Exploring EFM and AFM: Applications in Science
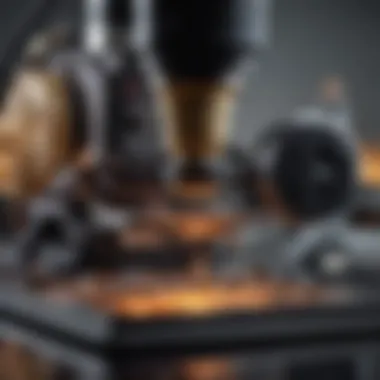
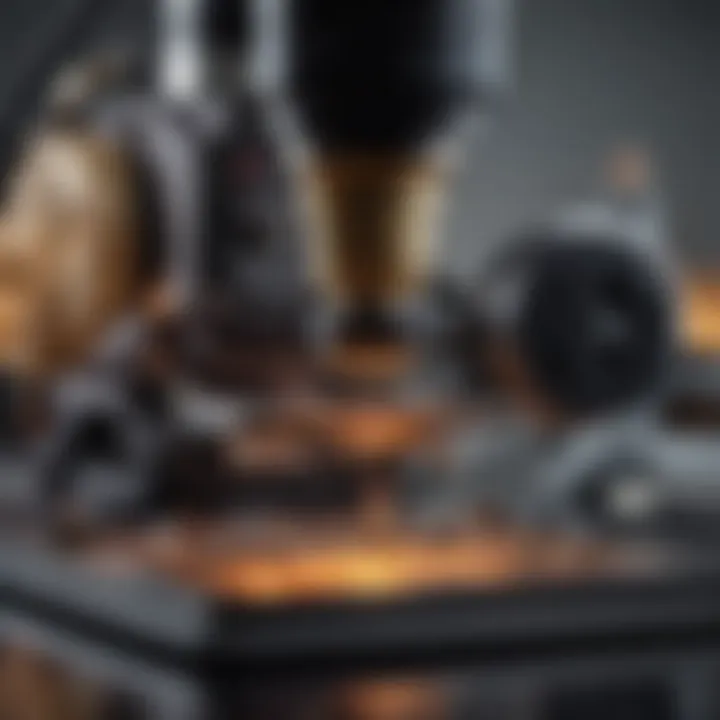
Intro
The study of microscopy has evolved significantly over the past few decades, leading researchers to utilize advanced techniques like Electrochemical Force Microscopy (EFM) and Atomic Force Microscopy (AFM). These methodologies provide detailed insights into material properties and biological systems at the nanoscale. Understanding the principles, applications, and implications of these techniques is essential for anyone engaged in scientific research today. This article aims to explore the intricate world of EFM and AFM, highlighting their unique features and contributions across various disciplines.
Recent Advances
Latest Discoveries
Recent developments in EFM and AFM have unveiled numerous exciting possibilities in scientific investigation. Researchers have made notable strides in utilizing EFM for electrochemical imaging, enabling real-time observation of chemical reactions at interfaces. This provides new avenues for studying materials' behavior during catalytic processes or energy storage applications, advancing fields like battery technology and catalysis.
In the realm of AFM, innovations such as high-speed imaging and multi-modal approaches have emerged. These cutting-edge techniques allow scientists to obtain topographical and mechanical information simultaneously, enhancing the understanding of material properties at the nanoscale. For instance, the integration of AFM with optical microscopy enables comprehensive studies of live biological samples.
Technological Innovations
Technological breakthroughs in instrumentation have propelled EFM and AFM forward. Improvements in cantilever sensitivity and stability have led to enhanced resolution and accuracy, vital for characterizing delicate samples. Specific advancements include:
- Modifications in cantilever designs: Enhanced sensitivity facilitates the detection of minute forces.
- Integration of machine learning: This aids in data processing and analysis, allowing for deeper insights.
- Real-time imaging capabilities: These innovations have revolutionized how researchers observe dynamic processes in materials and biological systems.
Understanding these modern advancements is crucial because they demonstrate the ongoing transformation in microscopy fields, enabling researchers to explore uncharted territories.
Methodology
Research Design
The methodological framework of EFM and AFM studies typically incorporates both experimental and computational approaches. Researchers define clear objectives, ensuring that chosen techniques align with their goals. EFM requires a precise setup that includes a potentiostat to control the electrochemical environment while measuring the force interaction. Conversely, AFM setups focus on adjusting scanning parameters to capture high-resolution images of samples.
Data Collection Techniques
For effective data collection, specific protocols and equipment are essential:
- EFM Techniques: Researchers often adopt dual-frequency techniques to enhance the signal-to-noise ratio. This allows for the effective mapping of electrostatic forces produced by surface charges.
- AFM Techniques: AFM data collection often employs tapping mode to minimize sample damage and achieve higher resolution.
Both EFM and AFM require careful execution and calibration to ensure reproducibility and validity of results. Overall, the processes involved are highly specific to the type of analysis being conducted, contributing to the reliability and depth of the findings.
Intro to EFM and AFM
The realm of scientific research is increasingly leveraging advanced microscopy techniques to provide deeper insights into various materials and biological systems. Two prominent methods in this domain are Electrochemical Force Microscopy (EFM) and Atomic Force Microscopy (AFM). Both techniques, while distinct in their operational principles, share a common goal of enhancing our understanding of the nanoscale world.
In this section, we will set the context for these methods, highlighting their unique aspects and their significance in the scientific community.
Setting the Context
Electrochemical Force Microscopy and Atomic Force Microscopy are pivotal tools in many fields, including materials science, biology, and nanotechnology. These techniques are designed to offer high-resolution imaging and analysis of surfaces at the atomic level. EFM is particularly specialized for studying electrochemical properties and reactions, while AFM provides insights into surface topography and mechanical properties.
To appreciate the relevance of EFM and AFM, one must consider their foundational roles in characterizing materials and biomolecules. For instance, AFM can dissect the physical characteristics of a protein at the nanoscale, revealing its structural complexities. Meanwhile, EFM can assess the influence of electrochemical processes in real-time, an invaluable asset in energy-related research.
Importance in Scientific Research
The importance of EFM and AFM in scientific research cannot be overstated. They have introduced revolutionary advancements, enabling researchers to observe phenomena that were previously invisible or difficult to analyze. Here are some key points that underline their contributions:
- High Resolution: Both techniques are capable of achieving atomic-level resolution, which is crucial for detailed analysis of structures.
- Diverse Applications: EFM and AFM are applicable in a wide range of research areas, from understanding molecular interactions in biology to exploring new materials for batteries in energy storage research.
- Real-time Monitoring: With EFM, researchers can observe changes during electrochemical reactions, thus offering insights into dynamic processes that occur at the nanoscale.
The potential of EFM and AFM extends beyond mere observation; they are essential not just for visualization but also for influencing future technological innovations.
As we delve deeper into their fundamental principles and applications, it is essential to recognize how these techniques not only contribute to existing knowledge but also pave the way for novel discoveries in various scientific disciplines.
Fundamentals of Atomic Force Microscopy (AFM)
Atomic Force Microscopy (AFM) serves as a foundational technique in the realm of nanotechnology and materials science. Its significance lies in its ability to provide high-resolution imaging, thereby revealing the surface characteristics of materials at the atomic and molecular levels. Understanding the fundamentals of AFM is essential for researchers who wish to apply this technology innovatively in various scientific fields.
Basic Principles and Mechanism
The operating principle of AFM relies on measuring the interaction forces between a sharp probe and the sample surface. The probe is mounted on a cantilever, which bends in response to attractive or repulsive forces. This deflection is measured with a laser beam that is focused on the cantilever. This allows for the generation of topographic maps of the surface. The very small size of the probe enables imaging with extraordinary detail, typically in the nanometer scale.
Modes of Operation
AFM employs various modes to suit diverse research needs. Each mode has unique attributes that dictate its application potential.
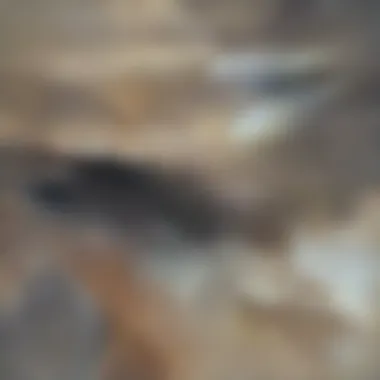
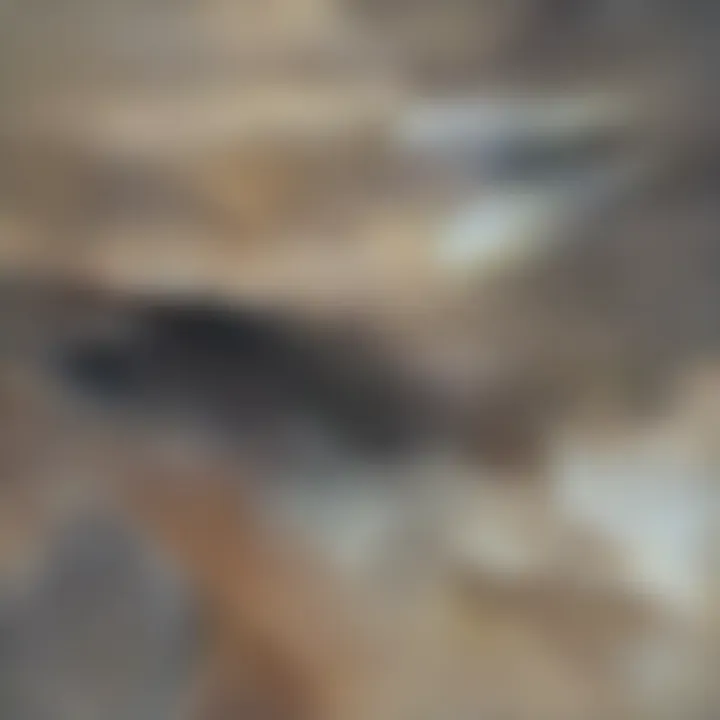
Contact Mode
In contact mode, the probe directly contacts the surface of the sample. This mode is notable for its ability to provide high-resolution topographic measurements. Contact mode is beneficial in various applications, particularly where fine detail of surface structures is paramount. However, one disadvantage is its potential to damage soft or delicate samples due to the contact force between the probe and the surface. This may limit its use with certain biological specimens or soft materials.
Tapping Mode
Tapping mode uses an oscillating probe that intermittently contacts the surface. This method allows for imaging with reduced lateral forces. The major advantage of tapping mode is its ability to image softer samples without causing damage. Moreover, it facilitates the collection of data about both topography and material properties such as stiffness. However, tapping mode can sometimes yield lower resolution images compared to contact mode, depending on the sample being analyzed.
Non-contact Mode
Non-contact mode operates without direct contact between the probe and the sample surface. This mode relies on measuring forces that decay with distance, such as van der Waals forces. It is particularly useful for imaging sensitive samples, as there is a minimal chance of inducing damage. The primary drawback is that non-contact mode may produce lower resolution images than the contact mode. It is a preferred choice for observing delicate biological materials or organic films.
Instrumentation Overview
A thorough understanding of the instrumentation involved in AFM is pivotal for its effective utilization. The components and scanning processes are integral to achieving accurate and reproducible results.
Components of an AFM
The main components of an AFM include the cantilever, the probe, the laser, and the optical detector. Each part plays a crucial role in the overall functionality of the AFM. The cantilever's deflection results from the interactions with the sample, and the optical detection system translates this into a measurable signal. This arrangement ensures the high sensitivity and precision that AFM is known for. One weakness could be the sensitivity of these components, making proper calibration and maintenance vital for reliable results.
Scanning Process
The scanning process involves the probe traversing the sample surface in a controlled manner. The AFM software translates the deflection data into a three-dimensional topographic map of the surface. This efficient procedure allows for the analysis of surface morphology at nanoscale resolution. However, the scanning speed and resolution settings can influence the outcome, making it essential to optimize these parameters for each specific study. Inadequate optimizing can lead to misinterpretation of results, which hinders the overall effectiveness of AFM in practical applications.
"Understanding the fundamentals of AFM is key for researchers aiming to employ this cutting-edge technology in novel applications across various scientific fields."
This exploration of AFM provides a comprehensive understanding of its principles, modes, components, and process. Each aspect contributes to its revolutionary role in advancing scientific research.
Applications of AFM in Various Fields
Atomic Force Microscopy (AFM) has become a cornerstone technique across various fields of scientific research. Its ability to manipulate and image surfaces down to the atomic level provides an array of benefits. Researchers can use it to gain insights into the structure and properties of materials while contributing significantly to advancements in technology and understanding species at the molecular level. In this section, we will explore the applications of AFM in key areas such as materials science, biology, and nanotechnology. Each field capitalizes on the unique capabilities of AFM, revealing intricate details that traditional microscopy cannot deliver.
Materials Science
Materials science stands to gain tremendously from AFM applications. This field involves the study and development of new materials, with AFM providing critical insights into their structure at the nanoscale.
AFM can determine the mechanical properties, such as hardness and elasticity, of materials like polymers, composites, and thin films. By measuring the force required to indent a material, researchers derive crucial data about its properties. AFM also assists in the analysis of surfaces and interfaces, which is essential in material development. This application is particularly vital in developing advanced coatings and semiconductor materials.
Benefits of AFM in materials science include:
- High-resolution imaging: AFM images can reach atomic resolution, allowing researchers to observe defects or irregularities that may impact material performance.
- Three-dimensional data: Unlike other techniques, AFM provides three-dimensional topographical data that can be invaluable in characterizing surfaces.
- Versatility: AFM can be used in various environments, including liquid, proving useful for analyzing thin films or coatings under real-life conditions.
Biology and Biomedical Research
AFM plays a significant role in biology and biomedical research. An important advantage is that it allows researchers to study soft biological samples without any damage, unlike other microscopy methods. The resolution of AFM enables the examination of cell membranes, proteins, and DNA at an atomic scale.
The ability to monitor molecular interactions is vital in drug discovery and development. AFM can be used to map the topography of cells and tissues, providing insights into biological processes such as cell adhesion, migration, and differentiation.
Key points of AFM's application in biology include:
- Live cell imaging: AFM can capture live cells in a physiological environment, allowing observation of dynamic cellular processes.
- Molecular force measurements: The technique measures interactions between biomolecules, aiding in the understanding of molecular mechanisms in diseases.
- Biomaterial characterization: AFM assists in assessing the properties of biomaterials, which is crucial for implants and tissue engineering.
Nanotechnology
In the field of nanotechnology, AFM is indispensable for the fabrication and characterization of nanoscale materials. Nanoscale structures can be studied with precision, which is vital for developing new nanomaterials and devices.
AFM allows researchers to manipulate nanoscale objects and obtain vital data like surface roughness, which directly impacts material properties and device performance. Additionally, AFM techniques can deliver information on electrical, magnetic, and thermal properties at the nanoscale. This means that AFM not only aids in the characterization of nanomaterials but also serves as a tool for assembly in nanoscale engineering.
Benefits of AFM in nanotechnology include:
- Precision: AFM provides high accuracy and resolution, critical for manipulating individual nanostructures.
- Multi-functionality: It combines imaging capabilities with the measurement of various physical properties.
- Integration in fabrication processes: AFM is becoming pivotal in integrating nanomaterials into larger systems for electronic and optical applications.
By offering unparalleled insights into the nanoscale world, AFM fuels innovation across materials science, biology, and nanotechnology.
As we continue to explore the potential of AFM, its diverse applications present a promising horizon for scientific research.
Preface to Electrochemical Force Microscopy (EFM)
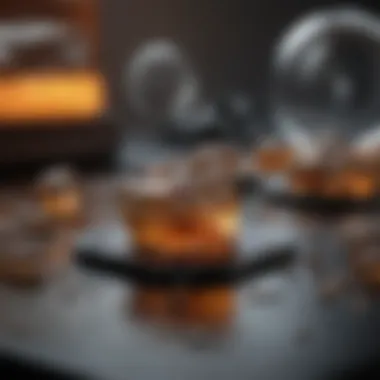
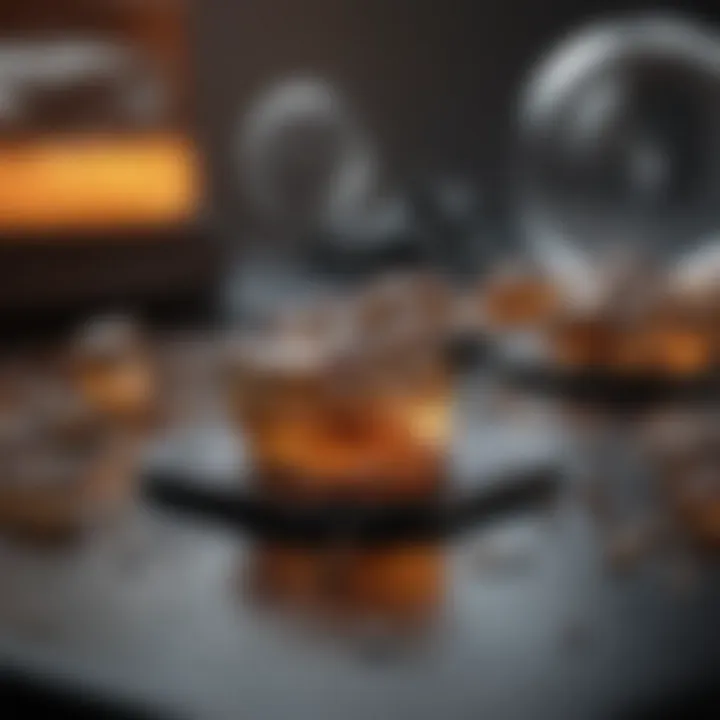
Electrochemical Force Microscopy (EFM) represents a significant evolution in the field of nanoscale imaging and characterization. This technique incorporates the principles of Atomic Force Microscopy (AFM) while introducing electrochemical capabilities that allow for the study of conductive materials in an electrochemical environment. The integration of electrochemistry with atomic force microscopy extends the range of applications significantly, making EFM a powerful tool for scientists and researchers.
This article aims to dissect the principles and workings of EFM in a detailed manner. By explaining the operational principles, EFM reveals its advantages in tackling complex problems that conventional techniques may struggle with. The relative ease with which EFM can be utilized to explore surface interactions at the nanoscale brings forward exciting prospects in science, particularly in materials and electrochemistry.
Principles of EFM
EFM operates based on principles similar to those of AFM. The technique employs a sharp conductive probe that interacts with a sample surface through electrostatic and van der Waals forces. However, the distinguishing factor of EFM is its ability to combine these interactions with electrochemical measurements. This combination allows researchers to study surface phenomena in real-time or under varying conditions, such as changing the potential of the sample.
When the conductive probe approaches the sample, it measures the forces acting upon it, which can be influenced by the electrochemical activity happening at the surface. The feedback mechanism adjusts the probe's height according to the forces detected, allowing for precise mapping of the surface's topography and electrical properties. Additionally, EFM can provide insights into the chemical reactivity of materials, which is critical in fields like energy storage and conversion.
Key Differences Between EFM and AFM
While EFM and AFM share core mechanisms, several differences set them apart. Understanding these differences is crucial for selecting the appropriate technique for specific applications:
- Sensitivity to Electrochemical Environments: EFM is specifically designed for use in liquid or gaseous environments where electrochemistry can occur. Traditional AFM, on the other hand, is generally utilized in air or vacuum conditions.
- Capability for Complex Data Interpretation: With EFM, researchers can gather both topographical and electrochemical data simultaneously. This dual capability allows for a deeper understanding of material properties.
- Specific Applications: EFM finds its applications in areas such as:
- Energy Storage Materials: Analyzing battery components under operational conditions.
- Corrosion Studies: Investigating the durability of materials in electrolyte solutions.
- Electrocatalysis: Examining the efficiency of catalysts in chemical reactions.
Understanding the differences between EFM and AFM can guide researchers in choosing the right technique for their experimental needs. The choice depends on the specific properties they wish to explore and the conditions under which they are studying their materials.
Applications of EFM in Scientific Research
The significance of Electrochemical Force Microscopy (EFM) in scientific research is profound. This innovative technique merges electrochemistry and microscopy, enabling detailed analyses at the nanoscale. EFM provides insights into materials' electrochemical behaviors, which is critical in various applications including energy storage, corrosion studies, and electrocatalysis. Understanding these applications is essential for researchers looking to advance technology and improve material efficiency.
Energy Storage Materials
Energy storage materials are crucial for the development of batteries and capacitors. EFM allows scientists to analyze these materials at the nanoscale, providing valuable information about their electrochemical properties. By examining the surface interactions between electrodes and electrolytes, EFM helps to understand how materials behave during charge and discharge cycles. This knowledge can lead to the design of more efficient and longer-lasting energy storage systems.
- Surface Mapping: EFM can create detailed maps of the surface properties of energy storage materials, identifying areas of high activity.
- Electrode Fabrication: Insights gained from EFM can improve processes for fabricating electrodes, ultimately enhancing performance and stability in energy devices.
Corrosion Studies
Corrosion can severely impact the longevity and safety of materials used in infrastructure, vehicles, and various industrial applications. EFM provides a powerful tool for studying localized corrosion processes. It can measure electrochemical potential distribution on surfaces, identifying potential corrosion sites. This ability enables proactive measures to enhance material resilience.
- Localized Measurements: EFM can detect small changes in the electrochemical environment, allowing for targeted corrosion prevention strategies.
- Material Selection: By understanding corrosion rates under different conditions, researchers can make informed decisions about material choices in engineering applications.
Electrocatalysis
Electrocatalysis is vital for advancing sustainable energy technologies, like fuel cells and electrolyzers. EFM facilitates the investigation of electrocatalytic surfaces, enabling researchers to study reaction kinetics and efficiency. This technique contributes to optimizing catalysts for various reactions crucial in energy conversion processes.
- Performance Evaluation: EFM aids in assessing the effectiveness of different catalytic materials, allowing researchers to identify the most promising candidates.
- Reaction Mechanisms: By analyzing the electrochemical interactions at play, EFM provides insights into the fundamental mechanisms behind electrocatalytic reactions.
Combining EFM with other analytical techniques can yield comprehensive insights into complex electrochemical systems, enhancing research depth.
In summary, the applications of EFM across energy storage materials, corrosion studies, and electrocatalysis underline its importance in scientific research. By offering in-depth analyses of electrochemical processes, EFM is paving the way for innovations in technology and materials.
Comparative Analysis of EFM and AFM
In the realm of scientific research, particularly in materials science and nanotechnology, comparing Electrochemical Force Microscopy (EFM) and Atomic Force Microscopy (AFM) is essential. This comparative analysis focuses on understanding their unique methodologies, strengths, and application contexts. Moreover, this analysis is crucial for selecting the right technique based on specific research goals and desired outcomes.
EFM and AFM both use a sharp probe to scan surfaces at the nano-scale. Nevertheless, they differ in their operational principles and the type of data they provide. AFM primarily offers topographical images, while EFM provides insights into electrochemical properties at the nanoscale. Thus, understanding their strengths and limitations is vital for researchers.
Strengths and Limitations
Strengths of AFM:
- High-resolution imaging: AFM allows for imaging of surfaces with atomic resolution, providing detailed topographical maps.
- Versatility: AFM can operate in various modes, including contact, tapping, and non-contact, catering to different sample types.
- Material characterizations: It can measure cantilever deflections to gather data on surface forces and mechanical properties.
Limitations of AFM:
- Complex sample preparation: Some materials may require specific conditions or coatings, complicating the experimental setup.
- Scan speed: AFM may have slower acquisition speeds compared to EFM, limiting its application in scenarios demanding rapid imaging.
Strengths of EFM:
- Electrochemical insight: EFM can probe electronic and ionic interactions, making it invaluable in energy storage and electrocatalysis studies.
- Simultaneous measurements: EFM enables concurrent topographical and electrochemical characterizations, providing comprehensive data.
Limitations of EFM:
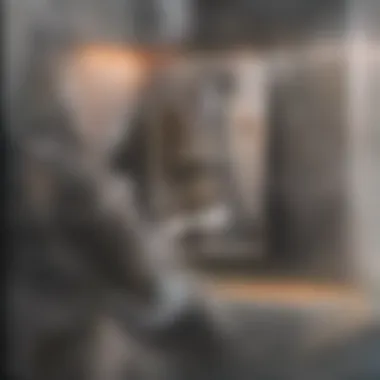
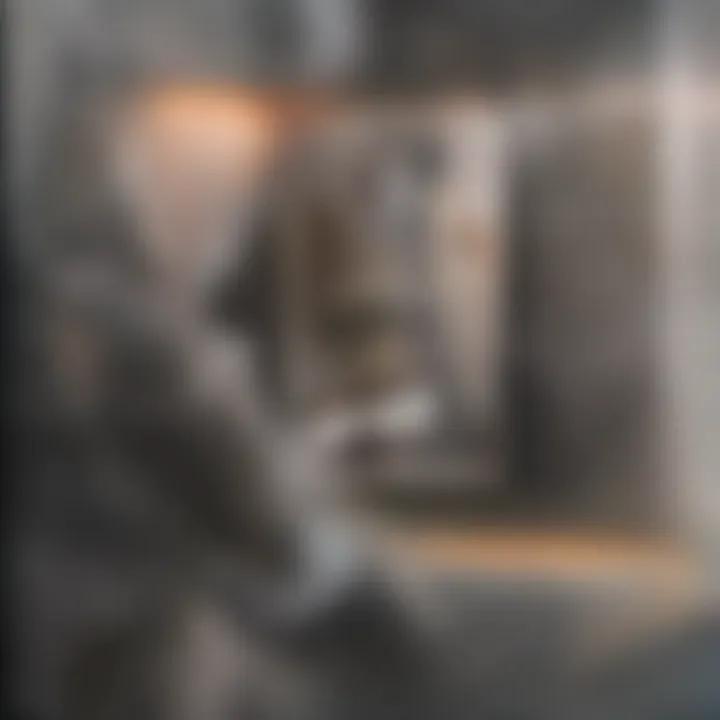
- Sensitivity to environmental factors: EFM measurements can be affected by humidity and temperature, which may introduce variability in results.
- Less versatility in imaging modes: Compared to AFM, EFM modes are limited, primarily focusing on electrochemical analysis.
Synergistic Use in Research
Combining the advantages of EFM and AFM can provide a more holistic understanding of materials at the nanoscale. Researchers often employ both techniques in tandem to leverage their complementary strengths. For instance, using AFM for high-resolution imaging followed by EFM for electrochemical characterization can yield detailed insights into the structure-property relationships of materials.
Some specific integrations include:
- Investigating nanostructured catalysts: Using AFM to visualize surface topology and EFM to assess catalytic activity relates morphological features to performance.
- Energy materials research: Employing both techniques helps understand charge transport mechanisms and surface interactions in batteries and supercapacitors.
"The combined use of EFM and AFM can reveal intricate details about the electrochemical behavior of nanoscale materials, thus accelerating the advancement of innovative technologies."
In summary, a comparative analysis of EFM and AFM reveals their distinct merits and challenges. Understanding these nuances enhances research outcomes and propels scientific exploration in relevant fields.
Challenges in EFM and AFM Techniques
The exploration of Electrochemical Force Microscopy (EFM) and Atomic Force Microscopy (AFM) presents various challenges. Understanding these limitations is essential for researchers to effectively utilize these techniques. Addressing the challenges can improve the methods and increase the accuracy of the results.
Technical Limitations
Both EFM and AFM possess inherent technical limitations that can affect their performance in research.
- Resolution and Sensitivity: Even though AFM provides high spatial resolution, limitations exist for specific types of samples. The sensitivity to noise can hinder performance, especially for softer materials.
- Scan Rate: The scan rate in AFM is limited due to the need for accurate measurements. A slow scanning process decreases the time efficiency but increases accuracy. Balancing these aspects can be tricky.
- Sample Preparation: The need for sample preparations can sometimes result in alterations. For example, delicate samples can be damaged during the fastening process, leading to less reliable results.
- Environmental Conditions: Environmental factors such as temperature and humidity can disproportionately affect EFM outcomes, leading to variable results. Maintaining stability in these conditions is challenging and often requires sophisticated setups.
Interpretation of Results
Interpreting results from EFM and AFM requires careful consideration of multiple factors.
- Complex Data Sets: The data generated can be complex. Often, understanding the influence of various parameters on the outcome is needed. Researchers must differentiate between the physical characteristics of the sample and the effects of the measurement tools themselves.
- Data Correlation: Establishing a meaningful correlation between different data collected can be difficult. It is key to relate image quality and other measurements to ensure valid conclusions.
- Reproducibility: Consistency in results is vital for scientific research. However, variations in sample environment or technique can lead to different results despite identical setups.
Reproducibility in scientific experiments is crucial for validating findings. Without it, the application of EFM and AFM can lead to misleading conclusions.
- Skill and Training: The skills of the operators significantly influence the interpretation of results. Adequate training is important to avoid common pitfalls and misinterpretations that could skew research outcomes.
In summary, while EFM and AFM offer valuable insights into various scientific fields, researchers must navigate technical challenges and interpretive difficulties diligently to achieve reliable and meaningful results.
Future Prospects of EFM and AFM
The field of microscopy is witnessing rapid advancements, particularly with Electrochemical Force Microscopy (EFM) and Atomic Force Microscopy (AFM). Understanding the future prospects of these techniques is essential for scientists and researchers aiming to leverage their unique capabilities in various disciplines. As technology progresses, EFM and AFM will likely evolve, offering improved functionalities and broader applications.
Emerging Technologies
New developments in microscopy are frequently emerging, promising enhanced capabilities and precision. One such technology is the integration of machine learning algorithms into EFM and AFM. These advanced computational techniques can significantly improve data acquisition and interpretation, allowing for more accurate measurements and analysis.
Other promising advancements include the miniaturization of equipment. Smaller devices will enhance usability in diverse environments, from laboratories to fieldwork. Moreover, combining AFM with techniques like spectroscopy or advanced imaging may reveal even more detailed information at the nanoscale.
Additionally, the incorporation of more sensitive sensors in EFM can lead to detecting electrochemical processes with greater resolution. This will enable researchers to study interactions at the molecular level, enhancing our understanding of catalytic processes in areas like sustainable energy and environmental chemistry.
Potential Areas of Expansion
The applications of EFM and AFM are set to expand significantly. Firstly, in materials science, the characterization of advanced materials will be crucial for developing new nanostructures and composites. This knowledge is essential for industries focusing on electronics, energy storage, and biomaterials.
In biology, the potential applications are vast. EFM and AFM can help study live cells, providing real-time insights into cellular processes. This could lead to breakthroughs in drug development and disease research.
Moreover, interdisciplinary research combining EFM and AFM with fields like quantum computing or synthetic biology presents exciting possibilities. Exploring these intersections may yield innovative solutions to complex problems.
"The future of microscopy lies at the intersection of technology and innovation, opening new avenues for research and discovery."
Finale
The conclusion serves as a critical component in reinforcing the overall insights presented throughout the article on Electrochemical Force Microscopy (EFM) and Atomic Force Microscopy (AFM). This section summarizes the essential findings and practical implications of these advanced imaging techniques. Emphasizing their applications in various scientific fields illustrates not only their versatility but also their indispensable contributions to ongoing research. Understanding the conclusions will guide professionals and students in appreciating the significance of EFM and AFM for future experimental designs and innovations.
Summary of Key Points
- EFM and AFM Techniques: Both EFM and AFM provide unparalleled insights at the nanoscale, facilitating advancements in materials science, nanotechnology, and biology.
- Applications: Key applications highlighted include energy storage materials, corrosion studies, and electrocatalysis, showcasing their relevance in multiple disciplines.
- Challenges: Addressing technical limitations and the interpretation of results is crucial for effective application in real-world scenarios.
- Future Directions: Emerging technologies and potential areas for expansion were discussed, emphasizing the continuing evolution of these methodologies.
It is evident that EFM and AFM are not merely tools for analysis; they are gateways to understanding complex systems at atomic and molecular levels. As research progresses, the insights gained from these techniques will undoubtedly lead to transformative innovations across various sectors.
Final Thoughts on Research Applications
In the realm of scientific research, EFM and AFM stand out due to their high precision and versatility. As these technologies develop, their integration into varied fields will likely yield substantial progress. Researchers must remain attuned to advancements and explore how these techniques can be adapted to fulfill specific research needs.
The integration of EFM and AFM into mainstream scientific investigations is but the beginning. As they become increasingly refined, their potential to influence future discoveries and enhance our comprehension of the physical world cannot be overstated. Users of these tools must approach their applications with curiosity and a willingness to innovate, ensuring that the future of research continues to benefit from their insightful capabilities.