Exploring the Role of mRNA Codons in Gene Expression
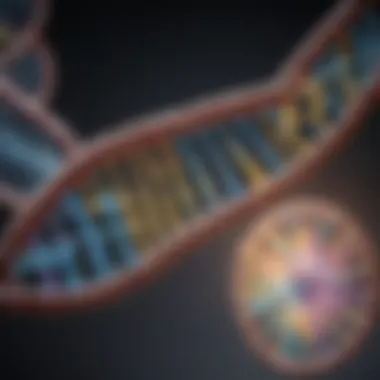
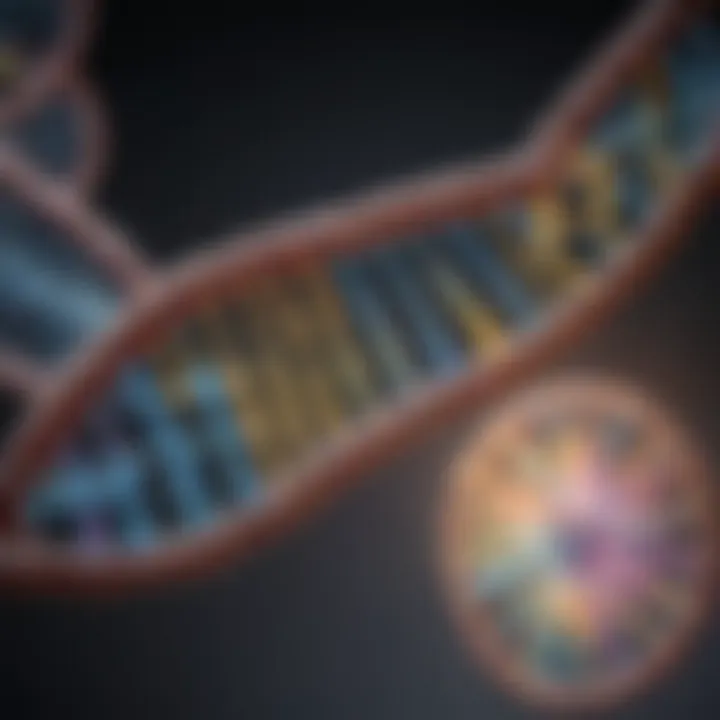
Intro
Understanding complementary mRNA codons is crucial for any serious student of molecular biology. They are not just random sequences of letters but are building blocks of life itself. Each codon plays a significant role in translating genetic information into proteins. This article takes you on a journey through the complexities of mRNA codons, detailing their importance in gene expression and laying the groundwork for further explorations into the field.
Recent Advances
In recent years, the field of molecular biology has seen substantial breakthroughs regarding mRNA codons and their complementary relationships. As scientists tirelessly research these relationships, they've made notable strides in both discovery and technological innovation.
Latest Discoveries
Recent research has brought intriguing new insights into how codon pairing impacts gene expression. For instance, a study published in a leading journal revealed that certain codons can influence the efficiency of protein synthesis significantly. These findings have prompted scientists to reconsider how they approach genetic coding. The ongoing research in this area continues to change the landscape of genetic studies.
"The relationship between mRNA codons and their corresponding amino acids is more intricate than previously thought."
Technological Innovations
Advancements in genomics also play a key role. High-throughput sequencing technologies, such as Illumina sequencing, have revolutionized how researchers can analyze codon sequences. The ability to quickly gather vast amounts of data has led to more intricate analyses and a deeper understanding of how codons interact in various biological contexts.
Another innovative technique involves CRISPR gene-editing tools that allow for precise alterations in codon sequences. Such tools not only provide insights into genetic functions but also hold promise for therapeutic applications.
Methodology
Understanding the methodologies behind researching mRNA codons can unveil a lot about how findings are reached. As science embraces new approaches, it's essential to appreciate the framework underpinning this research.
Research Design
Most studies begin with a clear hypothesis regarding codon interactions. Researchers typically employ comparative genomic studies, analyzing codon usage frequencies across different organisms. This comparative approach can help identify patterns that are consistent across species, suggesting fundamental biological principles.
Data Collection Techniques
To gather data, researchers utilize a combination of bioinformatics tools and laboratory techniques. For instance, RNA sequencing is pivotal for obtaining high-fidelity data on mRNA codons. Once the sequences are collected, bioinformatics software helps to align and analyze them, revealing relationships that might not be readily apparent through traditional methods. The integration of statistical models further aids in confirming these relationships.
By employing diverse research designs and data collection methods, scientists not only enhance their understanding of complementary mRNA codons but also ultimately advance their applications in various fields of biotechnology and genetic engineering. As research continues to develop in this exciting area, staying abreast of the findings will be crucial for anyone keen on exploring the depths of molecular biology.
Preamble to Codons
Understanding codons is fundamental to grasping how genetic information is translated into functional proteins. Codons, essentially, are sequences of three nucleotides found within mRNA that direct the synthesis of amino acids, the building blocks of proteins. This section will dive into the significance of codons in molecular biology, laying the groundwork for comprehending complementary mRNA codons.
The health of biological functions often hinges on these little triplet sequences. For someone delving into genetics, it’s akin to knowing the chords before strumming the guitar; it's the basis of musical expression that is protein synthesis. In a world where synthetic biology and gene editing have captured public interest, codon understanding becomes even more pronounced.
Defining Codons in Genetics
Codons serve as a translator of sorts, converting the information stored in DNA into proteins. In essence, a codon is a three-nucleotide-long nucleotide sequence within mRNA, which corresponds to a specific amino acid or a termination signal during protein synthesis. In the genetic code, there are 64 possible codons, yet only 20 standard amino acids to decode, leading to some amino acids being represented by multiple codons. This phenomenon is known as redundancy of the genetic code, which might appear as a safety net for proteins, helping to mitigate the risk associated with mutations.
Importance of Understanding Codons
- Gene Expression: Codons play a pivotal role in the overall process of gene expression. They determine the sequence of amino acids in a protein, affecting its structure and function.
- Mutation Implications: Misreading a codon due to mutation can lead to malfunctioning proteins. Knowing codons helps in predicting these changes and their effects on the organism.
- Biotechnology Advances: In fields like synthetic biology, the design of genetic constructs often relies on precise codon usage to optimize the expression of desired traits.
The Codon Structure
The codon structure is relatively straightforward but powerful. Each codon is a triplet of nucleotides, comprising adenine (A), cytosine (C), guanine (G), or uracil (U) in RNA. This structural simplicity belies their essential role in specifying the amino acid sequences of proteins. Each nucleotide functions as a letter in a genetic alphabet, allowing for a multitude of combinations to convey specific biological information.
Key Features of Codon Structure:
- Triplet Nature: The necessity of three nucleotides for a single codon means that the combinations facilitate a vast array of possible signals.
- Start and Stop Signals: Some codons are special; the AUG codon not only codes for methionine but also serves as a start signal. Conversely, UAA, UAG, and UGA function as stop signals, halting protein synthesis, underscoring the codon’s dual role in protein construction.
- Directionality: Codons are read in a 5’ to 3’ direction, establishing an orientation that is critical for correct protein assembly during translation.
Understanding the codon structure lays the foundation for grasping how mRNA interacts with tRNA and ribosomes during translation, ultimately impacting protein synthesis. As we will explore in the next sections, codons not only dictate the biological code but also provide insights into genetic engineering, biotechnology, and evolutionary adaptations.
"Genetic information is stored in the sequence of nucleotides. Codons are the bridge that translate this information into functional proteins."
Whether you're studying genetics in academia or applying this knowledge in research, delving into codons enhances our collective understanding of life at its most fundamental level.
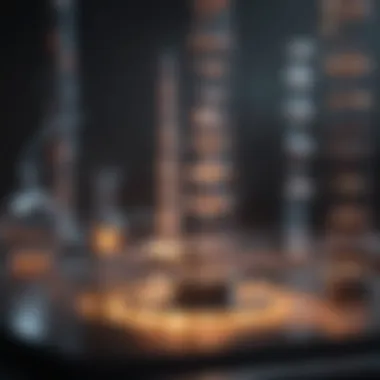
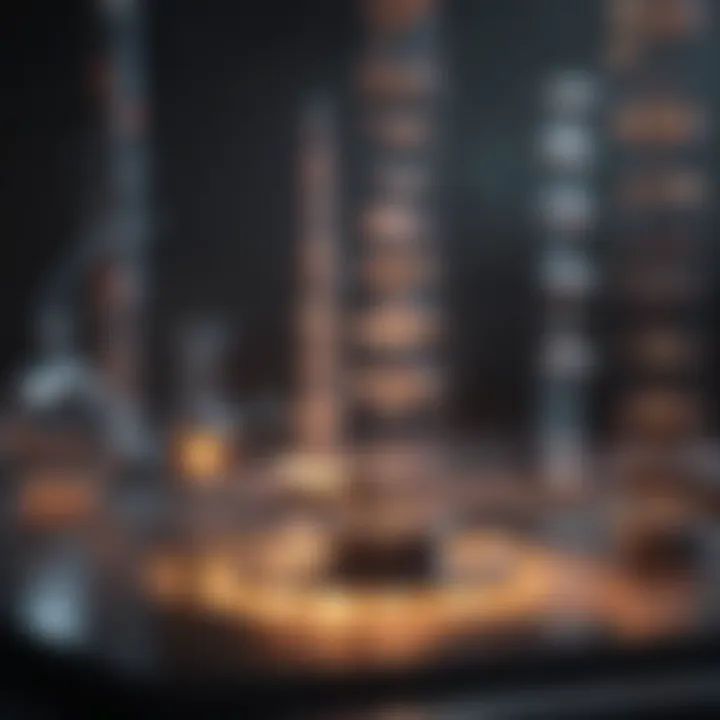
mRNA and Its Role in Protein Synthesis
The role of messenger RNA (mRNA) in the realm of protein synthesis is pivotal and fundamentally intertwined with genetic expression. Understanding this process is not just about memorizing terms; it’s about grasping how life’s code operates at a molecular level. mRNA serves as a bridge between the genetic blueprint embedded within DNA and the intricate machinery that constructs proteins. Without mRNA, the genetic message would never transition into action, leaving genes static and unexpressed.
When a gene is activated, transcription occurs, producing a strand of mRNA that mirrors the sequence of the corresponding DNA. This mRNA carries the necessary genetic information out of the cell nucleus and into the cytoplasm, where ribosomes await to read the codons and translate them into amino acids—the building blocks of proteins. This is the heart of molecular biology, showcasing the delicate choreography between various cellular components.
Key Benefits and Considerations of mRNA in Protein Synthesis:
- Information Transfer: mRNA provides a direct route for the genetic instructions encoded in DNA to reach the ribosomes. This ensures that the right proteins are synthesized at the right time.
- Regulatory Role: Beyond merely serving as a template, mRNA molecules can be selectively degraded or modified, allowing cells to regulate protein synthesis based on environmental cues or cellular needs.
- Therapeutic Implications: Advances in biotechnology, particularly the development of mRNA vaccines, have highlighted the potential of mRNA in medical therapies, underscoring the importance of understanding this molecule.
Understanding mRNA Transcription
Transcription is the initial process in which the genetic information from DNA is transcribed into mRNA. In the nuclei of eukaryotic cells, a complex interaction unfolds. Enzymes called RNA polymerases bind to a specific region of the gene known as the promoter. As the RNA polymerase advances, it unwinds the DNA strands while synthesizing a single strand of RNA that reflects the complementary base pairs of the gene.
During this process, certain elements influence transcription—promoters, enhancers, and transcription factors play crucial roles. Promoters are essential for initiating transcription, while enhancers can amplify transcription levels from a distance. Factors like cellular stress, nutrient availability, and developmental cues can all affect the efficiency and rate of mRNA synthesis.
Despite its intricacy, transcription leads to a mature mRNA strand ready for processing. This includes capping at the 5’ end, polyadenylation at the 3’ end, and splicing out introns. These modifications not only protect the mRNA from degradation but also prepare it for translation.
Translation Process: From mRNA to Protein
Once mRNA is synthesized, it embarks on its journey to the ribosome, where it undergoes translation. Ribosomes, the cellular machinery responsible for protein synthesis, interpret the sequence of codons in the mRNA. Each set of three nucleotides (a codon) corresponds to a specific amino acid, and this relationship is defined by the genetic code.
The process of translation involves several key steps:
- Initiation: The ribosome assembles around the mRNA. The start codon (AUG) signals the beginning of protein synthesis.
- Elongation: tRNA molecules, each carrying a specific amino acid, pair with corresponding codons on the mRNA. Peptide bonds are formed between amino acids as the ribosome moves along the mRNA strand, elongating the polypeptide chain.
- Termination: When the ribosome encounters a stop codon, the process concludes. The newly formed polypeptide is released, folding into a functional protein.
The translation process is not merely mechanical; it’s finely tuned and heavily regulated. Various factors, including the availability of tRNA and ribosomal proteins, contribute to the overall efficiency and fidelity of protein synthesis.
Understanding the interplay of mRNA transcription and translation offers crucial insights into how proteins are synthesized, which, in turn, influences cellular functions and organismal traits.
Complementary mRNA Codons Explained
Understanding complementary mRNA codons is crucial for grasping how genetic information translates into functional proteins. This concept isn’t just a trivial detail; it’s the backbone of molecular biology. The pairing of codons – sequences of nucleotides in mRNA – plays a pivotal role in the synthesis of proteins, which are the workhorses of the cell. Without proper codon complementarity, a cascade of issues may emerge, potentially leading to incorrectly synthesized proteins, malfunctioning cellular processes, or even disease.
Nature of Complementarity
When we delve into the nature of complementary mRNA codons, the focus naturally shifts to the precise way in which nucleotide sequences interact. Each codon, composed of three nucleotides, corresponds to specific amino acids during the process of translation. This triplet nature means that the mRNA codons must align perfectly with the corresponding anticodons found on the transfer RNA (tRNA) during protein synthesis.
Interestingly, the complementary relationship between codons and their anticodons reflects a delicate design in molecular biology. This interaction is not just a simple one-to-one match; rather, it utilizes specific base-pairing rules that dictate which nucleotides bind together. For instance, adenine pairs with uracil while guanine pairs with cytosine. These complementary pairs ensure a level of fidelity in translation, crucial for maintaining the integrity of genetic messaging, and underscores the elegance of genetic coding.
"The fidelity of codon-anticodon pairing is fundamental to the robustness of protein synthesis, ensuring that the intended amino acid sequence is accurately assembled."
Base Pairing Rules
The rules surrounding base pair matching are fundamental to understanding how codons pair with their complements. At a basic level, the four nucleotides—adenine (A), cytosine (C), guanine (G), and uracil (U)—have specific partners. Here’s a quick rundown:
- Adenine (A) pairs with Uracil (U)
- Cytosine (C) pairs with Guanine (G)
- Guanine (G) pairs with Cytosine (C)
- Uracil (U) pairs with Adenine (A)
This strict set of pairing rules forms the cornerstone of the genetic code. It dictates how information is read during translation, ensuring that the correct amino acids are assembled in a sequence that defines the protein’s final structure and function.
An accidental substitution or omission in a codon can lead to misreads or mispairings that might result in nonfunctional or harmful proteins. Such mistakes can unravel years of evolutionary adaptations, pointing to the delicate balance within cellular processes.
Decoding the Genetic Code
The quantifiable significance of decoding the genetic code cannot be overstated, particularly in the realm of molecular biology. This section embarks on an exploration of how understanding codons and their complementary relationships is central to comprehending the vast intricacies of gene expression and the synthesis of proteins. Codons are not mere sequences; they are the language that helps translate the blueprint of life encoded in DNA into functional proteins, fundamentally influencing biological functions.
Analyzing the codons involves understanding various concepts and elements. The genetic code is a set of rules by which information encoded in genetic material is translated into proteins. Consisting of sequences of nucleotides, codons dictate which amino acids are assembled into proteins, a process essential for all cellular functions. As such, the importance of decoding these codes is apparent; it informs numerous applications from agricultural improvements to medical therapies.
Key elements in understanding the genetic code include:
- Universality: The genetic code is largely universal across all living organism, suggesting a common evolutionary origin.
- Redundancy: Multiple codons can code for the same amino acid, which offers a buffer against mutations.
- Biological Precision: Errors in decoding the genetic code can have significant implications, leading to diseases and disorders.
Decoding not only facilitates advancements in science, but it also serves as a foundation upon which further discoveries are built, making it a critical topic in this article.
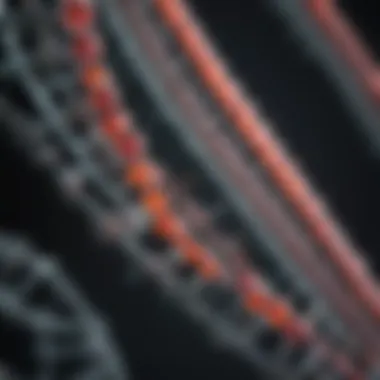
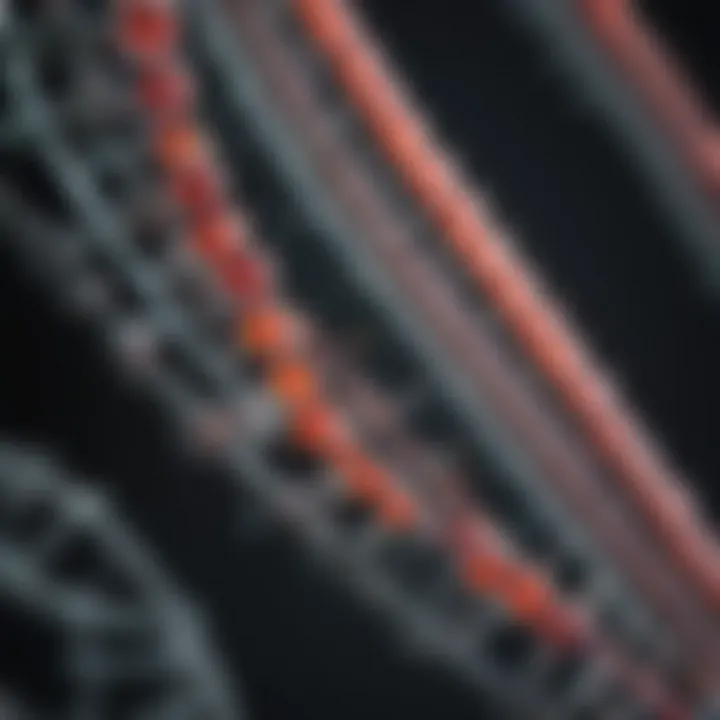
Codon Tables and Their Utility
Codon tables act as a guide for researchers, providing a systematic overview of the specific correspondence between nucleotide sequences and amino acids. Understanding this correspondence is crucial for anyone involved in genomic studies, protein engineering, or synthetic biology.
Codon tables are structured to reflect the relationships between the 64 possible codons and the 20 standard amino acids. To visualize this, consider the following aspects:
- Reference Tool: Codon tables serve as a quick reference. For instance, the codon AUG not only indicates the start of protein synthesis but also codes for Methionine.
- Error Checking: When analyzing genetic sequences, codon tables help check for anomalies or deviations from expected patterns, which could indicate mutations.
- Customization: Certain organisms may exhibit variations in their preferred codon usage, which can impact the efficiency of protein production. Thus, understanding these nuances becomes essential in genetic engineering efforts.
Furthermore, this Wikipedia page provides a comprehensive view of codons and amino acids and is an excellent starting point for deeper exploration.
Identifying Amino Acids from Codons
The process of identifying amino acids from codons lies at the heart of protein synthesis. Every codon in mRNA corresponds to a specific amino acid during translation, and understanding this relationship is pivotal for several scientific investigations. This relationship unfolds through a complex yet beautifully orchestrated mechanism known as translation.
- Decoding Process: Using transfer RNA (tRNA) molecules that match specific codons, the ribosome facilitates the binding of amino acids in the correct sequence. Each tRNA carries a corresponding amino acid; for example, the codon GAA is matched by tRNA carrying Glutamic acid.
- Implications for Research: Knowledge of how to decode these relationships allows for precision in protein design and therapeutics. Researchers can manipulate sequences to explore protein functions or develop novel proteins for medical applications.
- Technological Relevance: Bioinformatics tools help automate this process, employing algorithms to predict amino acid sequences based on input mRNA codons, thereby streamlining research workflows.
Understanding how to identify amino acids from codons has far-reaching implications, particularly for initiatives ranging from drug development to synthetic biology. This connection encapsulates why decoding the genetic code is not only a scientific pursuit but a pragmatic approach to addressing some of the modern world's pressing genetic challenges.
Technical Methods for Determining Codons
In the intricate world of molecular biology, the need for precision in determining codons cannot be overstated. Understanding the specific sequences of mRNA codons allows researchers to delve deeper into protein synthesis and gene expression. The importance of various techniques used in this domain serves as the backbone for many biotechnological applications. By leveraging advanced methodologies, scientists are equipped to explore and manipulate genetic codes more effectively.
Techniques in this arena often bridge theoretical knowledge with practical applications. They unravel the complexities of biological systems, allowing for innovative solutions in genetic engineering, medical research, and synthetic biology. The multi-faceted nature of these methods not only contributes to enhanced accuracy but also expands the horizon for discovery and application in genetic sciences.
Sequencing Techniques Overview
Sequencing techniques form the cornerstone of codon determination, offering a comprehensive look at genetic material. By analyzing sequences of nucleotide bases, researchers can accurately assess codon sequences within mRNA. Here are some key methods:
- Sanger Sequencing: Often regarded as the gold standard, this method involves replication of DNA fragments using specific chain-terminating nucleotides. By employing electrophoresis, researchers can visualize the sequences, providing a clear picture of coding regions.
- Next-Generation Sequencing (NGS): This revolutionary technology allows for massive parallel sequencing, enabling the quick analysis of entire genomes. With NGS, it becomes feasible to identify codons on a large scale, effectively broadening the scope of genetic research.
- Illumina Sequencing: This specific NGS technique employs reversible dye terminators, which enable the sequencing of multiple samples simultaneously while yielding high accuracy.
- Nanopore Sequencing: Utilizing nanoscale pores, this method enables real-time sequencing of long-chain nucleic acids. Its capacity to provide continuous data adds a new dimension to codon determination by allowing analysis of RNA directly without reverse transcription.
Each sequencing technique offers unique advantages and challenges, and choosing the right one often hinges on the specific goals of a research project.
Bioinformatics Tools for Codon Analysis
The digital age has transformed the capabilities of genetic analysis through powerful bioinformatics tools. These software applications facilitate the understanding of codon usage, mutations, and evolutionary relationships among species. Highlights among these tools include:
- BLAST (Basic Local Alignment Search Tool): This tool allows researchers to compare nucleotide sequences against large databases, identifying homologous sequences and providing insight into codon functionalities.
- Geneious: A comprehensive platform that integrates various methods for bioinformatics analysis, providing streamlined workflows for DNA, RNA, and protein analysis.
- Codon Usage Database: This invaluable resource compiles codon usage frequencies across different organisms, offering insights into adaptive evolution and facilitating gene design strategies.
- R and Bioconductor: These programming tools are quite popular for statistical analysis of biological data, enabling customized analysis of codon preferences and translation efficiency.
Such tools are essential for interpreting the results from sequencing and for drawing biologically relevant inferences from the data.
"Accurate codon determination is not just a process; it’s an essential step in understanding the language of life itself."
Applications of Codon Determination
Understanding complementary mRNA codons opens up a multitude of avenues in scientific research and application. The meticulous analysis and determination of these codons not only underscore the foundations of molecular biology but they also propel advancements in numerous fields. Essentially, codon determination acts like the Rosetta Stone of genetics, allowing the translation of genetic information into functional proteins that embody the essence of life itself.
When delving into these applications, three primary domains stand out: genetic engineering, synthetic biology, and medical research. Each has its own nuances and implications that speak volumes about the necessity of accurate codon determination.
Implications in Genetic Engineering
In genetic engineering, the right codons can mean the difference between success and failure in creating organisms with desired traits. By understanding codon usage bias—where different organims have different preferences for certain codons for the same amino acid—scientists can tailor genes for optimal expression in particular host cells.
For instance, when inserting a human gene into a bacterial vector, researchers must select the appropriate codons to prevent misfolding or incomplete translation. This careful selection process optimizes protein yield, ensuring that the end product is functional. In practice, this has resulted in innovations such as insulin production and genetically modified crops that are resistant to pests and diseases.
"Codon optimization not only enhances protein expression but also reduces production costs, accelerating the path to market."
In this light, the principles of codon determination are vital to developing robust genetic modifications and ensuring their success in real-world applications.
Role in Synthetic Biology
Synthetic biology takes a step further, using codon determination as a foundation for designing new biological parts or systems with functionalities that do not occur in nature. Through the manipulation of mRNA codons, scientists can construct synthetic pathways, utilizing organisms as living factories to produce complex compounds, including biofuels and pharmaceuticals.
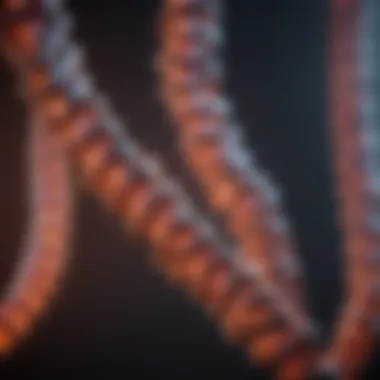
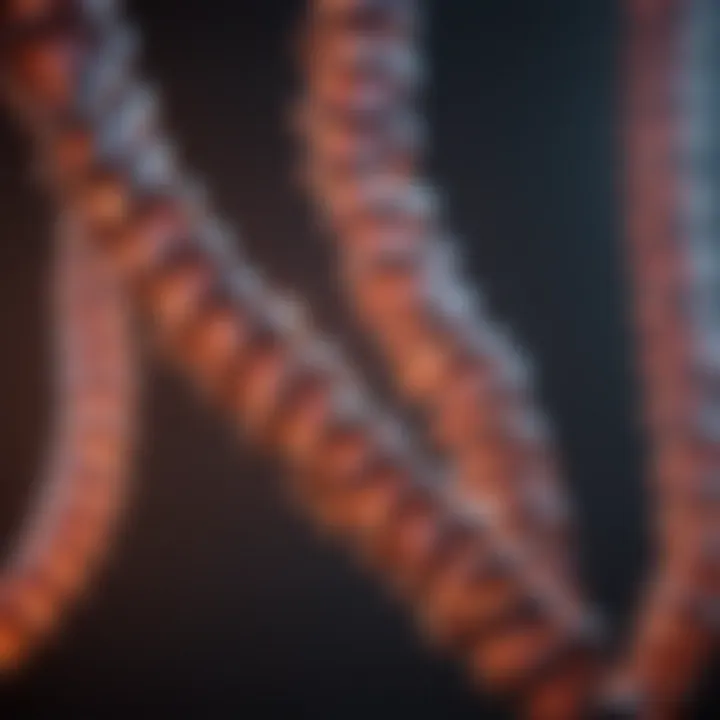
By employing algorithms to predict the best codon combinations, researchers can design for high-yield synthesis of metabolites that are crucial for energy or health. For instance, using engineered microbes and optimized codons can lead to the production of valuable enzymes for the biofuel industry, significantly reducing dependency on fossil fuels.
Contributions to Medical Research
In the realm of medicine, codon determination is indispensable, particularly in the development of gene therapies. As researchers strive to correct genetic disorders, understanding how specific codons influence the expression of therapeutic proteins in target cells becomes imperative.
Moreover, codon usage also figures prominently in vaccine development. For instance, during the COVID-19 pandemic, mRNA vaccines such as those produced by Pfizer and Moderna rely heavily on the precision of codon use. By ensuring that the codons for viral proteins are effectively translated, these vaccines can elicit a strong immune response, showcasing how molecular insights translate into ground-breaking health solutions.
In summary, as we explore the role of codon determination across various domains, it’s clear that its implications are vast, ranging from enhancing genetic engineering workflows to paving the way for innovations in synthetic biology and medical research. This area stands at the nexus of scientific exploration, proving crucial for the future landscape of genetic science.
Case Studies and Practical Examples
Understanding the complexities of complementary mRNA codons is not just academic; it has tangible implications in various scientific arenas. Case studies and practical examples provide a window into how these theories are applied in real-world situations. They serve as illuminating illustrations that bridge the gap between theory and practical application, allowing students and professionals alike to appreciate the relevance of codons in contemporary research and biotechnology.
In biotech and genetic engineering, knowing how to determine complementary mRNA codons can shape outcomes significantly. This understanding aids researchers in predicting how changes in the genetic code can impact protein synthesis. With growing concerns around genetic disorders and the pursuit of innovative therapies, the relevance of these case studies in practical applications become all the more paramount.
Real-World Applications in Biotechnology
The world of biotechnology offers a plethora of applications where complementary mRNA codons play a crucial role. Here’s where theory meets tangible benefits:
- Gene Therapy: Using complementary mRNA codons, scientists can design tailored therapies to correct genetic defects. This is essential in treating conditions like cystic fibrosis and muscular dystrophy.
- Vaccine Development: The success of mRNA vaccines, such as those used for COVID-19, rests partially on understanding codon pairings. The precise translation of mRNA into proteins ensures the immune system can recognize and combat pathogens effectively.
- Synthetic Biology: By manipulating codons, researchers can engineer new organisms with desired traits. This opens doors for sustainable biofuels and enhanced agricultural products, addressing global challenges such as hunger and climate change.
These applications show not just the scientific significance of complementary codons, but also their potential to address pressing global issues. Understanding these processes gives researchers the upper hand in innovating solutions that can impact lives.
Notable Research Findings
A number of research studies highlight the critical intersection of complementary mRNA codons and their practical ramifications. Here are some noteworthy examples:
- The ENCODE Project: This ambitious initiative revealed vast complexities about the human genome, highlighting how non-coding mRNA plays a role in gene regulation. Studies like this emphasize the importance of understanding mRNA structures and their codon components.
- CRISPR and Its Innovations: Research has documented how coupling CRISPR technology with complementary codon knowledge can improve genome editing techniques, bolstering the potential for treating genetic disorders. Researchers are fine-tuning their approaches based on codon effectiveness and minimizing off-target effects.
- Personalized Medicine: Recent advances in genomics have led to studies that explore how individual codon variations can affect drug metabolism. Understanding these nuances allows healthcare professionals to tailor medications to individual genetic profiles, improving patient care considerably.
"The journey from understanding codons to applying them in biotechnology is not just a path of learning; it is a voyage toward real-world impact."
These findings underscore that the understanding of complementary mRNA codons is not simply a matter of genetic curiosity but a driver of scientific advancement and innovation. By looking beyond the microscope, researchers can see how their work influences broader societal needs.
Future Trends in Codon Research
The exploration of complementary mRNA codons is not merely an academic exercise; it stands at the forefront of modern genetic research. As scientists strive to decode the intricate mechanisms of life, emerging trends in codon research offer not only insight but also promise substantial advancements in various fields. Understanding how codons influence gene expression can lead to innovations in genetic engineering, agriculture, and even medicine, ensuring that the relevance of this knowledge stretches well beyond the walls of a laboratory.
Emerging Technologies in Genomics
Recent advancements in genomic technologies are proving to be game-changers in the determination of complementary mRNA codons. Next-Generation Sequencing (NGS), for instance, allows researchers to decipher entire genomes with unprecedented speed and accuracy. This technology not only speeds up the identification of codons but also opens the door to real-time analysis of genetic mutations. With NGS, scientists can rapidly sequence mRNA from various organisms, enabling them to compare codon usage across species and identify evolutionary patterns.
Furthermore, CRISPR technology is revolutionizing gene editing, enhancing our ability to manipulate specific codons within a genome. By understanding which codons are necessary for producing desired traits, researchers can effectively target these sequences, allowing for precision modifications. For instance, in agricultural biotechnology, tailor-made organisms can be developed to exhibit traits like drought resistance by adjusting their codon sequences, thus improving crop yields and sustainability.
A significant boom in bioinformatics tools is also worth noting. Programs such as BLAST and Codon Usage databases can now analyze vast amounts of genetic data, making it easier to determine optimal codons for protein synthesis. These tools, combined with machine learning algorithms, can predict which codons might yield the best results for specific proteins, thus paving the way for enhanced protein engineering.
The Future of Genetic Coding
Looking ahead, the future of genetic coding is likely to shift towards a more integrated approach that combines various fields, including synthetic biology and biocomputation. As scientists better understand the nuances of codon interactions and their influence on larger genetic networks, we can expect to see a more holistic view of genetic coding emerge. This will play an essential role in creating bio-designs for applications in medicine and environmental stewardship.
Also, there's a noticeable trend towards personalized medicine where an individual's genetic information can dictate the most effective treatment pathways. Understanding mRNA codons is crucial here; it allows for tailored therapies that take into account the unique codon compositions of each person, leading to targeted therapies that minimize side effects while maximizing efficacy.
The interplay of these trends not only enhances our comprehension of genetic coding but also holds significant implications for future research and applications. As synthetic biologists work alongside geneticists, the synergies from this collaboration are bound to unlock new potentials — from developing organisms that can produce pharmaceuticals to creating biofuels derived from engineered microbes.
In the grand tapestry of genetic research, understanding complementary mRNA codons is the thread that connects knowledge to application, weaving innovations that are yet to be discovered.
Overall, the ongoing trends in codon research unveil exciting possibilities. By embracing emerging technologies and fostering interdisciplinary collaboration, the scientist community can transform the way we understand and manipulate genetics, ultimately leading to breakthroughs that were once deemed out of reach.
Epilogue
Understanding the intricacies of complementary mRNA codons is nothing short of crucial in molecular biology. This knowledge not only advances academic pursuits but also drives various real-world applications in fields such as genetics and biotechnology. As this article has demonstrated, the process begins with a solid grasp of codon structure, transitioning into the pivotal role these codons play from transcription to translation.
In summarizing the key insights presented throughout the article, we find that the nature of complementarity directly affects how genes are expressed. By detailing base pairing rules, we see a foundational structure emerge, laying the groundwork for decoding genetic sequences. This understanding can lead to significant developments in areas like genetic engineering, where accurate codon identification can enhance or alter gene expression for desired outcomes.
Furthermore, as we explored technical methods for determining codons, we emphasized the practicality of these techniques in real-world scenarios. Advances in sequencing and bioinformatics tools have made the identification of complementary mRNA codons more efficient, opening the door for innovative research and applicable solutions in the biomedical field. The relationship between technology and biology, particularly regarding complementary codons, serves to highlight the ongoing evolution of genetic research.
"The future of codon research is not merely an academic conversation; it’s a critical intersection of biology and technology that holds the potential to change the very fabric of genetic understanding."
Ultimately, comprehending codons is not merely an intellectual exercise; it translates into tangible benefits across diverse scientific disciplines. The ability to determine complementary mRNA codons plays an intrinsic role in enhancing genetic understanding and advancement, establishing a foundation that can enable breakthroughs in both research and application.