretation and decision-making.
As sequencing technologies continue to evolve, the integration of bioinformatics will be essential in ensuring that the generated data contributes meaningfully to scientific knowledge and clinical applications.
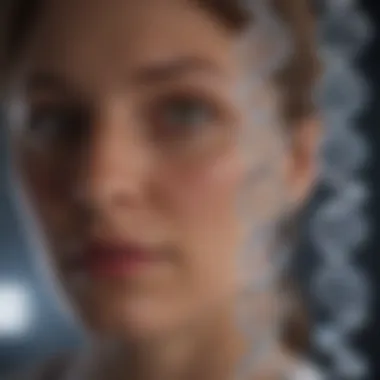
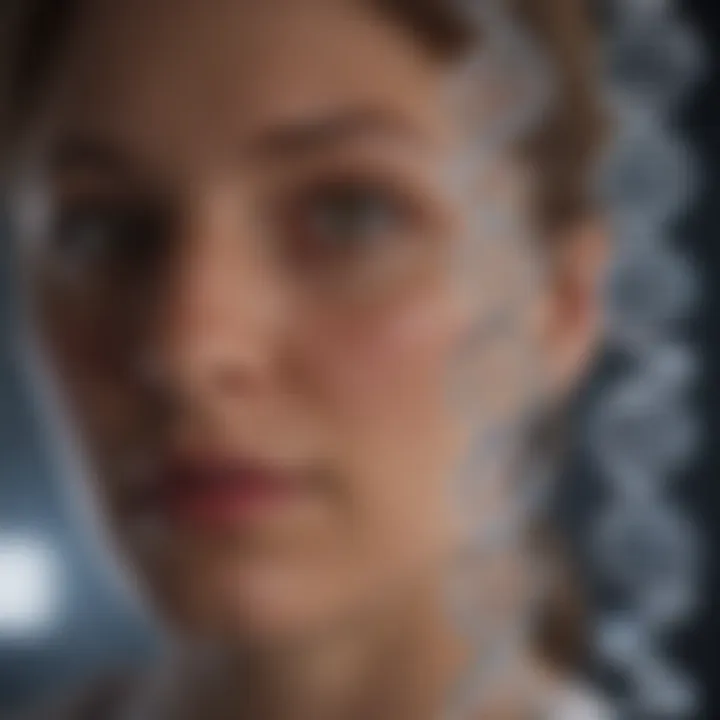
"The collaboration between sequencing technology and bioinformatics is what will drive the next wave of genomic discoveries."
Technological innovations in sequencer devices and bioinformatics tools indicate a promising future for direct DNA sequencing. The evolution of these technologies is not merely about enhancing efficiency but also about expanding the horizons of what can be explored in the realms of genetics.
Applications of Direct DNA Sequencing
Direct DNA sequencing plays a crucial role in numerous fields, providing insights and advancements that significantly enhance our understanding and capabilities. The importance of this topic lies in its ability to yield precise genetic information that can be applied across various domains, including healthcare, agriculture, and biodiversity studies. With the advent of new methodologies and technologies, the applications of direct DNA sequencing have expanded, leading to innovative solutions that address both existing challenges and upcoming needs.
Clinical Diagnostics and Personalized Medicine
In the clinical sector, direct DNA sequencing has transformed diagnostic processes. By analyzing genomic information, healthcare professionals can identify genetic disorders and predispositions to diseases much earlier than traditional methods allowed. This precision is vital for tailoring specific treatment regimens based on individual genetic profiles, a concept widely known as personalized medicine.
- Benefits of Clinical Applications:
- Enhanced Diagnostic Accuracy: Early detection of genetic conditions allows for timely intervention.
- Tailored Treatment Plans: Treatments can be designed to suit genetic variations among patients, leading to better outcomes.
- Prognostic Insights: Genetic sequencing enables healthcare providers to give patients informed predictions about disease progression.
Personalized medicine is becoming a standard approach, particularly in oncology, where cancer treatments can be optimized based on the genetic makeup of tumors.
Agricultural Biotechnology
In agriculture, direct DNA sequencing unlocks potential for genetic improvements in crops and livestock. By identifying favorable traits, such as disease resistance or improved yield, scientists can apply this information to breeding programs more effectively.
- Key Applications in Agriculture:
- Genomic Selection: Farmers can utilize genomic data to select superior plants and animals, thereby enhancing productivity.
- Trait Mapping: Identifying specific genes responsible for desirable traits helps in the development of enhanced breeds.
- Sustainable Practices: Genetic modifications that promote resilience to climate change can contribute to sustainable agricultural practices.
These advancements support food security by ensuring that agricultural practices can adapt to climate variability and pest pressures, promoting a more stable food supply.
Evolutionary and Environmental Studies
The utility of direct DNA sequencing also extends into evolutionary biology and environmental science. Scientists can analyze genetic data to uncover relationships among species and understand evolutionary adaptations over time. This understanding is crucial in conservation efforts as well.
- Applications in Environmental Science:
- Biodiversity Monitoring: Sequencing helps track genetic diversity within populations, which is essential for conservation strategies.
- Phylogenetic Studies: Understanding evolutionary relationships through genetic data helps elucidate species evolution and migration patterns.
- Ecological Impact Assessments: Genomic data can provide insights into the impact of environmental changes on different species.
As environmental challenges intensify, genetic insights from direct sequencing can assist in developing strategies for biodiversity conservation and management, ensuring ecological health.
"The future of research and application in DNA sequencing holds immense promise, particularly as techniques continue to evolve."
Overall, the application of direct DNA sequencing is multifaceted, with implications that resonate through medicine, agriculture, and ecology. Such advancements not only enhance theoretical understanding but also provide practical solutions to real-world problems.
Challenges in Direct DNA Sequencing
Understanding the barriers within direct DNA sequencing is crucial for advancing this technique. Despite the advancements in methodology and technology, several challenges remain that can significantly impact the effectiveness of sequencing projects. These challenges not only hinder scientific progress but also affect the broader implementation of sequencing technologies in clinical and research settings.
Accuracy and Error Rates
Accuracy in DNA sequencing is paramount. Even a single mistake in the nucleotide sequence can lead to incorrect conclusions in genetic studies, impacting everything from basic research to clinical diagnostics. Direct DNA sequencing methods, such as those based on polymerase chain reaction (PCR), are not immune to errors.
Errors can arise due to a variety of factors, including low-quality samples, contamination, and limitations of the sequencing technology itself. For instance, some third-generation sequencing technologies may have increased error rates compared to traditional methods like Sanger sequencing. This variability in accuracy is a fundamental concern, especially when the results are applied in personalized medicine or genetic counseling.
To enhance accuracy, various techniques can be employed:
- Consensus Sequencing: This approach involves sequencing the same DNA fragment multiple times to generate a consensus sequence, thus reducing the impact of random errors.
- Quality Control Measures: Implementing robust protocols for sample collection and handling can diminish contamination risks.
- Advanced Algorithms: Utilizing sophisticated bioinformatics tools for data analysis can help in identifying and correcting errors in the sequencing data.
Ultimately, addressing the accuracy and error rates in direct DNA sequencing is essential for the reliability of genetic analyses.
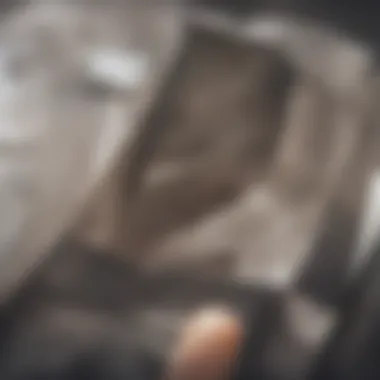
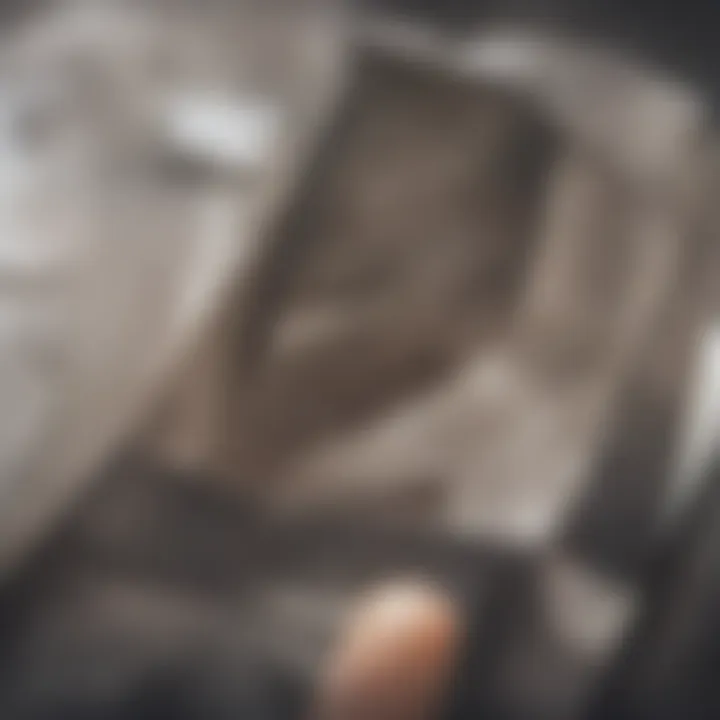
Cost Effectiveness and Accessibility
The cost of DNA sequencing has gradually decreased, yet it remains a notable challenge for broader accessibility. High-quality sequencing technologies, particularly those used in clinical applications, can still be prohibitively expensive. This poses significant barriers for many laboratories, especially in low-resource settings.
Several factors contribute to the overall cost of direct DNA sequencing, such as:
- Equipment: High-throughput sequencers require significant investment.
- Operational Costs: Maintaining the sequencers, along with associated supplies and skilled personnel, adds to the financial burden.
- Data Management: The analysis and storage of the large volumes of data generated necessitates advanced bioinformatics tools, which may also involve high costs.
Increasing accessibility to direct DNA sequencing will likely require:
- Innovative Funding Models: Establishing grants and subsidies could aid institutions with fewer resources.
- Collaboration Networks: Encouraging collaborations across institutions can facilitate shared access to sequencing technologies.
- Public Awareness Initiatives: Educating stakeholders about the importance of sequencing could lead to increased investment in technology.
Future Perspectives
The future of direct DNA sequencing holds immense potential as advancements in technology continue to emerge. This section explores significant elements that may influence the trajectory of DNA sequencing, showcasing both benefits and considerations.
Emerging Technologies and Trends
In recent years, several innovative technologies have surfaced that promise to reshape the landscape of direct DNA sequencing. These advancements not only enhance the accuracy and speed of sequencing but also reduce costs, making the process more accessible to a wider range of researchers and clinicians. For instance, developments in nanopore sequencing allow for real-time analysis of long DNA sequences. This method enables scientists to understand complex genomic structures more efficiently.
Additionally, advancements in artificial intelligence (AI) are playing a crucial role in analyzing sequencing data. Machine learning algorithms can interpret vast amounts of genetic data quickly, providing insights that were previously elusive. This integration of AI with direct DNA sequencing can significantly improve the detection of genetic disorders and tailor treatments based on individual genetic profiles.
Other noteworthy trends include the push towards portable sequencing devices. These small-scale systems are being developed to facilitate fieldwork, making it possible to conduct genetic investigations in remote locations. Such accessibility could revolutionize studies in environmental biology, forensic science, and more, as researchers no longer rely exclusively on laboratory-based sequencing.
- Nanopore Sequencing: Enables real-time analysis of long sequences.
- AI Integration: Improves data interpretation and insights.
- Portable Devices: Enhances accessibility for field research.
This refreshing wave of technologies indicates that direct DNA sequencing will continue to evolve, promising greater efficiency and wider applications.
Ethical Considerations in DNA Sequencing
While the advancements in direct DNA sequencing are impressive, they also raise important ethical concerns that demand careful consideration. As technology improves, the implications of sequencing personal and genetic data become more significant. Privacy issues stand as a primary concern. With the increased capacity to sequence DNA, safeguarding individual genetic information must be a priority.
Moreover, there is the question of data ownership. Who owns the sequences generated from a person's DNA? This question is critical, especially in clinical settings where genetic data can influence health decisions. Regulations surrounding the use, storage, and sharing of DNA data must evolve in conjunction with technological progress to protect patients and researchers alike.
Additionally, the risk of discrimination based on genetic information merits attention. As genomic data becomes more available, there may be potential misuses by insurers, employers, and other entities. Legal frameworks must adapt to preclude such misuse and ensure that genetic information is treated with the utmost respect.
"With the rapid strides in DNA sequencing, we must also stride towards ensuring that ethical standards keep pace."
Therefore, while exploring the future of direct DNA sequencing, it is paramount to maintain a balance between innovation and ethics, ensuring that the continued evolution of this field respects individual rights and societal norms.
Ending
In the realm of genetics, the significance of direct DNA sequencing cannot be overstated. It serves as a cornerstone for understanding genetic structures and functions, providing critical insights essential for research and clinical applications. The exploration of various sequencing methodologies reveals how they uniquely contribute to advancements in the field, enhancing both accuracy and efficiency.
The methods outlined in this article underscore the evolution from traditional approaches to cutting-edge technologies. This progression illustrates not only technical improvements but also a shift in our understanding of genomics in various applications. The benefits of these developments include enhanced diagnostic capabilities, personalized medical treatments, and valuable insights in evolutionary research.
Furthermore, as we navigate through challenges related to accuracy and accessibility, it becomes clear that the future of DNA sequencing is intertwined with ongoing innovation. This includes addressing cost-related barriers that can limit access to new technologies, particularly in clinical settings.
"The challenge lies not just in sequencing, but in interpreting and utilizing that data effectively to impact health and science positively."
As we look ahead, the implications of direct DNA sequencing are immense, not just for laboratory settings but also for everyday applications in healthcare and environmental studies. The focus on ethical considerations will play a critical role as these technologies become more integrated into society.
Summarizing Key Insights
The discussion within this article encapsulates several key insights regarding direct DNA sequencing:
- Technological Evolution: From Sanger sequencing to advanced NGS and third-generation methods, each progression has narrowed down inaccuracies and expanded the scope of genomic exploration.
- Applications Beyond Research: Direct DNA sequencing greatly influences fields such as personalized medicine, agricultural biotechnology, and evolutionary science.
- Challenges in Accuracy and Cost: Despite advances, issues remain regarding the error rates and costs associated with sequencing techniques, necessitating ongoing research and development.
- Ethical Implications: As genomic data becomes widely accessible, ethical considerations surrounding privacy, consent, and the use of genetic information must be addressed.
The Future of Genomic Analysis
The trajectory of genomic analysis is rapidly evolving, with several emerging trends shaping the landscape of direct DNA sequencing:
- Integration of AI in Bioinformatics: Artificial intelligence will increasingly play a role in data analysis, ensuring that the vast datasets from sequencing are interpreted accurately and efficiently.
- Accessibility Improvement: Innovations focused on reducing costs and improving the usability of sequencing technologies are paramount. This democratization will enhance its availability to underserved regions and populations.
- Personalized Medicine Paradigm: The ongoing development of customized healthcare plans based on individuals’ genetic profiles will change how we approach medical treatment and preventative care.
- Research Expansion: As sequencing becomes less resource-intensive, the potential for a greater understanding of the genetic basis of diseases and traits increases significantly.