Exploring Methane Bubbles: Formation and Impact
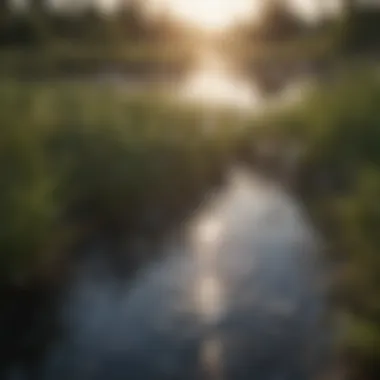
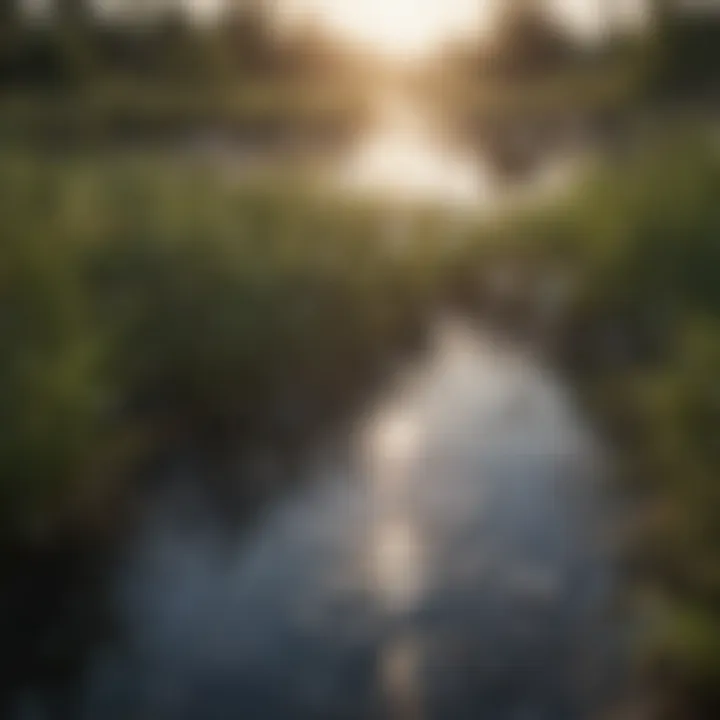
Intro
Methane bubbles, both in aquatic and terrestrial contexts, represent a fascinating area of study within environmental science. These ephemeral phenomena arise through a variety of processes, including microbial activity and hydrocarbon deposits. Understanding the formation of methane bubbles is more than an academic exercise; it ties directly into broader environmental issues such as climate change and ecosystem health.
The formation of these bubbles occurs in diverse environments. In aquatic settings, anaerobic digestion occurs in wetlands and oceans, producing methane. Similarly, in terrestrial environments, permafrost thawing leads to the release of methane accumulated over thousands of years. The ways in which these bubbles form have significant implications for greenhouse gas emissions, which contribute to global warming.
Environmental scientists increasingly recognize the impact of methane bubbles on climate dynamics. As potent greenhouse gases, these bubbles influence atmospheric composition and, consequently, climate patterns. Moreover, the interaction of methane with other chemical components in the environment is a critical area of exploration.
In this article, various facets of methane bubbles will be discussed, including their formation mechanisms, environmental impacts, and future research directions. The interplay between methane emissions and climate policy will also be examined, providing readers with insights into how this aspect of environmental science might influence regulations and public awareness.
Understanding Methane Bubbles
Understanding methane bubbles is essential for comprehending the complex interactions within our ecosystems. These bubbles, primarily composed of methane gas, emerge through various natural processes. Their formation and presence significantly contribute to discussions about climate change, environmental health, and greenhouse gas emissions.
The study of methane bubbles offers important insights for researchers analyzing the role of this potent greenhouse gas. Learning how these bubbles form helps in year-round monitoring of methane contributions to the atmosphere. Methane is known to be much more effective than carbon dioxide at trapping heat in the atmosphere, making its study crucial to understanding global warming.
This section sets the stage for a comprehensive exploration of methane bubbles, diving into their definitions, characteristics, and chemical composition. Understanding these aspects is key for evaluating the broader implications for both terrestrial and aquatic environments.
Definition and Characteristics
Methane bubbles are pockets of gas that form within sediments in various environments, like wetlands, ocean floors, or even in permafrost layers. These bubbles can vary in size, ranging from mere millimeters to significant collections spanning several meters in diameter.
One defining characteristic of methane bubbles is their ability to ascend through water or sediment. When they rise, bubbles can release methane into the atmosphere or water column, creating potential impacts in both areas. The physical formation of these bubbles can be affected by pressures and temperatures of their surrounding environments, leading to various sizes and concentrations.
Chemical Composition of Methane
Methane, a simple chemical compound, is composed of one carbon atom and four hydrogen atoms, represented as CHโ. This composition makes it a relatively lightweight gas, contributing to its buoyancy and tendency to rise through water and sediment layers.
Interestingly, methane can be produced from both biogenic and thermogenic sources. This simple structure allows it to play a role in various biochemical processes, such as anaerobic digestion carried out by microorganisms. Understanding methane's chemical properties enables researchers to analyze its stability and interactions with other compounds in the environment, further illustrating its environmental significance.
Methane's potency as a greenhouse gas, combined with its various pathways of production, underscores the need for rigorous study in multiple environments.
Overall, understanding the definition, characteristics, and chemical composition of methane bubbles lays the groundwork for exploring their origins and implications fully. This knowledge is vital for the future discussions on climate change and ecological health.
Formation of Methane Bubbles
The formation of methane bubbles is central to understanding their implications on the environment. These bubbles not only signify the presence of methane but also underscore its influence on climate change and ecological systems. As methane is a potent greenhouse gas, knowing how and where it forms helps in assessing its impact on global warming. Moreover, studying the formation processes can reveal interactions between biological and geological mechanisms that contribute to methane emissions, making this a crucial area of focus.
Biogenic Sources
Microbial Processes
Microbial processes are a significant contributor to methane formation. Microorganisms in anaerobic conditions break down organic material, resulting in methane production. This process, known as methanogenesis, highlights a key characteristic of biogenic sources: their reliance on biological activity. Since it primarily occurs in natural settings such as wetlands and digestive tracts of animals, it is crucial for understanding methane's role in the carbon cycle.
A unique feature of microbial processes is their capacity to trap carbon in organic forms before releasing it as methane, which may be advantageous in maintaining ecosystem balance. However, they can also lead to detrimental greenhouse gas emissions when the release of methane is not managed.
Anaerobic Environments
Anaerobic environments play a vital role in methane production. These include wetlands, rice paddies, and landfills, where oxygen is scarce. The absence of oxygen creates ideal conditions for microbial processes that generate methane. This characteristic makes anaerobic environments a beneficial focus for this article.
A unique feature is that these regions often act as both sources and sinks of greenhouse gases, influencing overall carbon dynamics. Their contribution to methane emissions is significant, but they also offer opportunities for carbon sequestration, which can be seen as an advantage in climate strategies.
Thermogenic Sources
Geological Processes
Geological processes refer to the natural mechanisms that lead to methane formation deep within the Earthโs crust. These processes involve the thermal decomposition of complex hydrocarbons, and highlight the relevance of geological time scales in understanding methane generation. This key characteristic provides insight into how much methane is locked within geological formations and how it can be released over time.
The unique aspect of geological processes lies in their ability to contribute to large-scale methane reserves, such as those found in shale formations or undersea sediments. However, these processes often take longer to understand and quantify, presenting challenges in real-time monitoring and management of methane emissions.
Natural Gas Reservoirs
Natural gas reservoirs also represent a crucial thermogenic source of methane. These reservoirs comprise large quantities of methane trapped within geological formations, typically resulting from millions of years of organic material degradation under high pressure and temperature. One key characteristic is that these reservoirs can be economically tapped for energy, making them a significant focus for energy policy discussions.
A unique feature of natural gas reservoirs is their ability to emit methane as unevenly pressure changes occur. This can lead to accidental releases into the atmosphere, posing a risk for climate impact. The balance between energy needs and environmental concern makes understanding these reservoirs essential in formulating both industrial practices and mitigation strategies.
Methane Bubbles in Aquatic Systems
Understanding methane bubbles in aquatic systems is essential for assessing their role in environmental health. They impact both freshwater and marine environments, affecting water quality and ecosystem dynamics. Their presence can indicate various biogeochemical processes and serve as a biophysical therapist for aquatic systems. By closely examining these bubbles, we can gain insights into broader ecological implications and climate change.
Freshwater Ecosystems
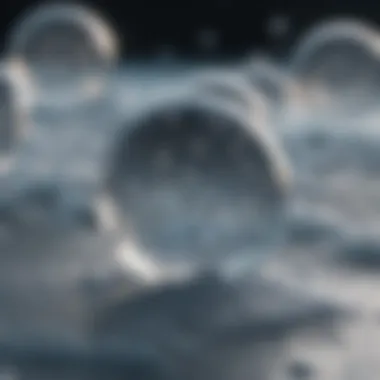
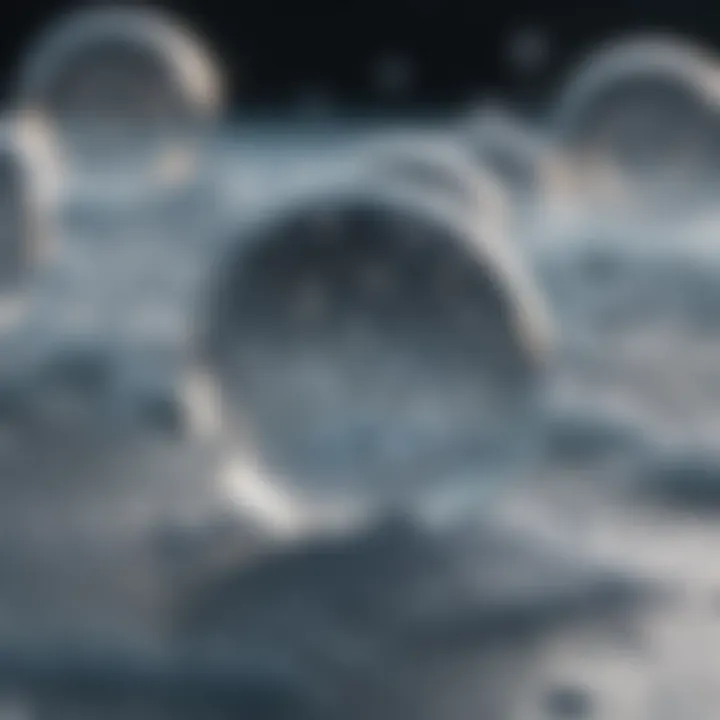
Impact on Water Quality
Methane bubbles significantly influence water quality, creating a complex interplay between chemical constituents. When these bubbles escape from the sediment, they can alter dissolved gas concentrations. This factor often leads to fluctuations in oxygen levels, affecting aquatic biota. A notable characteristic is that increased methane concentrations can lead to conditions such as hypoxia. This state generates stress for fish and other aquatic organisms, resulting in potential shifts in population dynamics. Thus, discussing water quality in relation to methane bubbles provides essential insights into ecosystem health.
Advantages of analyzing the impact on water quality include a better understanding of the mechanisms driving these interactions. Monitoring methane levels can also enable researchers to predict shifts in productivity and biodiversity in freshwater ecosystems.
Ecosystem Interactions
Ecosystem interactions are vital in understanding how methane bubbles fit into the larger freshwater ecosystem. These interactions include nutrient cycling, predation, and symbiosis. A compelling aspect involves the way methane can serve as an energy source for certain microorganisms, facilitating a unique microbial community. This means the presence of methane bubbles can directly affect food webs and the resilience of freshwater ecosystems.
Highlighting ecosystem interactions allows us to recognize the broader implications for aquatic life. On one side, the unique contributions of these bubbles can enhance biodiversity by promoting various microbial processes. However, potential disadvantages include destabilizing native species, leading to dominance by opportunistic forms.
Marine Environments
Oceanic Methane Seeps
Oceanic methane seeps are significant features in marine environments. These seeps act as natural conduits for methane to escape into ocean waters, influencing ocean chemistry. A critical aspect of these seeps is that they provide an environment for unique biological communities, such as chemosynthetic bacteria that utilize methane for energy. This unique feature supports the idea that these seeps create localized hotspots of productivity in otherwise nutrient-poor areas.
Understanding oceanic methane seeps has implications for marine ecosystems. The presence of methane can enhance local biodiversity by creating new niches for specialized organisms. However, unacceptable conditions may arise if methane concentrations become too high, potentially leading to negative impacts on surrounding habitats.
Effect on Marine Life
The effect of methane on marine life is a topic of ongoing research. Methane bubbles can influence various forms of marine life, from plankton to larger species. A key characteristic of this interaction is the potential for methane to disrupt normal biological activities and processes. For instance, alterations in methane levels might affect reproductive cycles in some fish species, influencing population dynamics.
Highlighting the effects on marine life emphasizes the vulnerability of the ecosystem to changes in methane concentrations. The increase in methane can foster thriving microbial communities, but this dynamic can also lead to conditions unfavorable for many marine species. Understanding these interactions is critical for developing effective management strategies to mitigate potential negative impacts.
Methane Bubbles in Terrestrial Environments
Methane bubbles in terrestrial environments are significant in understanding the interplay between methane production and ecological dynamics. The unique conditions of wetlands, peatlands, and even agricultural soil contribute to methane emissions. These environments are focal points for both methane generation and consumption, making them a critical component in carbon cycling. Their role in global warming and environmental health cannot be overstated.
Wetlands and Peatlands
Wetlands and peatlands serve as essential environments for methane production. They are characterized by waterlogged conditions that allow anaerobic microbial processes to thrive. This fosters the production of methane, contributing to the overall greenhouse gas emissions in the atmosphere.
Role in Carbon Cycling
The role of wetlands and peatlands in carbon cycling is crucial. They act as carbon sinks, storing significant amounts of organic carbon. However, when these areas are disturbed, the stored carbon can be released in the form of methane. The key characteristic of these environments is their high organic matter content and low decomposition rate. This makes wetlands a beneficial choice for understanding methane emissions. However, the delicate balance can be disturbed by human activity, leading to increased methane release. The unique feature of carbon cycling in these areas lies in their potential to either mitigate or exacerbate climate change, depending on land use and environmental management practices.
Biodiversity Factors
Biodiversity plays a vital role in the functioning of wetlands and peatlands. The various organisms present contribute to the stability of these ecosystems. Their interactions can influence the amount of methane produced. The key characteristic here is that a diverse ecosystem is generally more resilient to changes, leading to lower methane emissions. This makes it a popular choice for studies on methane impact and mitigation. However, the loss of biodiversity can disrupt these systems, resulting in increased emissions. The unique feature of biodiversity factors in this context is their direct correlation to methane dynamics, highlighting the necessity of preserving these ecosystems.
Soil Methanogenesis
Soil methanogenesis involves the production of methane in soil, mainly by microbes during anaerobic conditions. This process contributes significantly to methane emissions, particularly in agricultural lands where certain practices create favorable conditions for production.
Microbial Dynamics
Microbial dynamics in soil are crucial for understanding methane generation. Certain bacteria thrive in anaerobic conditions and break down organic matter to produce methane. This characteristic is essential as it shows the biological processes that drive emissions. By studying these dynamics, one gains insights into potential mitigation strategies. The unique feature of microbial populations is their sensitivity to environmental changes. Changes in temperature or moisture can significantly affect their activity and, consequently, the amount of methane produced.
Agricultural Implications
The implications of methane production in agricultural contexts are significant. Agricultural practices such as flooded rice fields or manure management can create conditions conducive to methane production. The key characteristic of these practices is that they can lead to considerable methane emissions. By addressing them, agriculture can play a pivotal role in climate change mitigation. A unique feature of agricultural implications is their dual nature; while agriculture can contribute to emissions, it also has the potential for innovative practices that reduce methane, such as improved land management and use of cover crops.
Emphasizing the role of wetlands, peatlands, and agricultural practices can lead to better understanding and management of methane emissions, hence benefitting climate strategies.
Environmental and Climate Impact
The environmental and climate impact of methane bubbles is a critical focus within the broader debate on climate change. Methane serves as a potent greenhouse gas, being significantly more effective at trapping heat compared to carbon dioxide over a short period. Understanding this aspect is essential for grasping the role methane bubbles play in global warming, which ultimately influences environmental policies and mitigation strategies.
Greenhouse Gas Emissions
Effects of Methane on Global Warming
Methane contributes substantially to global warming. Its ability to trap heat in the atmosphere is much greater than that of carbon dioxide over a 20-year period. This characteristic makes methane a key player in discussions surrounding climate change mitigation. However, its relatively short atmospheric lifespan means that immediate reductions in methane emissions could lead to rapid changes in warming potential. The unique feature of methane's potency allows for targeted policies that focus on short-term climate benefits.
- The following elements highlight this dynamic:
- Methane is over 25 times more effective than carbon dioxide at absorbing heat over a 100-year period.
- Reducing methane emissions can yield immediate climate benefits.
- Its concentration in the atmosphere has rapidly increased since the industrial revolution.
Comparative Analysis with CO2
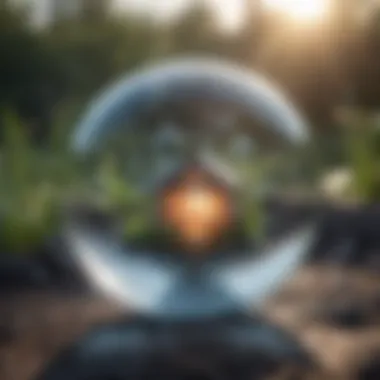
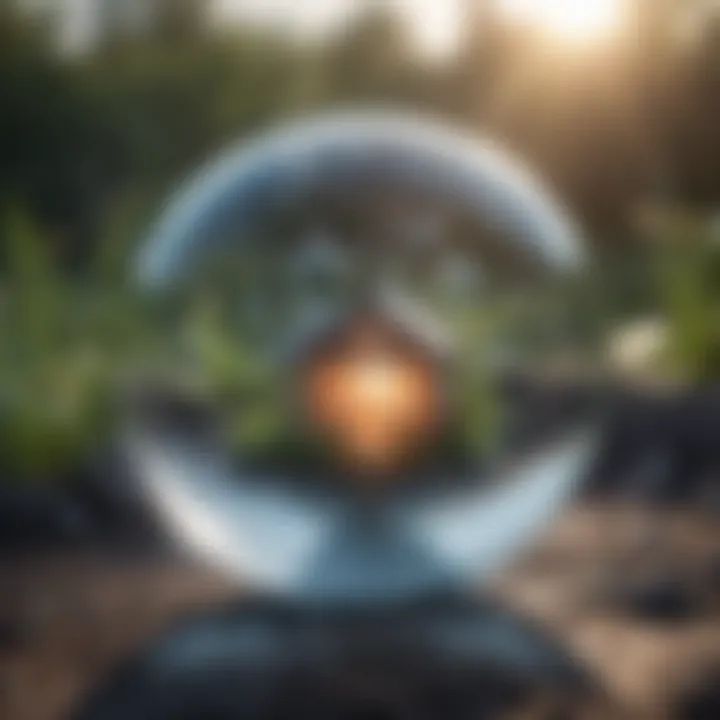
Comparing methane to carbon dioxide illuminates their different roles in climate science. While carbon dioxide is a significant long-term contributor, methane's immediate effects on warming create urgency in regulating its emissions. This comparative analysis reveals how targeting methane could complement broader carbon reduction strategies.
- The distinct features of this comparison include:
- Methane stays in the atmosphere for a shorter time, which can lead to faster climate outcomes when reduced.
- Its increase in emissions is often linked to specific activities such as agriculture and fossil fuel extraction.
- Policy discussions can benefit from this understanding, enabling targeted interventions.
Ecosystem Dynamics
Disruption of Ecological Balance
Methane bubbles can lead to significant disruption of ecological balance. As methane escapes into the atmosphere, it alters the natural interactions among various species and ecosystems. This disruption can threaten certain organisms while providing advantages to others, thus changing the community structure.
The implications of such disruptions extend far beyond the immediate environment, influencing carbon cycling processes and enhancing feedback loops in climate systems.
- Key aspects to consider:
- Changes in species interactions can lead to biodiversity loss.
- The alteration of nutrient cycling may affect local agriculture and food systems.
Implications for Biodiversity
The implications for biodiversity are profound. As ecosystems adjust to increased levels of methane and the resulting climate effects, many species may find it difficult to adapt. This can lead to shifts in species composition and potentially the extinction of sensitive species. Understanding these implications is crucial for conserving biodiversity and ensures that conservation strategies are well informed.
- Notable points regarding biodiversity impact include:
- Many species are already stressed by climate change, making them more susceptible to additional pressures from methane-related shifts.
- Protecting habitats can help maintain biodiversity, but effective approaches require recognizing methane's role in ecosystems.
In summary, the environmental and climate impacts of methane bubbles are complex yet vital areas of study. Their role as a greenhouse gas and their influence on ecosystems underline the need for comprehensive research and policy solutions aimed at mitigating climate change.
Research Methodologies
The study of methane bubbles involves complex processes and various conditions that necessitate a robust approach to research methodologies. Understanding how methane bubbles form and their environmental impacts requires precise techniques and effective data gathering. Each methodology provides unique insights, supporting a comprehensive analysis of methane's role in climate change and ecological dynamics.
Field Studies
Field studies are essential in examining methane bubbles in situ. They involve direct observation and sampling from natural environments where methane is generated or released.
Sampling Techniques
The sampling techniques employed in field studies significantly influence the quality of the data gathered. One key characteristic is the ability to collect samples from diverse environments, including freshwater and marine systems. This flexibility allows researchers to assess variations in methane concentrations across different geological and ecological contexts.
The most beneficial choice in sampling techniques is often the use of portable gas analyzers. These instruments enable researchers to perform real-time monitoring of methane emissions in various settings. A unique feature of these analyzers is their capacity to integrate multiple environmental parameters, enhancing the context for gas measurements. However, there are disadvantages as well, including costs associated, which may limit access in some studies.
Data Collection Methods
Data collection methods are crucial for capturing the dynamic behavior of methane bubbles. Effective methods range from in situ measurements to controlled sampling techniques. These methods focus on accurately recording methane emissions and understanding the ecological influences at play.
One key characteristic of successful data collection is the utilization of continuous monitoring systems. This ensures that fluctuations in methane levels are recorded over an extended period, providing a clearer picture of trends. The unique feature here is the potential for remote data transmission, which allows real-time data analysis without needing immediate presence in the field. However, this approach requires careful calibration of instruments, which can be a complex and time-consuming process.
Laboratory Analyses
Laboratory analyses play a pivotal role in validating field data and understanding the chemical properties of methane. These methods allow researchers to conduct controlled experiments, leading to deeper insights into methane's behavior and environmental role.
Instrumental Techniques
The instrumental techniques utilized in laboratory analyses are vital for detailed examination of methane. Common techniques include gas chromatography and mass spectrometry. One key advantage of these methods is their high sensitivity, enabling detection of trace amounts of methane in samples. This sensitivity is crucial for studying methane in various environmental contexts.
A unique feature of gas chromatography is its ability to separate different components within a gas sample. This allows researchers to assess the composition of methane and its potential precursors accurately. However, disadvantages include the need for specialized training and equipment, which may not be feasible for all research teams.
Experimental Setups
Experimental setups are designed to replicate conditions necessary for methane formation and emission. These carefully controlled environments help in simulating various factors affecting methane production.
One key characteristic of well-designed experimental setups is the ability to manipulate variables, such as temperature and pressure. This control enables researchers to draw more accurate conclusions about the factors influencing methane emissions. The beneficial choice for many studies is the utilization of large-scale mesocosms, which can mimic natural ecosystems in a controlled environment.
However, the unique feature of these setups is the potential for inconsistencies between the artificial environment and the natural world. This can lead to challenges in translating findings directly back to real-world scenarios, creating a gap that researchers must address in their analyses.
Current Findings in Methane Research
The topic of current findings in methane research is crucial for understanding the broader implications of methane emissions on climate change. Recent studies highlight critical trends and advancements in how methane is detected and measured. This section explores these emerging patterns, which can help develop effective strategies for mitigating methane's impact on our environment.
Trends in Methane Emission
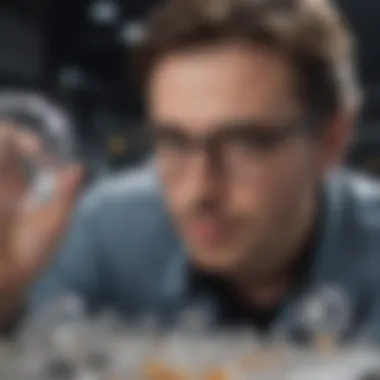
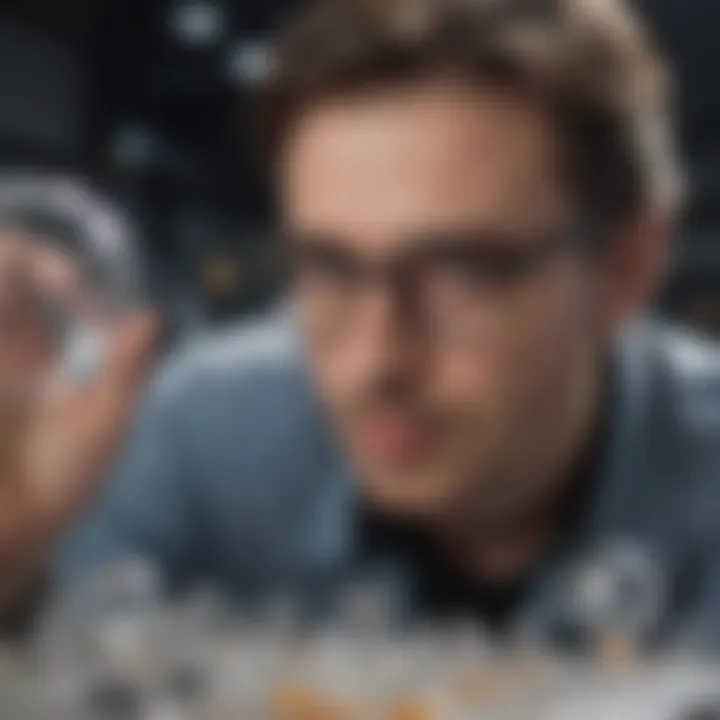
Geographic Variability
Geographic variability refers to the differences in methane emissions across different locations. This aspect is important due to its insights into localized sources of methane. Understanding where emissions are highest allows for targeted interventions that could reduce overall greenhouse gas emissions. Areas like wetlands, rice paddies, and landfills tend to have varied levels of methane production, influenced by both natural and human factors. This makes it a beneficial focus in the study as it highlights the need for localized data collection and management.
Unique features of geographic variability include its dependence on climate, biogeochemical cycles, and land use patterns. Such variability can be advantageous in modeling methane distribution and developing localized policies. However, one disadvantage lies in the data disparity; some regions may have extensive research, while others remain under-studied, creating gaps in understanding.
Influencing Factors
The influencing factors of methane emissions are myriad, ranging from agricultural practices to geological settings. This highlights the complexity involved in emissions modeling and offers insights into how human activity affects methane levels. The key characteristic of influencing factors is their varied nature; they can be both natural, such as temperature changes, and anthropogenic, such as livestock management.
In the context of this article, understanding these influencing factors is beneficial as it allows us to identify key contributors to methane emissions. Unique features might include specific agricultural practices or land use changes, each having distinct effects on emissions levels. The advantages of identifying these factors include developing informed policy choices that target emission reductions. However, the disadvantage is the challenge of integrating diverse datasets to paint a complete picture.
Innovative Detection Techniques
Remote Sensing
Remote sensing involves the use of satellite technology to monitor methane concentrations. This method has become crucial in methane research due to its ability to provide extensive data over large areas. The key characteristic of remote sensing is its non-invasive approach, which allows for real-time monitoring without disrupting ecosystems.
For this article, remote sensing is particularly valuable because it facilitates the identification of methane hotspots across the globe. The unique feature is its capability to detect methane plumes from afar, offering a broad perspective. Advantages include its ability to cover remote and inaccessible regions. A disadvantage, however, is the potential for difficulties in distinguishing methane from other gases, which may lead to data inaccuracies.
Satellite Observations
Satellite observations provide another avenue for tracking methane emissions. Similar to remote sensing, this technique allows for monitoring atmospheric methane levels from space. The key characteristic is the advanced sensing technology used on satellites, which can capture high-resolution data.
This makes satellite observations an essential tool in current methane research. The unique feature is the combination of wide coverage and detailed data collection. Advantages include the ability to gather data globally and longitudinally, aiding in trend analysis. However, the disadvantage lies in the costs and expertise required to properly interpret the data collected.
The integration of geographic variability and innovative detection techniques is essential for developing actionable solutions to mitigate methane emissions and their effects on climate change.
Global Warming and Mitigation Strategies
The link between methane bubbles and global warming is critical. Methane, as a potent greenhouse gas, has a significant role in the climate change narrative. The contribution of methane to warming is far greater than that of carbon dioxide over a short-term span. Understanding the stresses that methane bubbles put on our environment is essential for framing effective mitigation strategies. This section outlines the various approaches to reduce methane emissions, emphasizing both policy frameworks and technological innovations that can support sustainable environmental health.
Mitigation Approaches
Policy Frameworks
Policy frameworks are essential tools in our efforts to mitigate methane emissions. They create structured guidelines for governments and agencies, ensuring a coordinated approach to reduce overall emissions. One significant characteristic of these frameworks is their ability to enforce compliance among industries known for high methane output, including agriculture and fossil fuels. This regulatory power makes them a popular choice within climate policy discussions.
A unique feature that sets effective policy frameworks apart is their adaptability. They can evolve as new research emerges, allowing for improvements based on the latest scientific evidence. The advantages of robust policy frameworks include enhanced accountability and measurable results, while potential disadvantages could involve economic impacts on industries needing compliance adjustments.
Technological Innovations
Technological innovations present another avenue for tackling methane emissions. They involve the development of advanced methods and tools aimed at detecting and reducing methane release. A key characteristic of these innovations is their ability to provide real-time monitoring. This is crucial for identifying leaks and implementing corrective measures promptly.
These innovations are beneficial as they can help industries optimize operations, ensuring minimal emissions. However, the unique feature of these technologies is their high initial cost and the ongoing need for maintenance and updates. This can be a considerable drawback for smaller organizations looking to implement such solutions.
Future Research Directions
Knowledge Gaps
Identifying knowledge gaps is fundamental in the continuing research of methane emissions and their effects. This aspect focuses on areas where scientific understanding is limited, such as the long-term effects of methane on specific ecosystems. Recognizing these gaps can guide future studies and is crucial for designing efficient policies and mitigation strategies.
One key characteristic of focusing on knowledge gaps is that it helps target research funding to areas that will yield the most impactful results. However, the disadvantage is that chasing these gaps can lead to fragmented research approaches if not managed adequately.
Interdisciplinary Collaboration
Interdisciplinary collaboration is vital in enhancing research quality regarding methane. This collaboration typically involves experts from various fields, such as climatology, ecology, and technology. The key characteristic of this approach is the diverse perspectives and expertise it brings, leading to more comprehensive solutions.
Such collaboration is beneficial as it allows for innovation to flourish, leading to more effective strategies for methane mitigation. However, the unique challenge of interdisciplinary work lies in integrating different methodologies and terminologies, which can slow down the research progress if not well coordinated.
Epilogue and Implications
The examination of methane bubbles brings to light not just their intriguing formation and characteristics, but also their significant role in shaping our understanding of environmental science. This article has navigated through the complexities surrounding the biogenic and thermogenic sources of methane, the unique ecosystems affected by these bubbles, and the broader environmental impacts they pose. The implications of methane bubbles extend far beyond mere curiosity; they challenge existing paradigms in climate science and signal a need for comprehensive mitigation strategies.
Integrative Understanding of Methane Bubbles
An integrative approach to understanding methane bubbles requires the amalgamation of various scientific disciplines. These bubbles serve as indicators of biological processes and geological formations. Their presence in wetland ecosystems points towards microbial activity while in marine environments, they highlight intricate geological interactions. Therefore, addressing how methane bubbles impact these ecosystems can unveil larger trends in global warming. Key areas of focus include:
- Formation Mechanisms: Understanding how and why methane bubbles form is critical. Exploring both microbial processes in organic matter decomposition and geological factors can clarify their origins and implications.
- Emission Levels: Regular monitoring of methane emission rates helps establish baseline data crucial for climate modeling. This data is essential as methane is a potent greenhouse gas.
- Ecosystem Interactions: The balance within ecosystems can be disrupted by methane release, affecting biodiversity. Integrating research concerning ecosystem dynamics and methane production is paramount.
This integrated understanding is vital for crafting informed policies and predicting future behaviors of methane-related systems in the context of climate change.
Broader Environmental Implications
Methane bubbles captivate attention due to their immediate environmental ramifications and their long-term implications for global climate patterns. One significant aspect is their contribution to greenhouse gas emissions. Methane is many times more effective at trapping heat in the atmosphere compared to carbon dioxide over a short time frame. Hence, the release of methane from natural sources impacts global warming considerably. Key implications include:
- Climate Change Acceleration: Understanding methane bubbles is critical in formulating climate action strategies. Their release signifies larger environmental shifts that necessitate urgent scientific and policy-based responses.
- Biodiversity Risks: The influence of methane on ecosystem dynamics raises concerns regarding species extinction and habitat loss. Particular attention to the feedback mechanisms involved can provide insights into broader ecological health.
- Public Policy Impact: Scientific insights from methane research must translate into effective public policies. These should include regulations on methane emissions and incentives for research into alternative technologies that mitigate this gas's release.
The exploration of methane holds the keys to understanding and combating one of the most pressing issues of our time: climate change.