First Strand cDNA Synthesis Protocol: A Comprehensive Guide
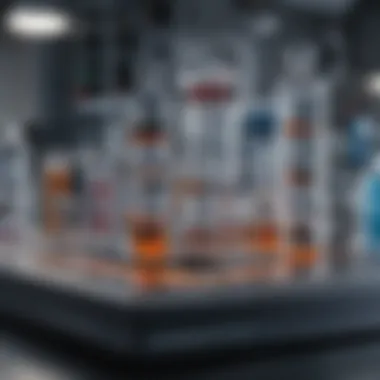
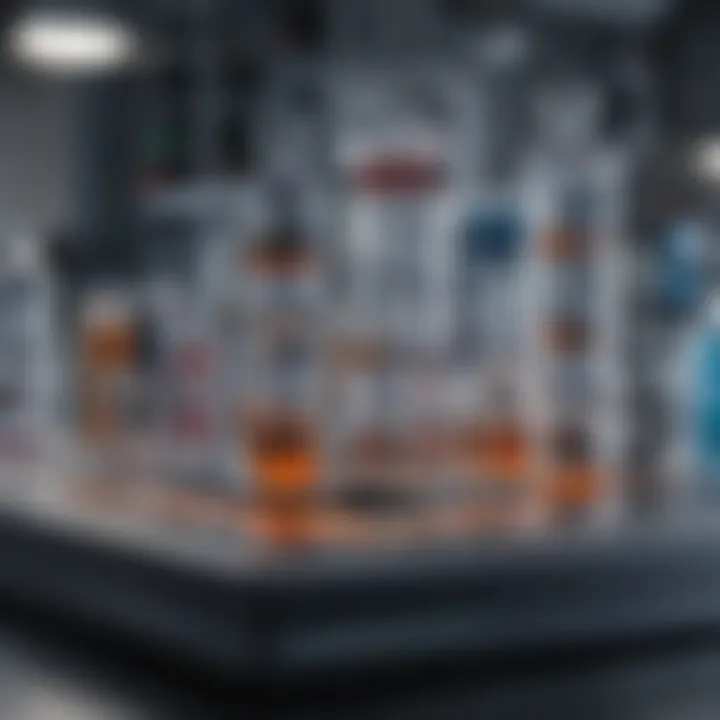
Intro
The first strand complementary DNA (cDNA) synthesis is a crucial technique in molecular biology. It serves as the foundation for many applications, especially in gene expression studies. Understanding the intricacies of this protocol is essential for both novice and experienced researchers.
This guide dives into the details of cDNA synthesis, highlighting its significance and applications. It outlines each step in the synthesis process, from choosing the correct reagents to optimizing conditions for maximum efficiency.
By addressing common challenges faced during this process, this guide aims to equip you with practical solutions and insights. The information presented will illuminate the pathway to effective first strand cDNA synthesis and showcase its relevance in contemporary research.
Recent Advances
Latest Discoveries
Recent advancements in cDNA synthesis have enhanced the accuracy and efficiency of the technique. One notable discovery is the implementation of different reverse transcriptases, which yield varying results regarding fidelity and performance. Researchers have been experimenting with commercially available enzymes like Superscript IV and RevertAid, finding significant differences in how they produce cDNA from RNA templates.
The focus has also shifted towards understanding the impact of various RNA extraction methods on the quality of cDNA synthesized. Innovations have emerged in isolating RNA to ensure high integrity and yield, paving the way for more reliable downstream applications.
Technological Innovations
Technological progress has changed how cDNA synthesis is approached. Automation of the synthesis process using liquid handling robots has minimized human error and improved reproducibility. Moreover, the development of real-time quantitative PCR (qPCR) tools aids in analyzing cDNA quality swiftly, helping researchers monitor synthesis effectiveness.
Newer methods, such as single-cell RNA sequencing, also rely heavily on robust cDNA synthesis protocols. These innovations underscore the importance of adapting to new tools, enhancing efficiency and accuracy.
Methodology
Research Design
The design of experiments centered around cDNA synthesis typically involves a systematic approach. Researchers often design their experiments to include appropriate controls, ensuring the reliability of results. This includes using RNA from known sources and varying conditions like temperature and enzyme concentration to gauge their impact on cDNA yield and quality.
Data Collection Techniques
Collecting data on cDNA synthesis involves various strategies. Effective techniques include:
- Spectrophotometry for assessing RNA concentration prior to synthesis.
- Gel electrophoresis to evaluate the size and integrity of the synthesized cDNA.
- qPCR for quantifying the cDNA products in real time.
- Next-generation sequencing for a comprehensive analysis of cDNA, especially useful in high-throughput studies.
These data collection methods ensure comprehensive analysis and substantiate findings in gene expression studies.
"Understanding the nuances in cDNA synthesis can help overcome common obstacles, ensuring that researchers obtain reliable results for their experiments."
In summary, the detailed exploration of the first strand cDNA synthesis protocol is important for advancing molecular biology applications. This article aims to provide essential insights into the entire process, aiding in producing optimal cDNA while addressing potential pitfalls along the way.
Prolusion to First Strand cDNA Synthesis
Understanding first strand complementary DNA (cDNA) synthesis is vital for anyone involved in molecular biology. This process serves as a foundation for generating cDNA from RNA templates, enabling the study of gene expression. The ability to convert RNA into cDNA broadens exploration in various fields, including genetic research and therapeutic developments.
Overview of cDNA
cDNA is a form of DNA synthesized from a messenger RNA (mRNA) template. This reverse transcription process enables researchers to study gene function and expression quantitatively. By providing a stable form of the genetic material, cDNA allows for further analysis and manipulation.
cDNA synthesis can be performed with different types of RNA, including total RNA and polyadenylated mRNA. Essential steps in the protocol involve the use of reverse transcriptase enzymes, which facilitate the formation of cDNA by utilizing RNA as a template. It is worth recognizing that the cDNA created in this process can be used in several downstream applications, including polymerase chain reaction (PCR) and sequencing.
Importance in Molecular Biology
Transcription processes are fundamental to genetics and cell biology. First strand cDNA synthesis opens new avenues for understanding how genes influence biological functions. It is particularly crucial for applications such as gene expression analysis and functional genomics. By measuring mRNA levels, researchers can discern patterns of gene expression across different conditions and treatments.
Moreover, cDNA synthesis aids in the study of RNA stability and degradation, as the cDNA product is more stable than the original RNA. This stability allows extended analysis and comprehensive profiling of gene activity, which is a significant factor in developmental biology and disease research.
"First strand cDNA synthesis is a key tool for interpreting the complex dance of gene expression."
In summary, first strand cDNA synthesis is an indispensable technique that supports various scientific inquiries. Understanding its underlying principles leads to innovative applications in research and medicine. As we advance, the insights gained from cDNA studies will continue to impact our comprehension of genetic and environmental interactions.
Principles of cDNA Synthesis
Understanding the principles of cDNA synthesis is essential for researchers and educators in molecular biology. These principles provide the necessary foundation for observing how RNA can be converted into complementary DNA. This process is crucial for numerous applications, such as gene expression analysis and cloning.
Reverse Transcription Process
The reverse transcription process is the cornerstone of cDNA synthesis. It involves enzyme-catalyzed reactions that use reverse transcriptase to create cDNA from an RNA template. The process typically occurs in three main steps:
- Initiation: The first step requires the binding of reverse transcriptase to the RNA template. Primers, often oligo(dT) or random hexamers, are used to provide a starting point for DNA synthesis.
- Synthesis: During this phase, the enzyme reads the RNA sequence and synthesizes complementary DNA. This synthesis proceeds in a 5' to 3' direction, forming a stable cDNA strand. The fidelity of this step determines the accuracy of the cDNA, which is vital for subsequent experiments.
- Termination: Once the reverse transcriptase reaches the end of the RNA template, the reaction is halted, and the cDNA product is generated. At this stage, the RNA strand may remain intact or can be degraded, depending on the specific protocol used.
This entire process emphasizes the need for optimal conditions, including temperature and pH, to ensure maximum efficiency and yield of cDNA formation.
Types of RNA Used
In cDNA synthesis, the type of RNA chosen significantly impacts both the process and the results. Here are the main types of RNA:
- Messenger RNA (mRNA): This is usually the primary target for cDNA synthesis. It carries genetic information from DNA to the ribosome, where proteins are synthesized. mRNA is preferred because it reflects the expressed genes in a given sample.
- Small Nucleolar RNA (snoRNA): These are non-coding RNAs that play a role in the modification of rRNA. While they do not code for proteins directly, they can be valuable in certain research areas where non-coding RNAs become relevant.
- MicroRNA (miRNA): Short non-coding RNA molecules involved in regulating gene expression. In some studies, cDNA synthesis from miRNA is also performed to understand their role in post-transcriptional regulation.
Each type of RNA comes with its challenges when extracting, quantifying, and converting to cDNA. Selecting the appropriate RNA is crucial, as it directly influences the quality and applicability of the subsequent cDNA results. Researchers must ensure RNA integrity and purity to facilitate effective cDNA synthesis.
In summary, grasping the principles of cDNA synthesis, especially the reverse transcription process and the types of RNA used, lays the groundwork for successful experimental design in molecular biology.
Materials Required for Protocol
In any scientific protocol, the selection of appropriate materials determines the success of the experiment. In cDNA synthesis, the quality and suitability of materials influence the integrity of the resultant product. It is essential to clearly understand both the reagents and the equipment necessary for carrying out the process effectively.
Reagents Overview
The success of first strand cDNA synthesis largely hinges on the choice of reagents. Each component plays a specific role in facilitating the reverse transcription process and should meet strict quality standards.
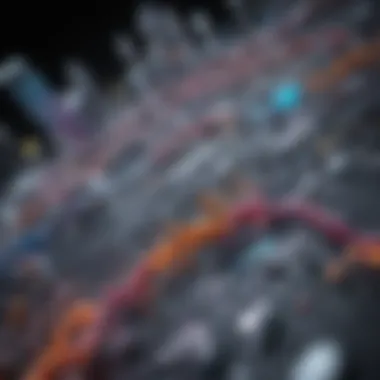
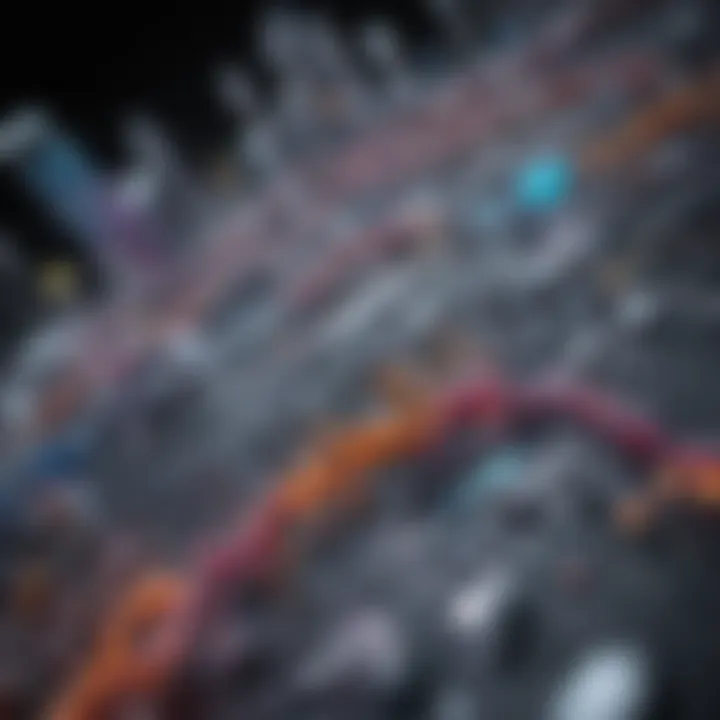
- RNA Sample: The starting material must be of high purity. Contaminated or degraded RNA can significantly lower yield and specificity.
- Reverse Transcriptase: An enzyme essential for converting RNA to cDNA. Different formulations may vary in efficiency and fidelity.
- Primers: Typically, oligo(dT) or random hexamers are used to initiate cDNA synthesis. Each type has its own advantages depending on the target gene.
- dNTPs: Deoxynucleotide triphosphates, required for cDNA elongation. Their concentration must be balanced, as excessive amounts can inhibit reactions.
- Buffer Solutions: These control the pH and ionic strength of the reaction mixture, ensuring optimal enzyme activity. A well-formulated buffer can prevent enzyme degradation.
- RNase Inhibitors: Helpful in protecting RNA from enzymatic degradation during the synthesis process. These inhibitors are particularly important if the RNA sample is exposed to potential RNases.
The careful selection of these reagents ensures effective synthesis and good yields of cDNA.
Equipment Setup
In addition to high-quality reagents, proper equipment setup is crucial for a successful cDNA synthesis protocol. The equipment must be calibrated and maintained to ensure precise functioning.
- Thermal Cycler: Temperature control is vital for both the reverse transcription and subsequent steps. Ensure that the thermal cycler is well-calibrated for the chosen settings.
- Microcentrifuge: This is used for quick spins to collect liquids at the bottom of tubes and to remove reagents. Regular maintenance is necessary to achieve consistent performance.
- Pipettes and Tips: Accurate pipetting is essential for maintaining precise reagent ratios. Filter tips are recommended to prevent contamination.
- Electrophoresis Apparatus: To evaluate the quality of synthesized cDNA, a gel electrophoresis system can be used. This will provide information on the size and purity of the cDNA product.
- Refrigerator and Freezer: Proper storage is essential for maintaining the activity of reagents. Ensure that these appliances are functioning effectively and that the reagents are stored according to manufacturers' guidelines.
"The importance of material quality cannot be overstated; even minor contaminants can result in failed experiments and misleading results."
In summary, a thorough understanding and careful selection of the materials required for the protocol is essential for successfully synthesizing first strand cDNA. The reagents and equipment lay the foundation for generating reliable and reproducible results.
Step-by-Step cDNA Synthesis Protocol
The Step-by-Step cDNA Synthesis Protocol is essential for researchers aiming to convert RNA into complementary DNA. This process is vital in various applications such as gene expression studies and functional genomics. Each phase of the protocol has specific roles and importance that contribute to the reliability and accuracy of the final cDNA product. Understanding each step allows for better troubleshooting and optimization of the synthesis process.
Isolating RNA
Isolating RNA is the very first step in cDNA synthesis. The purity and quality of the RNA sample significantly influence the success of the downstream processes. Thus, accurate isolation protocols must be followed to prevent contamination that can hinder reverse transcription.
During RNA isolation, different extraction methods can be employed; these include phenol-chloroform extraction and column-based purification. It's crucial to work in an RNase-free environment to avoid degradation. Researchers should evaluate the quality of the RNA using spectrophotometry or gel electrophoresis to ensure clear, intact RNA bands.
Key considerations for RNA isolation include:
- Sample source: Choose tissues or cell lines that have high RNA yields.
- Reagent purity: Ensure all reagents are RNase-free.
- Environmental conditions: Conduct isolations on ice to minimize RNA degradation.
"The quality of RNA directly affects the efficiency of cDNA synthesis, making its isolation a critical preliminary step."
Priming and Reverse Transcription
Once RNA is isolated, the next phase is priming and reverse transcription. This is where the actual synthesis of cDNA occurs. A primer, often an oligo(dT) or random hexamer, is crucial to initiate synthesis. The choice of primer depends on the target RNA.
The reverse transcription process involves an enzyme called reverse transcriptase. It catalyzes the synthesis of cDNA from the RNA template. Proper control over conditions such as temperature and pH is key to optimizing the activity of the enzyme. For instance, the initial incubation typically occurs at 25โ42ยฐC to allow for efficient primer annealing and extension by the enzyme.
Important points during this stage include:
- Enzyme selection: High-fidelity enzymes are preferred for accurate cDNA synthesis.
- Optimization of conditions: Adjust buffer concentrations to match the specific needs of the reverse transcriptase used.
Post-Reaction Cleanup
The final step in the protocol is post-reaction cleanup. This is necessary to remove excess primers, nucleotides, and enzymes that could interfere with subsequent reactions such as PCR. Common methods include using phenol-chloroform extraction, column purification, or ethanol precipitation.
Post-reaction cleanup ensures:
- Purity: Contaminants can inhibit downstream applications, making purification vital.
- Concentration: It may be beneficial to concentrate cDNA for future applications, adjusting the final volume accordingly.
Researchers should carefully verify the quality of the cleaned cDNA using techniques such as quantitative PCR. This validation step reassures that the cDNA is ready for use in further experimental procedures.
Optimizing cDNA Synthesis Conditions
Optimizing cDNA synthesis conditions is crucial for ensuring the efficiency and accuracy of the reverse transcription process. Both temperature control and reaction time play significant roles in the overall success of synthesizing high-quality cDNA. Proper optimization can lead to improved yields of cDNA and increased specificity, enabling reliable downstream applications such as quantitative PCR and gene expression analysis.
Temperature Control
Temperature control is one of the most vital factors influencing the synthesis of cDNA. This process requires a delicate balance between various temperature settings during different stages of the synthesis. Reverse transcription typically occurs at a temperature ranging from 37ยฐC to 50ยฐC. This is where the reverse transcriptase enzyme operates optimally, allowing efficient conversion of RNA into cDNA.
- High Temperatures: If the temperature is too high, the reverse transcriptase can become denatured. This reduces enzyme activity significantly and ultimately decreases cDNA yield.
- Low Temperatures: Conversely, if the temperature is too low, the reverse transcriptase may act inefficiently, resulting in incomplete conversion of RNA to cDNA. Therefore, finding the correct balance is key.
Factors influencing temperature settings include:
- Types of reverse transcriptase used
- Stability of RNA templates
- The presence of inhibitors in the reaction mixture
It is advisable to perform temperature optimization experiments. This can involve using a gradient PCR thermocycler to systematically test a range of temperatures during reverse transcription.
Reaction Time Considerations
The duration of the reverse transcription reaction also impacts cDNA synthesis. The length of time allotted for the reaction may vary based on factors such as:
- Concentration of RNA
- Type of reverse transcriptase
- The complexity of the RNA template
Typically, reverse transcription reactions range from 30 minutes to several hours. Shorter reaction times may not allow sufficient cDNA synthesis, while longer durations can lead to non-specific products or degradation of the RNA template.
To determine the optimal reaction time, researchers should conduct time-course experiments. This will help to assess the yield and quality of cDNA produced at different time intervals, enabling the identification of the most effective duration for specific experimental conditions.
Optimizing both temperature and reaction time is essential for maximizing the yield and quality of cDNA. Insightful adjustments based on experimental observations can significantly enhance the results of subsequent molecular analyses.
In summary, careful optimization of synthesis conditions is vital. It not only helps achieve high yields but also fosters specific and reliable results in applications like gene expression studies.
Common Challenges in cDNA Synthesis
cDNA synthesis is a vital technique in molecular biology, but it comes with its challenges. Understanding these challenges can help researchers optimize their processes and obtain more reliable results. Addressing these issues is crucial for achieving high-quality cDNA, which is needed for downstream applications such as quantitative PCR and gene expression analysis.
Degradation of RNA Samples
One significant obstacle in cDNA synthesis is the degradation of RNA samples. RNA is inherently fragile, making it susceptible to degradation by ribonucleases (RNases) present in the environment. RNases can quickly break down RNA during extraction or handling, leading to incomplete or misleading data.
To mitigate RNA degradation, it is essential to perform the following actions:
- Use RNase-free reagents and equipment: This minimizes the risk of contamination and degradation during RNA extraction and handling.
- Maintain low temperatures: Keep samples on ice whenever possible. This slows down enzymatic reactions that lead to RNA breakdown.
- Employ rapid extraction techniques: Utilizing methods like TRIzol or commercially available kits can enhance the speed and efficiency of RNA isolation, helping to preserve RNA integrity.
- Consider the quality of starting material: Always assess RNA quality and quantity using spectrophotometric measurements or gel electrophoresis prior to beginning synthesis.
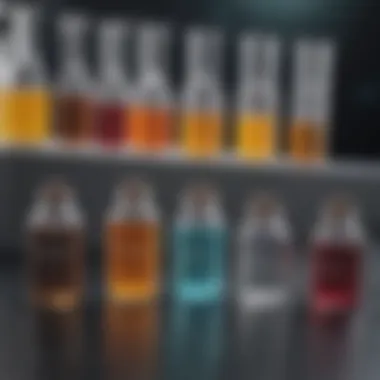
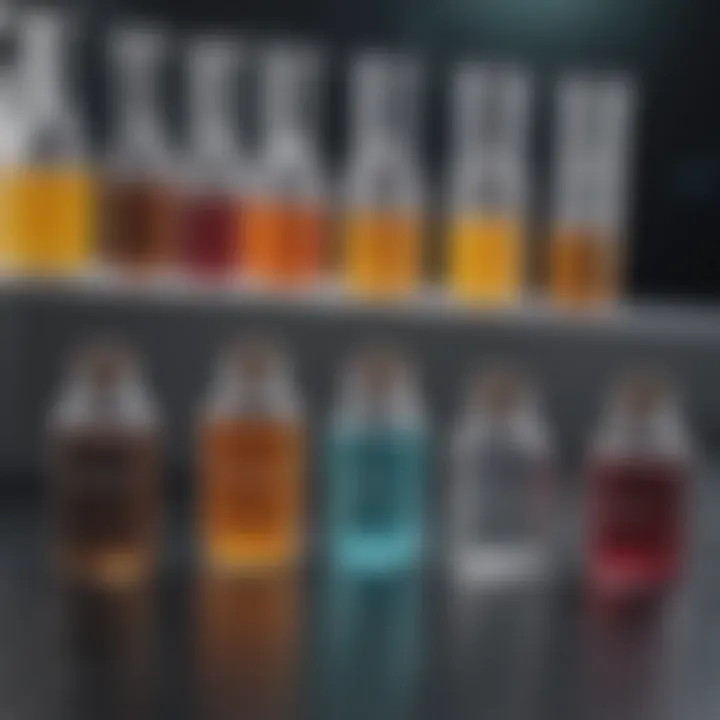
In summary, the degradation of RNA samples is a serious challenge that can have a significant impact on the success of cDNA synthesis. By taking preventive measures, one can enhance RNA stability and ensure better results.
Inefficiency of Reverse Transcriptase
Another common challenge in cDNA synthesis is the inefficiency of reverse transcriptase enzymes. Reverse transcriptase is responsible for synthesizing cDNA from RNA, but factors like enzyme choice, reaction conditions, and template integrity can all influence its performance.
Important considerations to improve reverse transcriptase efficiency include:
- Select high-quality reverse transcriptase: Choosing an enzyme that is known for its processivity and fidelity can greatly enhance the overall reaction. For instance, SuperScript IV is widely regarded for its robust performance.
- Optimize reaction conditions: Adjusting temperature, pH, and ionic strength can maximize the enzyme's activity. Generally, reverse transcription occurs optimally at 37 to 55 degrees Celsius, depending on the enzyme used.
- Proper template design: Ensure that the RNA templates used are free from secondary structures, as these can hinder enzyme binding and activity. Using appropriate priming strategies, such as oligo(dT) or random hexamers, can help to produce better cDNA yields.
- Monitor enzyme activity: Performing control reactions without templates can help identify if low cDNA yield is due to enzyme inefficiency rather than sample degradation.
By understanding and addressing the inefficiencies in reverse transcriptase performance, researchers can significantly improve their cDNA synthesis outcomes, leading to more reliable experimental data.
Troubleshooting Tips
Troubleshooting tips are crucial in the realm of first strand cDNA synthesis. They serve as a guide for researchers to navigate common issues that can arise during the synthesis process. Understanding these challenges helps improve the reliability of results and enhances the overall efficiency of experiments. In this section, we will explore specific problems that may lead to cDNA synthesis failures and strategies to mitigate them. This knowledge is particularly valuable for students, researchers, educators, and professionals alike, as it aids in refining their techniques and achieving desired outcomes.
Addressing Low Yield Issues
Low yield of cDNA can pose a significant barrier to the success of downstream applications. Various factors contribute to this issue, such as the quality of starting RNA, reaction conditions, and the efficiency of the reverse transcriptase enzyme.
- Quality of RNA: Ensure that the RNA is intact and free from contaminants. Testing the RNA concentration and integrity using spectrophotometry or gel electrophoresis can provide insights into its purity.
- Reverse Transcriptase Selection: Different reverse transcriptase enzymes exhibit varying efficiencies. It may be beneficial to experiment with enzymes like Superscript III or M-MLV, known for high yield rates.
- Reaction Conditions: Temperature and ionic strength are vital. Optimize the incubation temperature, as most enzymes prefer a specific range. Additionally, adjusting buffer components might enhance yield.
- Priming Strategy: Utilizing different primers such as Oligo(dT) or random hexamers can influence the amplification efficiency. Testing a combination of primers might provide a better yield.
- Reaction Scale: Increasing the reaction volume can sometimes improve the overall yield when working with limited RNA sources.
By understanding these factors, you can take proactive steps to enhance your yield during cDNA synthesis.
Improving Specificity
Specificity in cDNA synthesis is paramount for obtaining accurate and reproducible results. Non-specific amplification can lead to contamination of the cDNA pool with undesired products, complicating further analyses.
- Choice of Primers: The design of primers is critical in ensuring specificity. Avoiding primer-dimer formations and ensuring a proper melting temperature can greatly improve specificity.
- Optimal Primer Concentration: Too high or too low concentrations of primers can affect specificity. It is advisable to conduct a few preliminary experiments to find the right balance.
- Use of Hot Start Techniques: Employing hot start polymerases can prevent non-specific amplification at low temperatures. This ensures that the enzyme is activated only at higher temperatures, thereby enhancing specificity.
- Controlling PCR Parameters: Fine-tuning the annealing temperature and cycle number can significantly aid in enhancing specificity. Conducting gradient PCR can help define the most suitable parameters.
- Post-Synthesis Validation: Using techniques such as gel electrophoresis or qPCR can confirm that the cDNA produced is specific to the intended target. These validations are essential to avoid potential issues in downstream applications.
Applications of First Strand cDNA
First strand cDNA plays a crucial role in molecular biology. Its applications are wide-ranging and significant for various research fields. Understanding how to effectively use cDNA opens opportunities for studying gene function and expression. This section aims to highlight some key applications, including gene expression analysis and functional genomics studies.
Gene Expression Analysis
Gene expression analysis is fundamental in understanding how genes affect biological processes. When researchers want to know whether a gene is active, they often use first strand cDNA as a reference. By converting RNA back to DNA, scientists can create a stable template for amplification. This allows them to measure the levels of specific RNA transcripts in different conditions or treatments.
Benefits of Gene Expression Analysis using cDNA:
- Quantitative Measurement: Researchers can accurately quantify mRNA levels, leading to robust data.
- Response Evaluation: Helps in assessing how stressors, drugs, or environmental factors affect gene expression.
- Comparative Studies: Enables comparisons between different cell types, tissues, or developmental stages.
Moreover, technologies like quantitative PCR( qPCR) or RNA sequencing (RNA-seq) utilize cDNA for deeper insights into gene regulation. Both methods provide avenues for exploring gene interactions, pathways, and potential biomarkers.
Functional Genomics Studies
Functional genomics studies delve into understanding the roles of genes and their interrelations. First strand cDNA is essential in this context as it facilitates the dissection of complex biological systems. Researchers can analyze gene function and interaction networks through various experiments such as knockdown studies or gene overexpression.
"Functional genomics often relies on cDNA to bridge the gap between genomic data and biological insight."
Considerations when Utilizing cDNA in Functional Genomics:
- Construct Accuracy: Accuracy in cDNA synthesis impacts the quality of downstream applications.
- Library Preparation: Careful protocols are necessary when preparing cDNA libraries for sequencing.
- Experimental Design: Thoughtful design can uncover gene functions effectively.
In summary, the applications of first strand cDNA are vast and pivotal in molecular biology. Whether for gene expression analysis or functional genomics, its use enhances our understanding of genetic regulation and expression dynamics. Understanding these applications is essential for any researcher engaged in molecular biology.
Comparative Methods to cDNA Synthesis
The quest for understanding gene expression and the functional roles of RNA in biological processes has led researchers to explore diverse methodologies beyond traditional cDNA synthesis. Comparative methods to cDNA synthesis offer unique perspectives and advancements in transcriptomics. Each method has its advantages, influencing the choice based on experimental design and research objectives. Analyzing these techniques highlights their individual benefits while also presenting researchers with considerations for their applications.
Direct RNA Sequencing
Direct RNA sequencing is an innovative technique that allows for the analysis of RNA molecules without the need for conversion to cDNA. This method utilizes nanopore sequencing technology, which identifies nucleotides directly as they pass through a nanopore. One significant advantage of this approach is that it preserves the full-length sequence of the RNA, including modifications that may be lost during cDNA synthesis.
Some benefits include:
- Elimination of cDNA bias: Often, cDNA synthesis can introduce biases that affect the quantification of gene expression. Direct RNA sequencing circumvents this issue by providing data directly from RNA.
- Real-time analysis: This method facilitates real-time monitoring of RNA synthesis and degradation, allowing researchers to observe dynamic changes in RNA populations.
- Enhanced detection of rare transcripts: Since there is no amplification step involved, this technique excels in detecting low-abundance RNA species.
However, direct RNA sequencing does have challenges, including higher error rates compared to conventional sequencing methods and the need for sophisticated equipment. Additionally, it can be more costly. Despite these challenges, the method's ability to provide comprehensive RNA profiles contributes to its growing popularity in the field.
Other Amplification Techniques
Several alternative amplification techniques also assist researchers in obtaining sufficient amounts of RNA for downstream applications. These methods often complement cDNA synthesis when analyzing limited RNA quantities. Among them, methods like PCR-based amplification and in vitro transcription stand out.
- PCR-based amplification: Polymerase Chain Reaction (PCR) enables the amplification of specific RNA fragments after reverse transcription. This process enhances sensitivity and allows for the detection of specific transcripts in complex samples.
- In vitro transcription: This technique generates RNA from a DNA template. It is particularly useful when large amounts of RNA are needed. Researchers can create RNA probes or perform functional assays with the transcribed RNA, facilitating a range of experimental applications.
While these amplification methods provide valuable options, considerations include the potential for introduced errors and biases, similar to those seen in typical cDNA synthesis protocols. Furthermore, researchers must carefully select primer sequences in PCR to avoid non-specific products that may compromise results.
In summary, comparative methods to cDNA synthesis are integral to a comprehensive understanding of RNA dynamics. Each method, whether direct RNA sequencing or amplification techniques, offers distinct advantages and challenges. Adopting these alternative approaches can significantly enhance research capabilities, particularly in transcriptomics.
Future Directions in cDNA Research
Research on cDNA synthesis is moving forward at a rapid pace, with several innovative strategies and methodologies emerging. Understanding the future directions in cDNA research is significant for improving existing protocols and enhancing their application scope. As cDNA synthesis plays a crucial role in diverse fields like gene expression analysis, advancements in this area may lead to more efficient practices, speedier results, and increased accuracy.
Innovations in cDNA Synthesis Technology
Recent innovations promise to revolutionize first strand cDNA synthesis. One of the critical developments is the use of high-fidelity reverse transcriptases. These enzymes reduce errors during transcription, leading to more accurate representation of original RNA sequences.
Another promising advancement is the development of rapid and efficient one-step cDNA synthesis kits. These kits streamline the process by combining the reverse transcription and PCR amplification steps. By shortening the total time for cDNA synthesis, researchers can enhance throughput, allowing for rapid analysis of multiple samples.
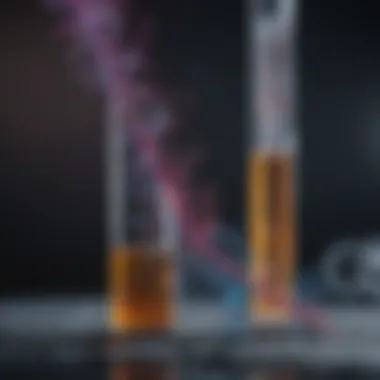
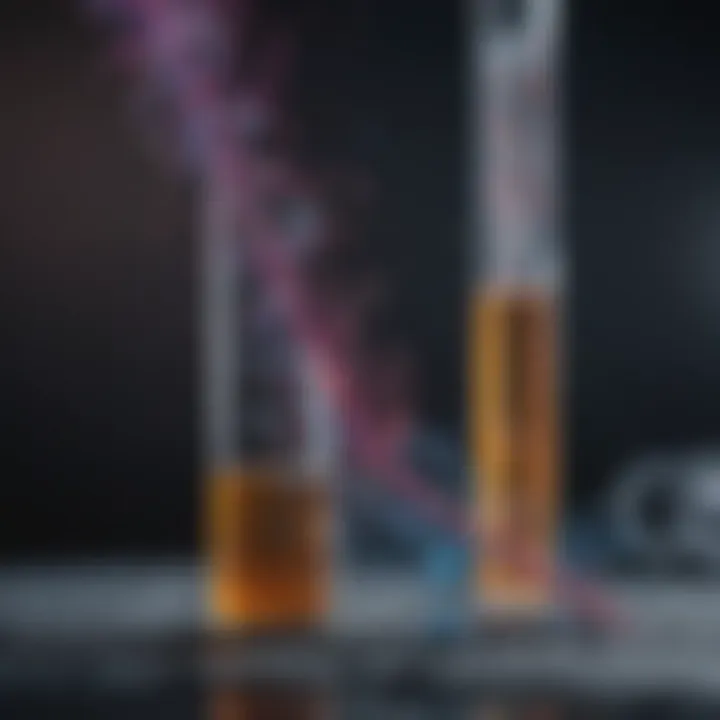
Furthermore, integration of microfluidics technology enhances the automation potential for cDNA synthesis protocols. Microfluidic devices can reduce sample volume requirements and improve monitoring of reaction parameters in real-time. This enhances control over the synthesis process, allowing for greater accuracy and less variability in outcomes.
Innovations in cDNA synthesis technology reflect a significant shift towards efficiency and accuracy in genomic research.
Integration with Other Genomic Technologies
The future of cDNA research is also intertwined with advancements in other genomic technologies. Techniques such as CRISPR-Cas9 gene editing and next-generation sequencing (NGS) are increasingly being integrated with cDNA synthesis. This integration can enrich the analytical capabilities available to researchers.
For instance, combining cDNA synthesis with NGS facilitates comprehensive transcriptomic analysis. This enables a better understanding of gene expression profiles under varying conditions, revealing insights that were previously difficult to uncover. This synergy can benefit studies focusing on specific diseases or developmental stages.
Moreover, the integration of qPCR with cDNA synthesis protocols allows for real-time monitoring of gene expression levels. This is particularly helpful in functional genomics studies where evaluation of gene activity is critical. Researchers can swiftly assess the impact of gene modifications using cDNA generated from targeted RNA samples, leading to faster experimental turnaround times.
In summary, exploring future directions in cDNA research and its innovations is essential for staying relevant in molecular biology. These advancements have the potential to shape new methodologies, establish best practices, and provide researchers with a robust toolbox for genetic analysis.
Closure
In the field of molecular biology, the first strand cDNA synthesis is crucial. This synthesis forms a connection between RNA and DNA, enabling researchers to study gene expression effectively. It is essential for a variety of applications, including diagnostics and functional genomics. Understanding the intricacies of the protocol improves the reliability of results. Effective cDNA synthesis not only facilitates experimentation, but also contributes to the advancement of ongoing biological research.
Summary of Key Points
The article focuses on several key elements related to first strand cDNA synthesis:
- Principles of cDNA Synthesis: Understanding the fundamentals helps in grasping the mechanics behind the reverse transcription process.
- Materials and Equipment: Proper selection and preparation of reagents and equipment can dramatically enhance the success rate of synthesis.
- Common Challenges: Identifying potential issues, such as RNA degradation and reverse transcriptase inefficiency, prepares researchers to tackle complications during experimentation.
- Optimizing Conditions: Adjusting factors like temperature and reaction time can improve yield and specificity.
- Applications: Knowledge of different uses for cDNA, such as gene expression analysis, adds relevance to the protocol, showcasing its importance across various fields of research.
Importance of Best Practices
Implementing best practices in cDNA synthesis is paramount for ensuring high-quality results. Key considerations include:
- Maintaining RNA Integrity: High-quality RNA is foundational. Handling samples with care prevents degradation.
- Choosing Reliable Reagents: Using high-performance reverse transcriptases can increase efficiency and yield.
- Strict Sterility Protocols: Avoiding contamination is crucial to obtaining valid results. All components should be handled in sterile conditions.
- Regular Calibration: Consistent calibration of equipment ensures proper temperature control, which is essential for the success of the reactions.
By adhering to these best practices, researchers can enhance their confidence in experimental results, pushing forward knowledge in the field of molecular biology.
Effective cDNA synthesis is not merely a procedural task; it is a gateway to revealing the complexities of gene activity, offering deep insights that were previously unattainable.
Cited Works
First, references establish the credibility of the information presented. By citing notable works in molecular biology, the document gains trust among researchers and scholars. Good scholarly practice involves acknowledging previous findings and methodologies that have laid the foundation for current research. This respect for past work ensures that readers can trace how concepts have evolved over time.
Secondly, references are essential for understanding the context and landscape of current studies in cDNA synthesis. They can elucidate the development of techniques, enabling readers to appreciate how methods have improved through innovations. This historical perspective is valuable for both novices and seasoned researchers who wish to understand the journey of scientific discovery.
Moreover, cited works allow for a deeper examination of challenges and solutions found in existing literature. These references act as resource points where readers can dive deeper into specific methodologies or problems they may encounter. For instance, if a researcher is dealing with RNA degradation issues, they can consult articles or studies that discuss relevant solutions.
In this article, references are meticulously selected to ensure they highlight the key points of the first strand cDNA synthesis protocol without overwhelming the reader. This selective citation is designed to provide clarity and prevent the narrative from becoming cluttered.
To summarize, references in this guide underscore the importance of building on previous work while offering a path for readers to extend their learning. The integration of well-chosen citations will not only enhance the article's authority but also equip readers with the resources necessary for their research endeavors.
Appendices
The appendices of an article serve a critical function. They allow for the inclusion of additional information that complements the main content without cluttering the core narrative. In the context of this article, the appendices provide essential supplementary data that can deepen the reader's understanding of first strand cDNA synthesis. This section is particularly relevant for both students and professionals who require further insights beyond the standard protocol.
Importance of Appendices in this Article
The appendices expand the scope of the guide by presenting detailed charts, tables, and graphs related to cDNA synthesis. These resources can be invaluable for visual learners and for those who seek to analyze data comprehensively. They also enable readers to access specific information quickly, which can enhance their practical application of the protocol.
Furthermore, the appendices can address topics that are crucial but do not fit neatly within the flow of the primary text. This includes advanced techniques, troubleshooting steps, or comparative analyses, which might be overwhelming if presented in the main sections. By compartmentalizing this information, the appendices make it easier for readers to digest complex topics at their own pace.
Benefits of Including Supplementary Data
- Enhanced Understanding:
- Practical Relevance:
- Facilitated Learning:
- Improved Accessibility:
- Supplementary data helps clarify the experimental design and outcomes, allowing for a richer comprehension of cDNA synthesis.
- Including raw data or results from studies allows researchers to see real-world applications, reinforcing the relevance of the protocol.
- Students and novice researchers can refer to these materials for additional educational resources, aiding their learning journey.
- Readers can access specific information without sifting through the main text, making it a more user-friendly experience.
The inclusion of well-organized and relevant appendices contributes significantly to this guide's effectiveness as a comprehensive resource. By offering these additional insights, the article not only informs but also inspires further inquiry and experimentation in the realm of molecular biology.
"Appendices are not just a supplement; they are an integral part of the narrative that support and enhance the reader's journey through complex information."
Supplementary Data
Supplementary data may include various forms of information, such as datasets from experiments, detailed methodologies, or case studies. The purpose is to provide evidence supporting claims made in the article and help readers compare their results with established benchmarks.
For instance, tables that outline the efficiency of different reverse transcriptases in cDNA synthesis can serve as crucial references during experimental design. Graphs depicting ideal conditions for RNA quality may also be beneficial.
Ensuring that this information is accurate and clearly presented will reinforce the overall credibility of the article, making it a reliable resource for ongoing research and implementation in molecular biology.
Acknowledgments
Acknowledgments serve as a pivotal component in any comprehensive guide, influencing the depth and credibility of the work. This section recognizes the individuals and institutions whose contributions enhance the quality and knowledge base of the article. Highlighting these contributions is not merely a nicety; it acknowledges the collaborative nature of scientific research, where findings often rely on collective efforts.
The significance of acknowledgments extends beyond mere recognition. It enhances transparency by explicitly stating the sources of support, whether financial, intellectual, or material. This is especially critical in fields such as molecular biology, where funding agencies, collaborative projects, and academic institutions drive research forward. By mentioning contributors, readers gain insights into the collaborative efforts that underpin the findings and protocols discussed in this article.
Furthermore, acknowledgments promote networking and building relationships within the scientific community. Recognizing contributors invites a sense of collegiality, which is essential in advancing research and discovery. When one institution or individualโs work is acknowledged, it can foster future collaborations, ensuring that knowledge and resources are effectively shared among researchers.
In this article, we particularly appreciate the following individuals and institutions for their contributions:
- Research Laboratories: Their provision of high-quality reagents and technical support is foundational.
- Institutions: Many of the advancements discussed stem from groundbreaking research conducted at various universities and research centers.
- Colleagues: Collaboration with fellow researchers has brought critical insights into optimizing cDNA synthesis.
This acknowledgment aims to reflect the collective contributions that have shaped the insights presented here.
"The essence of science is not to open the door to understood facts, but to set a sail on the sea of the unknown, guided by those who have ventured before us."
As we delve deeper into the synthesis protocols, it is this spirit of cooperation and shared pursuit of knowledge that empowers our understanding and application of cDNA synthesis.