Mastering Genomic Preparation Techniques for Research
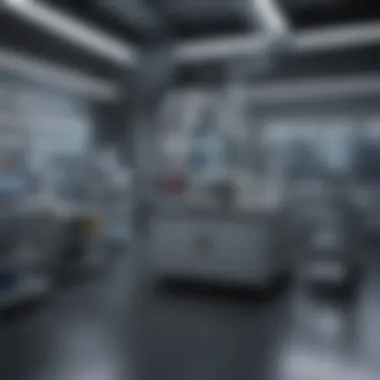
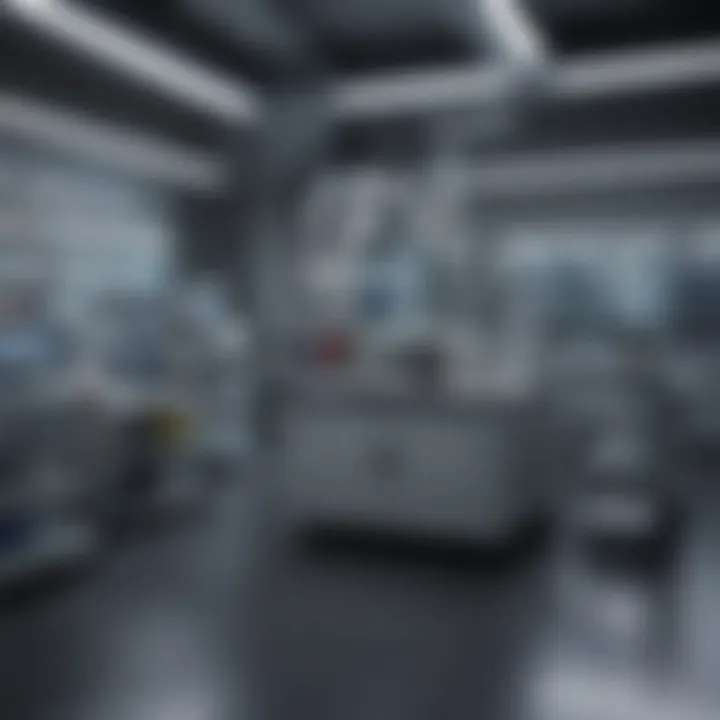
Intro
Genomic preparation encompasses the systematic processes necessary for extracting, purifying, and analyzing genetic material. This preparation is foundational for various research fields, including medicine, agriculture, and environmental science. The effectiveness of genomic studies relies heavily on the quality of the samples prepared.
A myriad of factors influences the success of genomic preparation, ranging from selection of the appropriate techniques to the rigor of the protocols employed. With advances in technology, the methodologies available today have improved significantly, allowing for greater precision and accuracy in research outcomes. This guide aims to navigate the complex landscape of genomic preparation techniques and provide valuable insights for students, researchers, educators, and professionals.
Recent Advances
Latest Discoveries
In recent years, the field of genomic preparation has witnessed considerable advancements. Novel extraction methods, such as magnetic bead-based techniques, have streamlined the process, increasing the efficiency of DNA and RNA recovery. Furthermore, advancements in next-generation sequencing technology have allowed for better analysis of genetic material, fostering a deeper understanding of genomic structures. Studies have also revealed that sample preservation methods, including temperature-controlled centrifugation, can significantly impact the integrity of genetic data.
Technological Innovations
The integration of artificial intelligence and machine learning into genomic preparation protocols represents a frontier in the research field. These technologies optimize sample preparation workflows, predict potential challenges, and recommend alternative methods to enhance sample quality. Moreover, automation is simplifying repetitive tasks in genomic laboratories, allowing researchers to focus on data interpretation and innovative applications of their findings.
"The convergence of genomics and technology is transforming research at an unprecedented pace."
Methodology
Research Design
Effective genomic preparation begins with a well-structured research design. This framework should align with the overall objectives of the study while considering the types of samples involved. The design needs to address the following factors:
- Type of genetic material (DNA, RNA, etc.)
- Source and quality of biological samples
- Purpose of the genomic analysis
- Anticipated challenges in sample handling
Data Collection Techniques
Selecting appropriate data collection techniques is pivotal in genomic preparation. These methods can include:
- Surgical extraction for tissues
- Non-invasive techniques for liquid biopsies
- Microdissection for isolating cells from heterogeneous samples
- Swab sampling for microbiome analysis
By employing robust data collection techniques, researchers can ensure that their genomic preparations yield high-quality and reliable results. Through this comprehensive guide, we will further explore the best practices and methodologies for successful genomic preparation.
Prelude to Genomic Preparation
Genomic preparation serves as a foundational step in the field of genomics, primarily focusing on the extraction and analysis of genetic material. This section emphasizes how crucial genomic preparation techniques are for research and application across various scientific domains. Effective genomic preparation ensures the quality and reliability of results, which are essential for advancing knowledge in genetics.
Definition and Scope
Genomic preparation involves the systematic collection, processing, and preservation of DNA and RNA samples from various sources. It encompasses several techniques aimed at isolating nucleic acids suitable for downstream applications. Its scope includes human, plant, and microbial genomes, each requiring tailored methods based on the unique characteristics of the sample type. This diversity underlines the necessity of understanding specific techniques and protocols for accurate genomic analysis. The handling of samples must comply with best practices to minimize contamination and degradation.
Importance in Genomic Research
The significance of genomic preparation cannot be overstated. It is pivotal in ensuring the integrity of data generated in genomic studies. Properly prepared samples yield higher-quality results, which facilitate precise interpretations in various research areas such as medicine, agriculture, and environmental science.
- Research Reliability: Well-prepared genomic samples result in lower rates of experiment failure and data discrepancies.
- Application Versatility: Diverse techniques allow for applications in clinical diagnostics, genetic engineering, and conservation efforts.
- Technological Advancements: As sequencing technologies evolve, so does the need for optimized preparation methods to keep pace with these innovations.
"Quality genomic preparation is a prerequisite for successful genomic research, impacting both the accuracy and depth of findings."
In summary, the introduction to genomic preparation reveals its critical role as both an enabler of scientific discovery and a determinant of research integrity.
Fundamental Concepts of Genomics
Understanding the fundamental concepts of genomics is essential for any scientist embarking on genomic research. These concepts lay the groundwork for more complex techniques and applications that will be discussed later in this guide.
Basics of DNA and RNA
DNA, or deoxyribonucleic acid, serves as the blueprint for all living organisms. It consists of sequences of nucleotides, which are the fundamental units. Each nucleotide contains a sugar, a phosphate group, and a nitrogenous base. There are four primary bases in DNA: adenine, thymine, cytosine, and guanine. The specific sequences of these bases encode genetic information that dictates the development, functioning, and reproduction of organisms.
RNA, or ribonucleic acid, plays a prominent role in translating the genetic information from DNA into proteins, which perform most vital processes in cells. Unlike DNA, RNA is usually single-stranded and contains uracil instead of thymine. RNA is crucial in various biological processes, including protein synthesis and gene regulation. The interplay between DNA and RNA is central to cellular function.
Understanding Genomic Structures
Genomic structures refer to the composition and organization of genetic material within an organism. In eukaryotic organisms, the genome is divided into chromosomes, which are structures that house the DNA. Humans, for instance, have 46 chromosomes arranged in 23 pairs. Complex genomes have repetitive sequences and could contain varying gene densities across different regions.
The structural elements of genomes are not just limited to coding regions but also include non-coding regions, which may have regulatory functions. Understanding these structures is vital as it impacts how genes are expressed and regulated.
As genomics advances, the identification and functional characterization of these structural elements become crucial. This can lead to significant implications in fields like personalized medicine, where understanding the genetic foundation of diseases can directly influence treatment approaches.
The study of genome structure is a cornerstone of genomics and is fundamental to interpret how genetic variations can lead to different phenotypes.
In summary, a solid grasp of DNA and RNA, along with the structural organization of genomes, forms the basis for advanced genomic studies and applications. This knowledge facilitates the evaluation of techniques in genomic preparation, adding depth to research endeavors.
Types of Genomic Samples
Understanding the types of genomic samples is crucial for effective genomic preparation techniques. Different sources of genomic material have unique characteristics, and the choice of sample type can significantly influence the outcomes of research. Each type of sample comes with specific considerations, such as the methods used for extraction, the integrity of the DNA or RNA, and the subsequent applications. By comprehending the differences in genomic samples, researchers can tailor their methodologies for optimum results.
Human Genomic Samples
Human genomic samples are primarily obtained from biological sources like blood, saliva, or tissue. These samples are invaluable in various fields, including medicine and genetics. The ability to analyze human genome variations has led to significant advancements in personalized medicine. However, one must consider ethical implications and regulatory requirements in the handling of human samples.
Extraction methods must maintain sample integrity and minimize degradation. For instance, blood samples may require immediate processing to prevent an increase in cellular degradation. Researchers should also be mindful of factors like demographic variability, as it can affect genomic data interpretation.
Plant Genomic Samples
Plant genomic samples are essential for agricultural and ecological research. These samples provide insights into genetic traits, disease resistance, and crop yield improvements, which are vital for food security. Common sources include leaf, root, and seed tissues. Each plant part holds specific genomic information, and the extraction process must reflect this diversity.
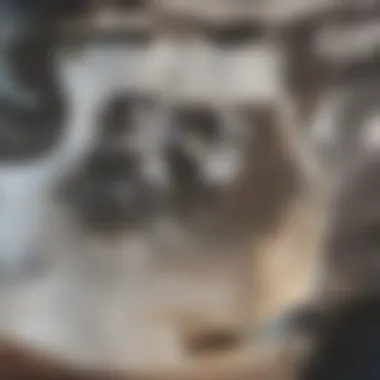
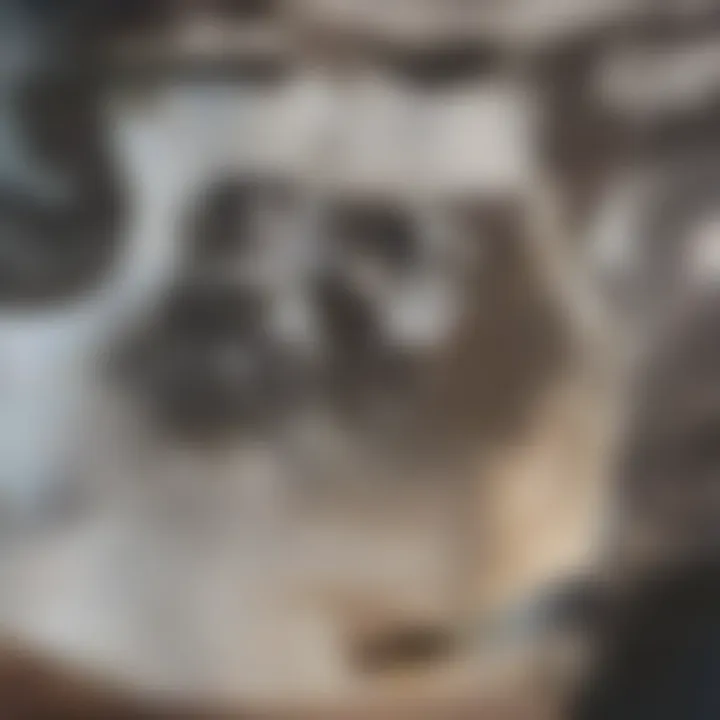
One consideration in handling plant genomic samples is the presence of secondary metabolites, which could interfere with DNA or RNA extraction. It is necessary to select appropriate techniques tailored for plant tissues. Efficient extraction methods like the CTAB method are often employed to purify genomic material while dealing with the complexities found in plant cells.
Microbial Genomic Samples
Microbial genomic samples are critical in microbiology, environmental studies, and biotechnology. These samples can be sourced from soil, water, or cultured organisms. The microbial genome can reveal interactions with the environment and contribute to fields like disease prevention and bioremediation.
Obtaining microbial samples can be particularly challenging due to their diversity and the necessity of capturing a representative population. Concentration methods, such as filtration or centrifugation, might be used to isolate specific microbes from environmental samples. Working with microbial DNA often requires specific conditions because of the potential for contamination and degradation, making sample handling and preparation techniques vital for accurate results.
Techniques for Genomic Preparation
The field of genomics is expanding rapidly, making the techniques for genomic preparation vital for researchers. These methods facilitate the extraction and analysis of genetic material, which is fundamental for diverse applications such as clinical diagnostics, agricultural development, and environmental assessments. Understanding these techniques allows professionals to optimize their workflows, leading to more accurate data generation and analysis. It ultimately enhances the capabilities to study genetic information in various contexts. Recognizing the methodology can also assist in addressing errors that can arise during preparation, improving the overall quality of genomic studies.
DNA Extraction Methods
Phenol-Chloroform Method
The Phenol-Chloroform Method is a classic technique used for DNA extraction, well-regarded for its effectiveness in purifying nucleic acids from cellular materials. This method utilizes a mixture of phenol and chloroform to separate DNA from proteins and other contaminants. Its significance lies in the ability to yield high-purity DNA, which is crucial for downstream applications like PCR and sequencing.
A key characteristic of the Phenol-Chloroform Method is its efficiency in removing proteins from nucleic acids. This results in cleaner samples that present fewer complications during analysis. However, one must consider that this technique requires careful handling of hazardous chemicals, which can pose safety risks. Moreover, the process can be time-consuming compared to more modern methods.
The unique feature of this method is its proven reliability in both laboratory and field settings. It remains a popular choice among researchers looking for consistency in DNA preparation, despite the downsides of chemical exposure and the procedural complexity.
Silica Membrane Method
The Silica Membrane Method represents an advancement in DNA extraction techniques, gaining popularity in various laboratory settings. This approach relies on the property of silica to adsorb DNA under specific conditions, allowing for simple and efficient extraction of nucleic acids. Its contribution to genomic preparation is noteworthy for the rapidity and simplicity it brings to the extraction process.
A compelling feature of the Silica Membrane Method is its capacity to purify DNA without the need for hazardous solvents. This makes it safer and broadly acceptable in clinical and educational contexts. Many available commercial kits offer its use, providing user-friendly protocols for researchers.
However, while it streamlines the process, variations in quality and yield can arise based on factors such as sample type and extraction conditions. This is something users should keep in mind when choosing this method.
Magnetic Bead Method
The Magnetic Bead Method has emerged as a modern technique for DNA extraction, utilizing magnetic beads coated with substances that specifically bind DNA. The method facilitates a quick cleanup of DNA from various sample types, contributing effectively to the overall genomic preparation process.
This technique's key characteristic lies in its speed and versatility, making it adaptable to different workflows. The ease of use is complemented by the significant reduction in time taken compared to traditional methods. Furthermore, it is less labor-intensive.
On the flip side, while magnetic bead extraction is efficient, it may occasionally lead to lower yields, especially in challenging samples. Thus, it is essential for researchers to assess the appropriateness of this method, depending on their specific requirements and sample conditions.
RNA Extraction Methods
Trizol Method
The Trizol Method is a widely recognized technique for RNA extraction, utilizing a reagent that facilitates the separation of RNA from DNA and proteins in a single-step procedure. This method is particularly important for researchers focusing on gene expression studies and functional genomics.
One of its key characteristics is its capacity to extract both RNA and DNA from the same sample. This dual extraction can save valuable time and resources. Furthermore, Trizol is known for its effectiveness in isolating high-quality RNA suitable for various downstream applications.
However, this method requires careful handling due to the toxicity of Trizol, presenting safety concerns. Additionally, residual organic solvents may occasionally interfere with subsequent analyses, necessitating thorough purification steps.
Mini Column Kits
Mini Column Kits offer another efficient option for RNA extraction, designed to provide user convenience while ensuring yield quality. These kits use a silica-based membrane within a column, allowing for RNA capture during centrifugation. This method is beneficial for its speed and straightforward implementation.
Important features include standardized protocols that minimize variability across different users and laboratories. This consistency makes it an attractive choice for researchers who need reliable results.
Nevertheless, costs associated with these kits can add up in high-throughput settings. Also, the effectiveness may vary depending upon the material being used.
CTAB Method
The CTAB Method is another approach utilized primarily for extracting RNA, especially from plant tissues. This method utilizes cetyl trimethyl ammonium bromide to facilitate the breakdown of cell walls and the isolation of RNA.
The unique value of the CTAB Method lies in its robustness with challenging materials, such as those containing high levels of polysaccharides or phenolic compounds. This characteristic makes it especially advantageous for plant research.
That said, the procedure can be labor-intensive and requires careful protocol adherence to ensure RNA integrity. Moreover, potential contamination with plant metabolites may pose challenges in subsequent assays.
"Choosing an optimal technique for genomic preparation is essential to achieve reliable results, paving the way for groundbreaking discoveries across various scientific fields."
In summary, understanding the diverse techniques for genomic preparation allows researchers to make informed decisions tailored to their specific needs and sample types.
Quality Control in Genomic Preparation
Quality control is a pivotal aspect of genomic preparation. It ensures that the genomic samples are suitable for downstream applications. Quality control involves monitoring processes throughout the sample preparation phase. This attention to detail can dramatically influence results in genomic analyses, including next-generation sequencing and microarray experiments.
Maintaining high-quality samples reduces the likelihood of erroneous data. Contaminants can obscure true genetic information. Degraded DNA or RNA can lead to misinterpretation of genomic data. Hence, implementing robust quality control measures is essential.
Importance of Quality Control in Genomic Preparation:
- Integrity Assurance: Evaluating the integrity of DNA and RNA helps ensure that the samples remain intact and functional.
- Purity Assessment: Assessing the purity of genomic material is crucial. Impurities can inhibit reactions required for sequencing or other analyses.
- Reproducibility: High-quality preparations lead to reproducible results across experiments.
Assessing Integrity and Purity
Assessing the integrity and purity of genomic samples is vital for successful experiments. Several methods exist to achieve a thorough evaluation of these characteristics. The goal is to establish the quality of the nucleic acids.
Techniques for Assessing Integrity and Purity:
- Gel Electrophoresis: This method separates nucleic acids based on size. It provides visual confirmation of integrity by showing distinct bands. A well-defined band indicates high integrity.
- RNA Quality Number (RQN): Specifically for RNA samples, RQN assesses quality based on electrophoretic mobility. A high RQN indicates minimal degradation.
- A260/A280 Ratio: The absorbance ratio helps ascertain nucleic acid purity. A ratio of ~1.8 for DNA and ~2.0 for RNA is considered acceptable, suggesting minimal protein contamination.
Utilizing Spectrophotometry
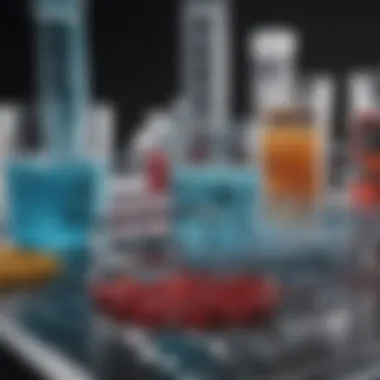
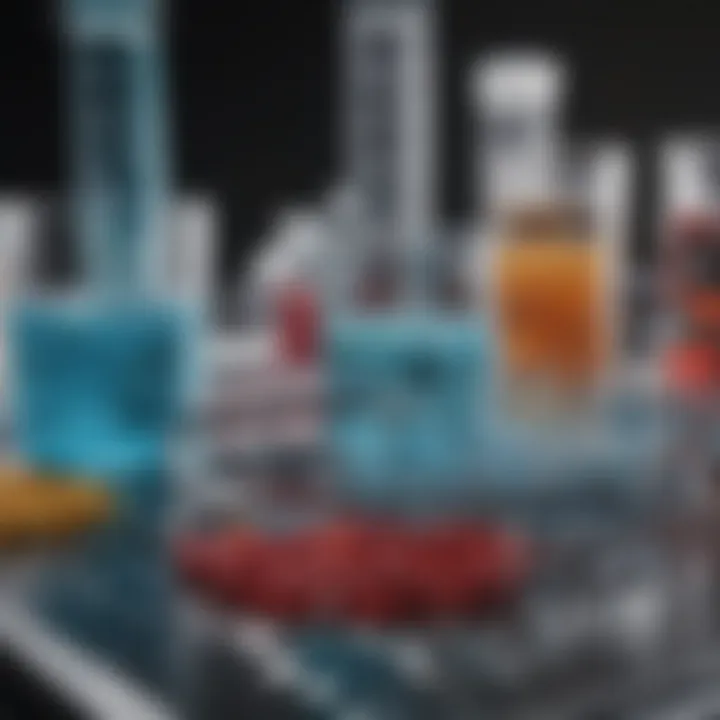
Spectrophotometry offers a non-destructive, rapid method for assessing nucleic acid concentration and quality. It relies on the absorption of UV light by nucleic acids. Different wavelengths reveal essential information regarding the samples.
Spectrophotometric Analysis:
- Principle: Nucleic acids absorb UV light at specific wavelengths. For instance, nucleic acids absorb at 260 nm. This can give a concentration reading based on the Beer-Lambert law.
- Procedure: Performing a spectrophotometric measurement involves placing a sample in a cuvette. Note the A260 measurement for concentration. Calculate purity using the earlier mentioned A260/A280 ratio.
- Benefits: Spectrophotometry is quick and does not require extensive sample preparation. Results can be generated in minutes, aiding in rapid decision-making for sample usage.
"Quality control in genomic preparation is the backbone of reliable genomic research. Without accurate assessments, the scientific conclusions drawn may be fundamentally flawed."
Genomic Preparation for Next-Generation Sequencing
Genomic preparation for next-generation sequencing (NGS) is a critical aspect of genomic research. This stage significantly influences the quality and accuracy of sequencing results. Since NGS technology allows the analysis of vast amounts of genetic data simultaneously, the preparedness of genomic samples becomes essential. Good preparation ensures that the data generated is both reliable and reproducible.
Effective genomic preparation includes several steps, from sample collection to library preparation. For researchers and professionals in the field, understanding these steps can optimize workflow and enhance data quality. Benefits of proper genomic preparation include minimizing contamination risks, maximizing yield, and ensuring high-quality sequencing output. By paying attention to these details, researchers can achieve more successful sequencing results.
Considerations during this phase encompass not just the techniques used, but also the quality control measures necessary to ensure that the samples are suitable for sequencing. These measures help detect potential problems that could arise during the sequencing process, such as inadequate DNA or RNA concentration, or the presence of contaminants. Therefore, it is crucial to incorporate rigorous protocols during genomic preparation.
Library Preparation Techniques
Library preparation is one of the key elements of genomic preparation for NGS. This process includes several steps: fragmenting genomic DNA, adding adapters, and amplifying the library. The quality of this library has a direct impact on the sequencing results.
- Fragmentation: It is typically done by physical methods like sonication or enzymatic digestion. The goal here is to create DNA fragments of a suitable size for sequencing.
- Adapter Ligation: After fragmentation, adapters are added. These small segments of DNA are necessary for the sequencing process. They provide a way for the sequencing platform to recognize and bind the DNA strands.
- PCR Amplification: The library is then amplified by polymerase chain reaction (PCR). This step increases the amount of DNA available for sequencing and improves the representation of the original sample.
Library preparation must be carried out with precision and care. Any errors during this process can lead to low yield or biased results, which could misrepresent the genetic information.
Adapters and Indexing Strategies
Adapters are essential in NGS workflows. They not only facilitate binding to the sequencing platform but also play a critical role in allowing the identification of individual fragments. Different indexing strategies help to multiplex samples, which enhances throughput.
- Unique Indexing: Each sample can be tagged with a unique sequence. This allows multiple samples to be sequenced in a single run without confusion over which data belongs to which sample.
- Dual Indexing: This method uses two unique indices per sample, reducing the risk of index hopping and improving data quality.
- Barcoded Adapters: A practical way of using adapters that contain barcode sequences. This method simplifies library preparation and allows a greater number of samples to be processed simultaneously.
Incorporating the right adapters and indexing strategies is vital. They affect both cost-effectiveness and the overall success of the sequencing project. Thus, researchers should be knowledgeable about current best practices and emerging technologies in this field.
Effective genomic preparation is not just a step in sequencing; it shapes the foundation upon which data quality is built.
Computational Tools in Genomic Preparation
Computational tools play a vital role in genomic preparation. Their importance spans data analysis, management, and interpretation, which are all integral for a successful genomic study. As researchers collect vast amounts of genomic data, they need efficient tools to analyze this information. Bioinformatics software facilitates this process, helping to decode complex genetic information.
Bioinformatics Software for Data Analysis
Bioinformatics software has revolutionized the way genomic data is analyzed. These programs allow researchers to predict gene functions, identify genetic variants, and even model genetic interactions. Common software tools include BLAST, Bowtie, and GATK. Each offers unique capabilities and is used in different contexts.
For example, BLAST is often used for comparing nucleotide sequences, while Bowtie is optimized for mapping short DNA sequences to long reference sequences. Furthermore, GATK helps in processing next-generation sequencing data, making it essential for variant discovery.
The integration of these tools often improves research quality. Efficient data analysis can lead to more accurate interpretations of genomic data. With evolving technologies, these bioinformatics platforms now incorporate machine learning algorithms. This ability enhances predictive accuracy and speeds up data processing significantly.
Pipeline Development for Processing Genomes
Developing a robust pipeline for processing genomes is essential in genomic projects. Pipelines streamline workflows, ensuring that each step of data processing is efficient and reproducible. A well-designed pipeline enhances the overall reliability of genomic findings and fosters collaboration among researchers.
A typical genomic analysis pipeline might include the following steps:
- Data Acquisition: Collect sequencing data from relevant sources.
- Preprocessing: Clean and filter raw data to eliminate poor-quality reads.
- Alignment: Use software like Bowtie or BWA to map sequencing reads to a reference genome.
- Variant Calling: Apply tools such as GATK to identify genetic variants.
- Annotation: Utilize databases to classify and interpret variants.
Having automated and well-documented pipelines ensures that researchers can reproduce results effectively. This enhances transparency and can lead to improved collaboration in the scientific community.
In summary, computational tools, especially bioinformatics software and well-structured pipelines, significantly influence genomic preparation. Their integration into the research process not only improves accuracy but also saves time, ultimately fostering advances in genomic science.
Applications of Genomic Preparation
Genomic preparation plays a foundational role in various scientific fields due to its ability to provide high-quality, reliable data from genomic samples. The meticulous process of genomic sample preparation influences outcomes in research, diagnostics, agriculture, and environmental science. Understanding how genomic preparation is applied across these domains deepens the appreciation of its significance in advancing knowledge and technology.
Clinical Applications
The contribution of genomic preparation to clinical applications cannot be understated. The primary objective in clinical settings is to ensure the accuracy of diagnoses and treatments. For instance, in cancer research, genomic data informs personalized medicine approaches, allowing for targeted therapies that improve patient outcomes. Advanced methods of preparation, such as nucleic acid extraction, are crucial for obtaining pure samples from diverse tissues. Proper preparation enhances the reliability of genetic testing, which aids in making informed health choices.
The implications extend to genetic disorders where early detection through genomic analysis can significantly alter treatment paths. Genetic screening utilizes well-prepared genomic samples to identify mutations associated with inherited diseases. The somatic variant analysis, achieved through proper preparation, is essential for tracking the evolution of diseases, thus facilitating timely interventions in clinical practice.
Agricultural Advancements
In agriculture, genomic preparation lends itself to innovations and improvements in crop and livestock management. Precise genomic preparation techniques enable the extraction of DNA and RNA from plant and animal samples, fostering advancements in breeding programs. The accuracy in genomic analyses allows scientists to select desirable traits more effectively, enhancing the efficiency of breeding and resulting in higher yields.
Moreover, genomic preparation assists in developing genetically modified organisms (GMOs) which can withstand pest or disease pressure, contributing to food security. The insights gained from carefully prepared genomic samples allow researchers to understand gene function and expression patterns better, paving the way for sustainable agricultural practices.
Environmental Monitoring
Genomic preparation is equally vital in environmental monitoring. It helps elucidate the genetic diversity of organisms within ecosystems, which in turn informs conservation strategies. The preparation of environmental DNA (eDNA) samples allows for the assessment of biodiversity in aquatic and terrestrial environments. By identifying species presence through prepared samples, researchers can track changes in ecosystems and detect the introduction of invasive species.
Using genomic techniques can reveal how organisms respond to environmental changes, such as climate change or pollution. Proper sample preparation ensures that the data collected is robust and reflective of true environmental conditions. Therefore, genomic preparation serves as a critical component in developing strategies aimed at preserving ecosystems and restoring habitats.
In summary, the applications of genomic preparation are vast and multifaceted, spanning clinical, agricultural, and environmental fields. Each domain benefits distinctly from the quality of genomic samples, enhancing research, improving health outcomes, and supporting sustainable practices.
Emerging Trends in Genomic Preparation
Emerging trends in genomic preparation are reshaping the landscape of genomic research. These trends not only enhance the accuracy of genomic analyses but also expand the scope of applications across various disciplines. In this section, we discuss two particularly significant trends: single cell genomics and metagenomic approaches. Both provide unique advantages and address various challenges in the field.
Single Cell Genomics
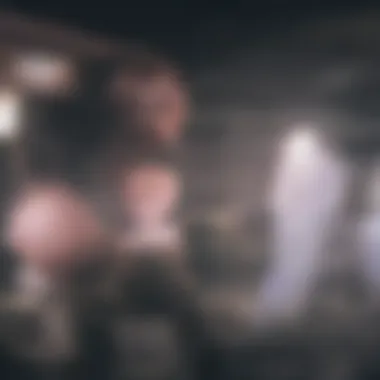
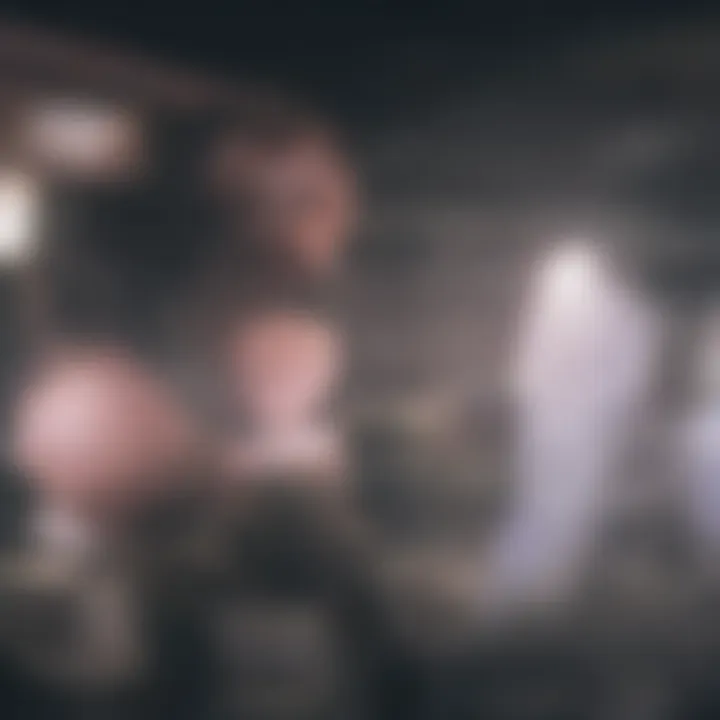
Single cell genomics is a transformative approach that allows researchers to study the genetic material of individual cells. This method overcomes limitations associated with bulk analysis, where average properties of a population obscure individual variations. Techniques like single cell RNA sequencing have opened doors to understanding cellular heterogeneity within tissues, which is crucial for fields like cancer research and developmental biology.
Benefits of Single Cell Genomics:
- Resolution of Cellular Diversity: This method allows detailed insights into cellular populations, revealing rare cell types that may contribute significantly to diseases.
- Personalized Medicine: By analyzing single cells from individual patients, clinicians can better tailor treatments to patient-specific mutations and cellular profiles.
- Temporal Dynamics: Researchers can track changes in gene expression over time within single cells, leading to a deeper understanding of biological processes.
Considerations:
- Technical Complexity: Single cell isolation and analysis require sophisticated technologies like microfluidics and advanced bioinformatics tools.
- Cost: The methodologies can be resource-intensive, limiting accessibility for some laboratories.
Metagenomic Approaches
Metagenomic approaches involve the analysis of genetic material recovered directly from environmental samples. This technique enables the study of complex microbial communities without the need for culturing individual species. Metagenomics has significant implications for environmental science, ecology, and human health.
Benefits of Metagenomic Approaches:
- Diversity Assessment: It allows researchers to characterize the full diversity of microbial communities, providing insights into ecosystem functions.
- Pathogen Discovery: Metagenomics can identify unknown pathogens in clinical samples, contributing to epidemiological studies and outbreak investigations.
- Biotechnological Applications: Insights gained from metagenomic analysis can lead to the discovery of novel enzymes and other biomolecules for industrial applications.
Considerations:
- Data Complexity: The analysis of metagenomic data requires advanced computational methods to manage vast datasets and unpack biological relevancy.
- Taxonomic Resolution: Identifying species accurately can be challenging due to high genetic variability within microbial families.
"Single cell genomics and metagenomics represent a paradigm shift in our ability to understand genetic complexities and interactions within biological systems."
In summary, the emergence of single cell genomics and metagenomic approaches signifies a new era in genomic preparation, offering innovative ways to explore genetic material and biological complexity. These methods pave the way for future advancements that could revolutionize research and clinical practices.
Challenges in Genomic Preparation
Genomic preparation is essential for the success of various research applications. However, challenges in this field can significantly impact the results and integrity of genomic data. Understanding these obstacles is critical for researchers, as they can influence the quality of genomic analysis.
Contamination Risks
Contamination poses a major threat during genomic preparation. Even minimal contamination can lead to misleading results. Common sources include environmental factors, handling errors, and cross-contamination between samples.
Researchers must implement stringent protocols to minimize these risks. This can include using sterile techniques, maintaining clean workspaces, and employing specialized equipment. It might also be helpful to perform routine checks to identify potential contamination sources systematically.
"Preventing contamination is crucial; it ensures the reliability and reproducibility of genomic research."
To further mitigate the risk, applying practices such as:
- Using dedicated tools for each sample
- Regularly sanitizing surfaces and equipment
- Employing positive displacement pipettes
Such measures are not merely precautions; they are necessary steps in ensuring the integrity of genomic data.
Sample Degradation Issues
Sample degradation is another pressing challenge in genomic preparation. DNA and RNA samples are sensitive to a range of factors, including temperature, enzymatic activity, and exposure to light. Degradation can occur rapidly if samples are not processed or stored properly.
Understanding the optimal conditions for storage and processing is vital. For example:
- Store samples at low temperatures to slow down degradation.
- Make use of protective agents like RNAlater to stabilize RNA samples.
In addition, researchers must be cautious when isolating nucleic acids. Prolonged exposure to harsh chemicals or improper handling can lead to significant damage. Therefore, following standardized protocols can help in preserving the integrity of genomic samples.
Finally, performing quality checks at different stages of preparation can be beneficial. Techniques such as gel electrophoresis or spectrophotometry can confirm the integrity and purity of the samples. By addressing these challenges proactively, researchers can maintain robust genomic preparations that facilitate accurate analysis.
Future Directions in Genomic Preparation
The evolving landscape of genomic research presents numerous opportunities and challenges. As technologies advance, the future of genomic preparation is crucial for enhancing the accuracy and efficiency of genomic studies. This section will delve into innovative technologies and the integration of AI, which hold great potential for transforming traditional genomic workflows.
Innovative Technologies
Technological advancements are continuously reshaping genomic preparation. One significant area of development is the improvement in sequencing technologies. Next-generation sequencing (NGS) has transitioned from first-generation platforms, allowing for faster and more cost-effective sequencing. This evolution enables researchers to analyze larger datasets, enhancing variant discovery and genomic characterization.
In parallel, advancements in sample processing techniques, such as microfluidics, are streamlining genomic preparation. By utilizing miniaturized systems, researchers achieve precision and higher throughput. This method reduces reagent costs and sample usage while minimizing contamination risks. Moreover, single-cell genomics has emerged, enabling the study of heterogeneity in cell populations. This technology offers insights into cellular behavior that bulk sequencing cannot capture.
Additionally, the development of portable sequencing devices means that genomic preparation can now be conducted outside traditional laboratory settings, such as in remote locations or during field studies. Such innovation opens doors to real-time data collection and analysis, significantly impacting fields like epidemiology and conservation.
Integration of AI in Genomic Workflows
Artificial intelligence is rapidly integrating into genomic workflows, pushing the limits of analysis and interpretation. AI algorithms now assist in data processing, allowing researchers to analyze vast genomic datasets efficiently. For instance, machine learning models can predict gene functions, identify variants associated with diseases, or assist in understanding complex biological systems.
Moreover, AI-driven tools enhance quality control processes during genomic preparation. Automated systems can monitor sample integrity throughout various stages, reducing human errors and ensuring reproducible results. By implementing AI, researchers can also develop predictive models that customize genomic preparation protocols tailored to specific sample types or research goals.
The future vision for genomic preparation includes seamless integration of AI with laboratory techniques. For example, automating sample extraction and analysis can lead to lower costs, increased reproducibility, and a faster turnaround of genomic data. This synergy between AI and genomics will likely produce innovative solutions to existing challenges within the field, such as contamination and sample degradation.
The convergence of innovative technologies and AI is set to advance genomic preparation significantly, paving the way for transformative discoveries in the realm of genomics, personalized medicine, and beyond.
The End
The conclusion serves as the vital endpoint of this article, providing a synthesis of the comprehensive analysis presented throughout. In this discussion of genomic preparation techniques, the emphasis has been placed on critical methodologies, tools, and their impact on research accuracy. It is essential for researchers to understand and master these techniques to ensure high-quality results in their genomic applications.
One of the specific elements highlighted is the importance of quality control measures. Without proper assessment of sample integrity and purity, the foundation of genomic analyses may be compromised, leading to erroneous conclusions. Incorporating robust quality control practices promotes confidence in findings, contributing to the advancement of knowledge in the genomic field.
Summary of Key Points
- Genomic Preparation Techniques: The article covers various techniques for preparing genomic samples, focusing on both DNA and RNA extraction methods.
- Quality Control: Emphasizes the necessity of assessing sample integrity, underscoring methods like spectrophotometry for reliable analysis.
- Applications: Discusses significant applications across clinical, agricultural, and environmental domains, showcasing the real-world relevance of genomic techniques.
- Emerging Trends: Presents a glimpse into innovative practices such as single cell genomics and metagenomic approaches.
- Challenges: Addresses potential pitfalls, including contamination risks and sample degradation issues, urging continuous improvement in methodologies.
Implications for Future Research
Future research in genomic preparation will thrive upon the foundational techniques outlined here. The integration of innovative technologies will further enhance the precision and efficiency of genomic analyses. As we move forward, understanding how to implement artificial intelligence tools in genomic workflows will be integral.
Moreover, the exploration of novel genomic preparation methods can drive discoveries in various disciplines. Researchers must remain adaptable, ready to embrace changes that push the boundaries of what is currently achievable. The implications of these advancements are vast, ranging from improved therapeutic strategies in medicine to enhanced crop yield and sustainability in agriculture.