Exploring the Depths of Genetics and Its Implications
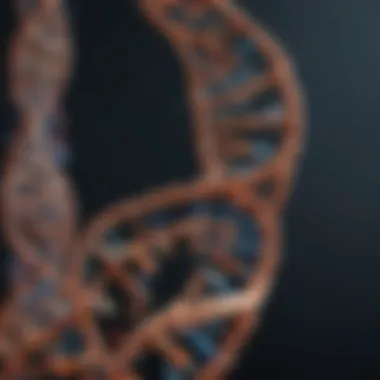
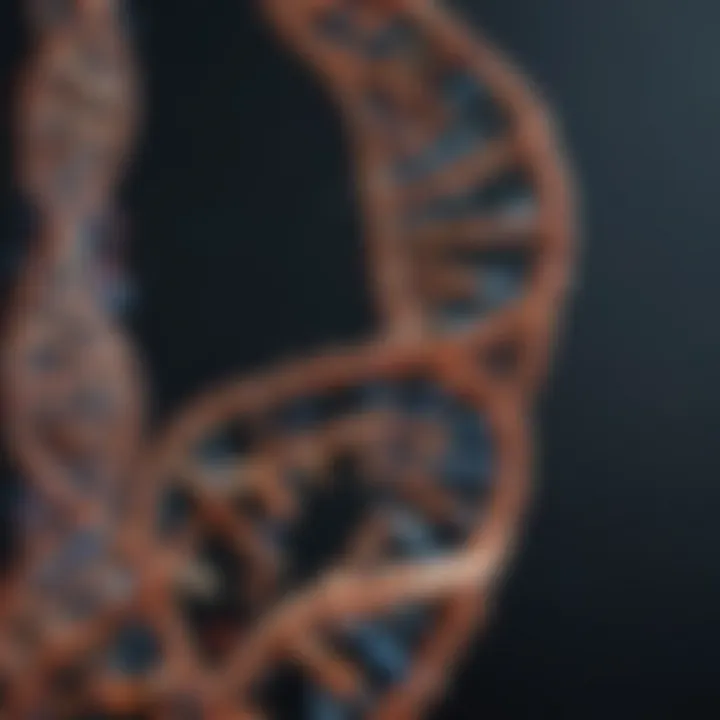
Intro
Genetics serves as the backbone of biological sciences, offering transformative insights into not just how life exists but also how it interacts and evolves. When we peel back the layers, we find a tapestry woven from strands of DNA, the basic unit of heredity, a component that holds the secrets of both our makeup and our vulnerabilities. Through generations, these secrets have informed everything from agricultural practices to medical breakthroughs, shaping human understanding of life itself.
As we stand on the brink of new discoveries, this exploration into genetics aims to shed light on several pressing questions. What drives the inheritance of traits? How have technological advances revolutionized our approach toward genetic research? Furthermore, how do these insights intersect with ethical considerations regarding genetic engineering and behavioral influences?
In the coming sections, our investigation will delve into both historical milestones and recent advances in the field, revealing the complex relationship between genetic science and its implications for society. This narrative is not merely theoretical; it invites everyone from students to seasoned professionals to understand how genetics plays a vital role in disciplines encompassing healthcare, environmental science, and even psychology.
With that groundwork laid, we can transition seamlessly into our first major topic.
Prolusion to Genetics
Genetics is a topic that sits at the very core of biological sciences. It dives deep into how traits are passed down from one generation to the next, helping us unlock the mysteries behind heredity. Understanding genetics is no longer a niche concern limited to scientists or biologists; it impacts everyone, from farmers who want to grow hardier crops, to medical professionals looking for tailored therapies for their patients.
This section introduces readers to the fundamental concepts and significance of genetics. It paints a picture of the intricate mechanisms that dictate not just what we look like, but also who we are on the inside—our predispositions to certain diseases, behaviors, and even some aspects of our personality.
Definition of Genetics
Genetics is the science that studies genes, genetic variation, and heredity in organisms. To put it simply, it is all about understanding how traits are transmitted through generations. This can encompass everything from blood type to complex mental traits. The term itself derives from the Greek word "genesis," meaning "origin" or "birth," which illustrates the link to life's building blocks.
History of Genetic Research
The path of genetic research is lined with groundbreaking discoveries and pioneering figures who have molded our understanding of biology. The tale of genetics is richly layered, full of triumphs and twists.
Key Figures in Genetics
Many standout individuals have played pivotal roles in shaping the field of genetics. Perhaps the most famous is Gregor Mendel, the Austria-born monk whose experiments with pea plants laid the groundwork for what we now refer to as Mendelian inheritance. Mendel’s meticulous work might have taken place in obscurity, but today it holds monumental significance for anyone diving into genetic studies.
Another notable figure is James Watson, alongside Francis Crick, who co-discovered the double helix structure of DNA. Their discovery in 1953 changed the course of biological sciences, laying the foundation for modern genetics and molecular biology. What’s fascinating is how these key figures often struggled during their lifetimes to have their work fully appreciated, yet their contributions ultimately spurred monumental advancements in various scientific fields.
Milestones in Genetic Discoveries
Milestones in genetic research signify important breakthroughs. For instance, the discovery of DNA's double helix structure was a milestone that led to further advancements, such as the Human Genome Project in the late 20th century. This ambitious project managed to map the entire human genome, opening doors we never knew existed before.
Moreover, the development of CRISPR technology has revolutionized gene editing, leading to limitless possibilities in medical treatment and biotechnology. Such discoveries highlight the dynamic nature of genetics, revealing how advances often build upon previous knowledge. Each milestone not only enhances academic understanding but also presents real-world applications that can impact health, agriculture, and industry.
Overall, recognizing these key figures and milestones is crucial in tracing the evolution of genetics. These insights set the stage for exploring the more complex aspects of genetic material and inheritance mechanisms that follow.
The Fundamentals of Genetic Material
Grasping the fundamentals of genetic material is vital in any exploration of genetics. This domain serves as a cornerstone upon which our understanding of inheritance, variation, and molecular biology is built. The structure and nature of genetic material provide insights into how traits are passed on from one generation to the next, and understanding this lays the groundwork for delving into more complex concepts related to genetic research and applications.
Structure of DNA
Double Helix Model
The double helix model of DNA is often hailed as a masterpiece of biological architecture. This structure, which looks like a twisted ladder, was discovered by James Watson and Francis Crick in the 1950s. The brilliance of this model is that it not only explains how genetic information is stored but also provides a mechanism for how it can be replicated.
One key characteristic of the double helix is its stability, thanks to the complementary base pairing between adenine with thymine and guanine with cytosine. This property is immensely beneficial for maintaining the integrity of genetic information through generations. Each turn of the helix carries vital code, protected by the robust backbone formed from sugar and phosphate groups.
However, the unique aspect of this model is its ability to unwind during replication. This unwinding allows enzymes to access the genetic code, making it possible to create copies — a process essential for cell division. While the model has practical advantages, the complexity of unwinding and rewinding means that any error can lead to mutations, which can, in turn, contribute to diseases or genetic disorders.
Components of Nucleotides
Understanding the components of nucleotides is crucial to comprehending how DNA functions. Each nucleotide, essentially the building block of DNA, comprises three key elements: a nitrogenous base, a sugar molecule, and a phosphate group. This triple configuration allows nucleotides to link together in chains, forming the larger structure of DNA.
The sugar and phosphate form the backbone of the DNA strand, ensuring stability. The nitrogenous bases, however, hold the genetic instructions. The uniqueness of nucleotides stems from their varying nitrogenous bases—adenine, thymine, guanine, and cytosine—which determine the specific traits and functions within an organism.
The significance of nucleotides is not limited to their role in DNA. They also play essential roles in cellular energy processes, such as ATP, which is fundamental for activities including muscle contraction and metabolic reactions. The disadvantage, though, lies in the potential for errors during DNA synthesis, which can lead to mutations affecting how cells function.
Genes and Chromosomes
Definition of Genes
A gene can be understood as a functional unit within DNA, responsible for the traits passed from parent to offspring. These segments of DNA are not static; they can be expressed or silenced depending on numerous factors, including environmental influences. The key characteristic of genes is their coding capacity. They contain instructions to build proteins, which are crucial for the structure and function of living organisms.
One of the advantages of genes is their ability to adapt and evolve over generations, allowing species to survive in changing environments. Their dynamic nature is a popular focal point in genetic studies, especially in exploring genetic diversity and its implications for evolution. However, the complexity of gene interactions often introduces confounding variables, complicating the understanding of specific traits.
Chromosome Organization
Chromosomes are fundamentally structures that organize and package DNA. They appear as tightly wound coils during cell division, ensuring that genetic material is accurately distributed to daughter cells. The key feature of chromosome organization is the presence of histones, proteins that help condense the DNA into a usable form. This structure aids in efficient gene expression and regulation, crucial for correct cellular function.
The advantages of chromosome organization extend to facilitating repair mechanisms for damaged DNA, promoting genetic stability. Chromosome abnormalities, however, can lead to disorders such as Down syndrome and Turner syndrome, underscoring the importance of proper organization for healthy development.
"The structure of genetic material is not just a detail; it is the framework upon which life builds its narrative."
In summary, understanding the fundamentals of genetic material — from the double helix and its nucleotide components to genes and chromosomal organization — provides a solid grounding for further exploration of genetic concepts like inheritance and variation. Each element plays a critical role, emphasizing the intricate interplay of biological molecules that drive the essence of life.
Mechanisms of Inheritance
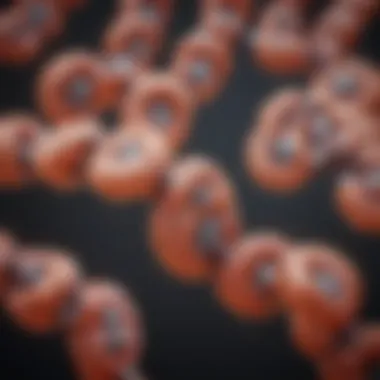
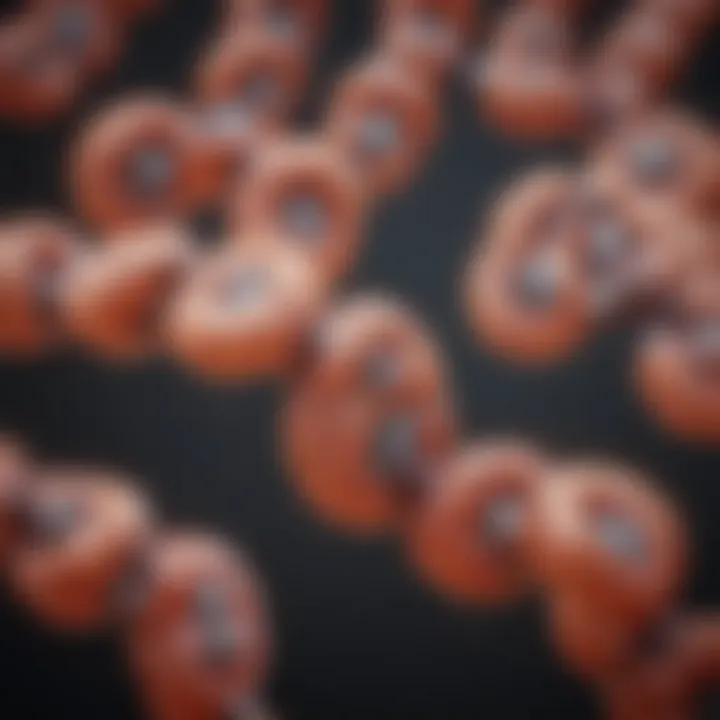
The study of inheritance mechanisms forms a cornerstone in the understanding of genetics. It provides insight into how traits are passed from parents to offspring, helping both academic and practical applications of genetic knowledge. Looking closely at these mechanisms allows for exploring not only the classic and widely accepted Mendelian principles but also the nuanced complexities of Non-Mendelian inheritance. This section will delineate these mechanisms and elucidate their relevance in genetics.
Mendelian Inheritance
Mendelian inheritance serves as the bedrock of genetics. Grounded by Gregor Mendel’s groundbreaking experiments with pea plants, this concept introduced us to the foundation upon which modern genetics was built. A pair of fundamental principles underpin this inheritance pattern: the Principles of Segregation and the Principles of Independent Assortment.
Principles of Segregation
The Principles of Segregation articulate how alleles segregate from one another during gamete formation. In simpler terms, an individual carries two alleles for each gene, one from each parent, and these alleles segregate when reproductive cells (gametes) are formed. This principle highlights that offspring inherit a random combination of alleles from their parents, hence explaining the variation seen in traits.
One key characteristic of segregation principles is that it sheds light on how dominant and recessive alleles interact. When a dominant allele is present, it will mask the recessive one, leading to observable traits that stem primarily from dominant alleles. This principle is beneficial because it lays the groundwork for predicting inheritance patterns, leading to better understanding in fields like agriculture and breeding.
The unique aspect of the Principles of Segregation is its straightforward nature, allowing for use of Punnett squares for calculations of probabilities of offspring traits. However, it can oversimplify the complexity of real-world genetic expressions, especially when multiple genes are involved in a trait's manifestation.
Principles of Independent Assortment
The Principles of Independent Assortment build on Mendel's initial discoveries, proposing that the alleles of different genes assort independently from one another during gamete formation. This principle advises that the inheritance of one trait generally does not affect the inheritance of another, creating a rich tapestry of genetic possibilities.
The key attribute that makes independent assortment appealing is its demonstration of genetic diversity. This principle underpins the generation of unique combinations of traits, which can have evolutionary advantages, such as enhancing survival through assorted phenotypes that respond differently to environmental changes.
However, while independent assortment is fundamental, its relevance can be diminished in certain scenarios, such as when genes are located closely together on the same chromosome, a phenomenon known as genetic linkage, which complicates assumption of independence.
Non-Mendelian Inheritance
In contrast to Mendelian inheritance, Non-Mendelian inheritance provides a broader perspective on how traits can be inherited. This category includes mechanisms such as incomplete dominance, codominance, and polygenic inheritance. Each of these forms expands on Mendel’s ideas, showcasing the complexities and additional layers of genetic interactions.
Incomplete Dominance
Incomplete Dominance occurs when the phenotype of heterozygotes is intermediate between the phenotypes of individuals homozygous for either trait. Effectively, it's like blending colors; for example, crossing a red flower with a white flower could produce pink flowers. This principle adds a depth to Mendelian genetics by illustrating that not all alleles are strictly dominant or recessive.
This form of inheritance is significant for its unique blending feature, allowing for a range of expressions that can affect genetic diversity. However, while this adds interest to genetic variety, it may complicate predictions of offspring traits, as the outcomes are not always clear-cut.
Codominance
Codominance takes a different approach; it represents a condition in which both alleles contribute equally and separately to the phenotype. For instance, in a situation involving blood types, the presence of A and B alleles results in a person with AB blood type. These traits don’t blend; both are manifested fully in the phenotype.
The distinctive characteristic of codominance distinguishes it from neither complete dominance nor incomplete dominance, making it a useful reference point in learning about inheritance. Its primary advantage lies in the clarity it offers in expressing heterozygous conditions. Conversely, it can also introduce complexity when considering multiple alleles and their various interactions.
Polygenic Inheritance
Polygenic Inheritance involves multiple genes affecting a single trait, creating a continuous range of phenotypes, like skin color or height in humans. This mechanism underscores that many traits are not determined by a singular gene, but rather by interactions of several genes, often influenced by environmental factors.
The critical aspect of polygenic inheritance is its ability to explain a wide spectrum of characteristics, appealing to those interested in traits that display continuous variation rather than discrete categories. However, it also presents a challenge as predicting outcomes becomes increasingly complex, requiring analysis of multiple factors.
In summary, understanding these mechanisms of inheritance, from Mendel's initial principles to the complexities of non-Mendelian modes, helps clarify the multifaceted ways genetics influences organisms. Each mechanism has its own set of features that bring depth to the discussion, reflecting genetics' role in diversity, evolution, and applicability in various fields.
Genetic Variation
Genetic variation is a cornerstone concept within the study of genetics, harboring importance far beyond the mere understanding of DNA and heredity. It underscores how genetic differences among individuals lead to a plethora of traits within a population, laying the ground for evolution and adaptation. In this section, we'll explore what fuels this variation, examine its sources, and discuss its critical role in evolution, making it a linchpin in the genetic discourse.
Sources of Genetic Variation
In this sub-section, we delve into three primary sources of genetic variation: mutations, recombination, and gene flow. Each plays a unique role in shaping the genetic landscape that influences both species resilience and diversity.
Mutations
Mutations are considered the raw fuel of genetic variation. Essentially, they are changes that occur in the DNA sequence, which can be spontaneous or induced by external factors like radiation or chemicals. The key characteristic of mutations is their unpredictability—some are harmless, while others may be detrimental or, in some cases, advantageous.
- Contributions: They often provide new alleles, which are crucial for variation in traits.
- Benefits: For this article, mutations are a popular choice because they offer a direct understanding of how traits can arise and influence evolution.
- Unique Features: A notable feature of mutations is that they act as a double-edged sword. While they can introduce beneficial variations, they may also lead to genetic disorders or decreased fitness in certain environments.
Recombination
Recombination occurs during meiosis, where homologous chromosomes exchange genetic material, resulting in offspring with a unique combination of traits that differ from either parent. This process maintains genetic diversity within a population.
- Contributions: By shuffling the existing genetic variation, recombination produces new combinations of alleles, further enriching the gene pool.
- Benefits: Its importance lies in preserving adaptability, a cornerstone of natural selection.
- Unique Features: Unlike mutations, which create new alleles, recombination merely redistributes them. This means it can enhance the evolutionary potential of a population without introducing completely new mutations.
Gene Flow
Gene flow refers to the transfer of genetic material between populations, typically through migration. It serves as a bridge, connecting separate gene pools, often enhancing genetic diversity.
- Contributions: By allowing genes to spread across different populations, gene flow can counteract the effects of genetic drift or inbreeding, fostering a more robust population.
- Benefits: This process acts as a safeguard against the potential loss of alleles and helps maintain diversity across wider geographic areas.
- Unique Features: However, gene flow's strength can vary. In some instances, it may dilute local adaptations if not managed correctly, introducing traits that may not be suited to the local environment.
Role of Genetic Variation in Evolution
Genetic variation is not just a biological curiosity; it is the very engine of evolution. It provides the source material upon which natural selection acts, allowing populations to adapt to their environments over time.
- Natural Selection: Those individuals with advantageous traits are more likely to survive and reproduce, passing on their genes to future generations.
- Evolutionary Potential: A broad pool of genetic variation is essential for a species' ability to adapt to changing environments, from climate shifts to disease pressures.
- Example in Nature: A classic illustration of this principle can be seen in the peppered moth. Prior to the Industrial Revolution, the light-colored moths were more prevalent due to their camouflage against the light-colored trees. Post-industrial darkening of the trees led to a shift, where dark-colored moths became more favorable, showcasing the immediate impacts of genetic variation governed by environmental changes.
Genetics in Health and Medicine
The intersection of genetics and health poses significant insights into understanding diseases, tailoring treatments, and improving overall patient care. This section of the article delves into two critical areas: genetic disorders and genomics in personalized medicine. Understanding these elements is crucial as they speak to the core of how genetic factors influence health and diseases.
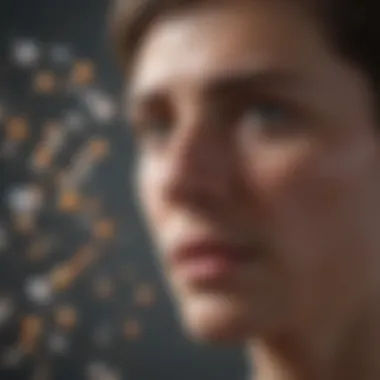
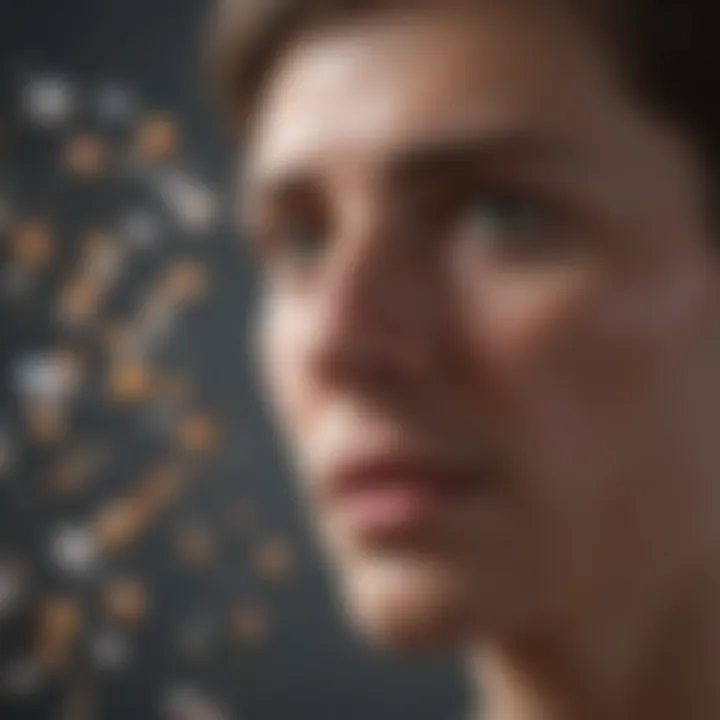
Genetic Disorders
Genetic disorders are diseases resulting from abnormalities in an individual's DNA. These can be inherited or arise spontaneously. The impact of these disorders ranges widely and often affects multiple systems within the body.
Types of Genetic Disorders
Genetic disorders can be classified into several categories, including:
- Single-gene disorders: These occur due to mutations in a single gene, such as cystic fibrosis or sickle cell disease.
- Chromosomal disorders: Resulting from changes in chromosome structure or number, an example being Down syndrome.
- Multifactorial disorders: These are caused by a combination of genetic and environmental factors, including conditions like heart disease and diabetes.
The key characteristic of these types is their distinct modes of inheritance and their varied phenotypes. Single-gene disorders, for instance, can be straightforward in their inheritance patterns, often following Mendelian principles. Each type brings its own set of consequences, both for the affected individuals and for genetic counseling and testing.
One unique feature of these disorders is the ability to screen for them. For example, newborn screenings can detect metabolic disorders before symptoms arise, permitting early intervention. However, these tests can also spark ethical debates, especially concerning the implications of genetic information for families and insurance companies.
Inheritance Patterns
The inheritance patterns of genetic disorders are critical for understanding familial risks. Patterns can be classified mainly into autosomal dominant, autosomal recessive, and X-linked.
Key characteristic of inheritance patterns is their predictive power and potential to guide medical decisions in families.
For example, in autosomal recessive disorders like cystic fibrosis, two parents must carry one copy of the mutated gene. Knowing this allows couples to make informed choices regarding family planning.
One unique aspect of these inheritance models is the ability to perform genetic testing to determine carrier status, which can profoundly impact patient management and determine potential treatments or preventative measures. However, this can also lead to anxiety around the implications of such knowledge.
Genomics and Personalized Medicine
Genomics, an area of genetics focused on the entirety of an organism's genes, represents a revolution in medical treatment. This has paved the way for an approach known as personalized medicine, where medical decisions are tailored based on an individual’s genetic makeup.
Impact of Genomics on Treatment
The impact of genomics on treatment strategies is substantial. Understanding a patient’s unique genetic profile enables healthcare providers to select medications that are more effective and cause fewer side effects. This personalized approach allows for the optimization of treatments for conditions such as cancer, where precision medicine can lead to improved outcomes.
The key characteristic of genomics in treatment is its ability to inform about drug metabolism; for instance, patients with specific genetic variants might process drugs differently. This has minimized adverse drug reactions.
The unique feature here is the emergence of targeted therapies, which have marked a shift in how conditions like breast cancer are treated. While the benefits are evident, ethical considerations remain, particularly concerning access to these treatments and the implications for precision healthcare.
Future of Personalized Medicine
Looking ahead, the future of personalized medicine holds great promise, driven by advancements in genomic research and technology. The goal is to extend the reach of genetic insights into more common diseases, moving beyond rare disorders and tailor treatment in broader contexts.
The key characteristic of future trends is the drive towards greater integration of genetic data with electronic health records, aiming to refine treatment protocols based on comprehensive data. Coupled with the ongoing advancements in AI and machine learning, this could drastically change the landscape of diagnostics and treatment.
Unexpectedly, personalized medicine may also lead to ethical dilemmas surrounding genetic privacy and data sharing. As genetic information plays a more prominent role in health care decisions, safeguarding this information will become paramount.
In summary, genetics in health and medicine shapes our understanding of diseases and their management significantly. By examining genetic disorders and the progression towards genomics and personalized medicine, we gain key insights into the future landscape of medical care.
Ethical Considerations in Genetics
The field of genetics, with its rapid advancements, brings forth significant ethical dilemmas that are critical to consider. As we delve into genetic research, from the examination of DNA to the potentials of gene editing, we encounter questions that affect individuals and society at large. Ethical considerations in genetics hold immense weight, as they shape policies, influence public perception, and guide the responsible application of genetic technologies. Understanding these ethical implications forms an essential part of recognizing how genetics intersects with moral principles, societal values, and human rights.
Ethics of Genetic Testing
Genetic testing serves as a powerful tool in diagnosing conditions before symptoms manifest. However, the ethical landscape surrounding this practice is complex. On one hand, genetic testing empowers patients with knowledge about their health risks, providing opportunities for early intervention. On the other, it raises questions concerning privacy, consent, and potential discrimination. For instance, if genetic data falls into the wrong hands, individuals could face prejudice in employment or insurance based on their genetic predispositions. This potential for misuse underscores the necessity for regulations that protect individuals' genetic information and uphold their autonomy in deciding whether to undergo testing.
Ethical discussions about genetic testing also involve the aspect of informed consent. Patients must be fully educated regarding the implications of the tests and the far-reaching consequences of their results on themselves and their families. As genetic testing technologies become increasingly accessible, the conversation surrounding these ethical considerations must evolve to safeguard against exploitation and misuse.
Gene Editing Technologies
With the dawn of gene editing technologies, particularly CRISPR-Cas9, the capacity to modify genetic sequences has stirred a wave of ethical debates.
CRISPR-Cas9 Overview
CRISPR-Cas9 represents a groundbreaking advancement in genetic engineering. It allows researchers to precisely target and edit specific genes within organisms. This manipulation can correct genetic defects and potentially eradicate hereditary diseases. The simplicity and cost-effectiveness of CRISPR-Cas9 distinguish it from previous methods, making it a popular choice for researchers aiming to push the envelope of genetic possibilities.
One notable aspect is its precision; researchers can modify DNA sequences down to individual base pairs. This high degree of accuracy yields significant potential for therapy, promising effective solutions for genetic disorders like cystic fibrosis and sickle cell anemia. However, the ease of use also raises concerns regarding unintended consequences. Modifications might lead to unforeseen genetic complications that could be passed down through generations.
Ethical Implications of Gene Editing
The ethical implications of gene editing technologies like CRISPR-Cas9 are profound. The prospect of
Influence of Genetics on Behavior
Understanding the influence of genetics on human behavior is like peering through a kaleidoscope: the patterns are complex, the images constantly shifting yet interconnected. This section aims to illuminate how genetic factors can play pivotal roles in shaping personality traits and behavioral dispositions. With the current advancements in genetic research, it has become increasingly clear that while the environment molds us, our genetic makeup provides a foundational blueprint for many aspects of our behavior. This understanding not only enriches our grasp of individual differences but also paves the way for further inquiries into psychological and medical fields.
Genetic Factors in Personality
The concept of personality has long intrigued psychologists. A fascinating angle to explore is how much of our personality is rooted in genetics versus shaped by our environment. Recent studies suggest that genes account for a significant portion of personality traits. For instance, twin studies have consistently shown that identical twins—who share nearly 100% of their DNA—tend to exhibit remarkably similar personality characteristics, even when raised in different environments. This phenomenon leads us to infer that certain traits, such as extroversion or conscientiousness, can be heavily influenced by hereditary factors.
- Personality Traits and Genetics
Several specific traits have been linked to genetic predispositions:
- Extraversion: Often correlated with dopamine-related genes, influencing the drive for social interactions.
- Neuroticism: Tied to genetic variations that affect emotional regulation, potentially leading to anxiety or mood disorders.
- Agreeableness: Linked to social behavior tendencies, shaping how individuals interact with others.
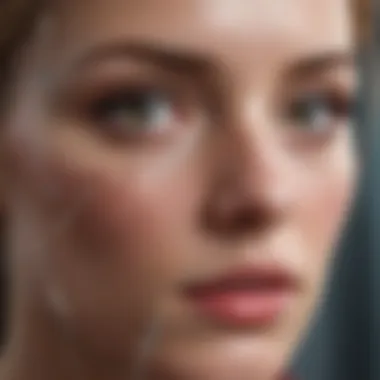
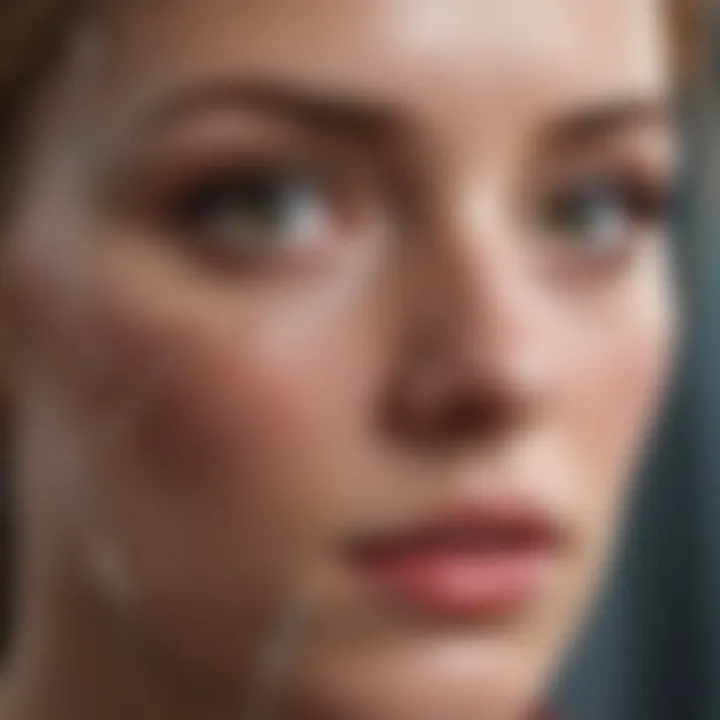
By understanding the genetic underpinnings of these traits, we can better appreciate how they manifest in daily interactions and long-term relationships.
Heritability of Behavioral Traits
When discussing behavior, the term heritability often arises. This measure explains how much of the variation in a trait can be attributed to genetic factors, differing significantly from the notion that behavior is a fixed entity. It’s crucial to note that heritability does not imply immutability—individuals are not predetermined solely by their genes; rather, behaviors can adapt through life experiences and social contexts.
"Behavioral traits, much like a carefully tended garden, can flourish or wilt depending on the nurturing received over time."
For instance, consider the heritability of aggressive behavior. Research indicates that genetic factors can account for a considerable percentage of aggression, but these impulses can be significantly tempered or exacerbated by environmental circumstances, such as parental guidance or peer influence.
- Key Points to Consider:
- Variability across populations: The heritability of certain behaviors can vary based on environmental conditions.
- Interactions with upbringing: Positive nurturing can enhance desirable behaviors, while adverse environments can exacerbate negative ones.
- Complex interplay between genes and environment: It’s often a dance between nature and nurture, muddling the waters further.
In summary, genetics undeniably shapes our personalities and behaviors, yet the intricate dance with environmental influences cannot be overlooked. This ongoing dialogue between genetics and surroundings is vital for an enriched understanding of human behavior, helping us tackle psychological constructs with both depth and nuance.
Applications of Genetic Research
Genetic research has a myriad of practical applications that extend far beyond the laboratory. It plays a crucial role in agriculture, forensic science, medicine, and many more fields. This section will delve into how genetic insights are shaping our world, exploring their benefits and the ethical considerations they bring.
Genetics in Agriculture
Genetically Modified Organisms
Genetically Modified Organisms (GMOs) are a prime example of genetic research applied to agriculture. These organisms have had their DNA altered using genetic engineering techniques, which can make them more resilient to pests, diseases, or climate challenges. The hallmark of GMOs lies in their enhanced traits, such as drought resistance or increased nutritional value. This adaptability makes them a compelling choice for food production, especially in regions facing environmental stress.
However, while GMOs can boost crop yields and reduce reliance on chemical pesticides, they are not without controversy. Critics often point to potential health risks and environmental concerns, suggesting that long-term effects remain untested. As a result, consumers and agricultural bodies frequently find themselves in a tug-of-war, trying to balance innovation with caution.
Benefits and Risks
The benefits of GMOs are multifaceted. For instance, they can lead to:
- Increased Crop Yields: Higher productivity per acre means more food can be produced to feed growing populations.
- Reduced Environmental Impact: Certain GMOs require fewer chemical inputs, which can lessen agricultural run-off.
- Enhanced Nutritional Profiles: Some GMOs have been engineered to contain more vitamins or essential nutrients.
On the flip side, there are significant risks tied to their use:
- Loss of Biodiversity: The prevalence of a few genetically modified strains may lead to a decrease in crop diversity.
- Resistance Development: Pests can evolve resistance to genetically modified defenses, leading to a cycle of increased chemical use.
- Economic Concerns: Farmers may become reliant on expensive seeds, leading to financial strain.
Genetics and Forensic Science
DNA Profiling
DNA profiling is a critical tool in forensic science, with far-reaching implications for criminal justice and personal identification. This technique allows for the analysis of an individual's unique DNA sequence, which can be compared against samples found at crime scenes. The primary characteristic of DNA profiling is its specificity; even minute samples can yield compelling matches, which is why it's invaluable in determining guilt or innocence.
However, it's important to note that DNA evidence is not infallible. Laboratory errors, contamination, or misinterpretation can lead to wrongful conclusions. Therefore, while DNA profiling significantly enhances investigative capabilities, it must always be handled with care to uphold the integrity of legal processes.
Applications in Crime Solving
The application of genetic research in crime solving extends beyond mere DNA matching. It encompasses:
- Cold Case Resolution: Techniques can breathe new life into decades-old unsolved cases, providing closure for families.
- Exoneration: DNA testing has led to the exoneration of wrongfully convicted individuals.
- Understanding Crime Patterns: Genetic data can help police understand trends in criminal behavior or gang affiliations.
Yet, this technology is not without its dilemmas. Ethical considerations come into play, especially regarding privacy and consent. Society must tread carefully, ensuring that the benefits of genetic advancements do not compromise civil liberties or lead to misuse of information.
"Genetic research is not just about understanding the past; it's about shaping the future in agriculture and justice in ways that are profound and lasting."
Future Directions in Genetic Research
The field of genetics continues to evolve rapidly, and understanding its future directions is crucial for anyone invested in biological sciences. This section aims to highlight pivotal elements that could shape genetic research in the years ahead. From novel technologies to the synergy with artificial intelligence, these aspects will not only enhance scientific inquiry but also address significant societal needs, making them indispensable in today’s research landscape.
Emerging Technologies
Emerging technologies in genetics encompass a variety of innovative tools and methodologies that hold potential to revolutionize the field. Some noteworthy advancements include:
- Next-Generation Sequencing (NGS): This technology allows for rapid sequencing of entire genomes, significantly reducing the time and cost compared to traditional methods. Its applications range from understanding genetic disorders to exploring evolutionary biology.
- CRISPR-Cas9 and Beyond: While CRISPR is well-known for gene editing, newer iterations and methods are being developed to refine its precision, reduce off-target effects, and expand its uses in therapeutic contexts.
- Single-Cell Genomics: This approach focuses on studying individual cells rather than bulk tissue, offering insights into cellular heterogeneity. It’s especially useful in cancer research, where the genetic diversity of tumor cells can influence treatment strategies.
- Synthetic Biology: Merging biology and engineering, synthetic biology enables the creation of new biological parts, devices, and systems. This opens doors to novel applications, such as biofuel production, which could address energy needs sustainably.
"Genetic research is not just about studying DNA anymore; it’s about reshaping our understanding of life itself."
The Role of Artificial Intelligence in Genomics
Artificial Intelligence (AI) is set to play a transformative role in the future of genetics. With massive datasets generated by genetic research, AI presents several advantages:
- Data Analysis: AI algorithms can process and analyze vast amounts of genetic data faster than humans. This capability is vital for identifying patterns and correlations that might remain hidden otherwise.
- Predictive Modeling: Machine learning models can predict the likelihood of genetic disorders based on an individual's genetic makeup, potentially leading to earlier interventions and personalized treatment plans.
- Drug Discovery: AI can expedite the drug discovery process by predicting how drugs will interact with specific genetic variants. This may improve the efficacy of treatments and reduce adverse effects.
- Integrative Genomics: By combining genetic data with other biological information, AI can provide comprehensive insights into complex diseases, facilitating targeted therapies.
Closure
In drawing this exploration of genetics to a close, it's imperative to reflect on the multifaceted nature of genetics and its vast implications. This section synthesizes the understandings gathered throughout, highlighting key elements, benefits, and considerations that weave together the narrative of genetic inquiry.
A central theme that emerges is the essential role genetics plays across various domains, from medicine to agriculture and beyond. The study of genetics isn’t merely about deciphering the code of life; it’s about poner our understanding of biological frameworks into practice. One standout benefit is how genetic research fuels advancements in personalized medicine, which tailors treatments based on an individual’s unique genetic profile. This not only enhances efficacy but also minimizes adverse effects, making medical interventions more precise.
Furthermore, the ethical considerations discussed throughout shed light on the responsibility inherent in genetic advancements. As technologies evolve, particularly with tools such as CRISPR, the dialogue surrounding their ethical implications remains crucial. We must tread carefully, balancing innovation with moral considerations. As the age-old saying goes, "Just because we can, doesn’t mean we should." Thus, a deeper understanding of this balance is needed for sound decision-making that aligns science with societal values.
"The understanding of genetics shapes not just science, but the fabric of society itself."
Finally, the exploration into behavioral genetics reinforced how intertwined genetics and behavior can be. While it’s tempting to oversimplify complex human behaviors to genetic determinants, a nuanced perspective recognizes the interplay of environment and genetics in shaping individuals.
In summary, the significance of genetics extends far beyond the confines of academic inquiry. It permeates various facets of modern life, influencing health outcomes, agricultural practices, and ethical frameworks. As genetic research continues to advance, it pushes the boundaries of what we understand about ourselves and the world around us, paving the way for future discoveries that could dramatically reshape our existence.