In-Depth Analysis of Electrical Transformer Calculations
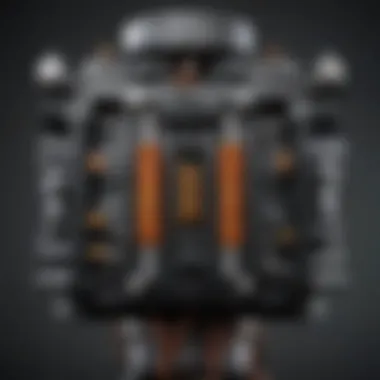
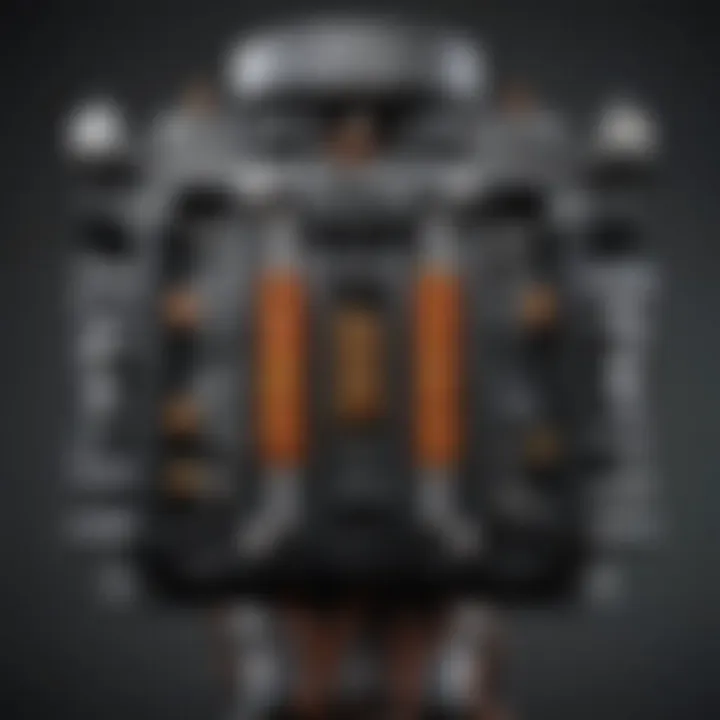
Intro
In the realm of electrical engineering, transformers hold a crucial position. These devices not only facilitate the transfer of electrical energy between circuits but also determine how effectively power is delivered for various applications. Understanding transformer calculations is essential for students, educators, researchers, and professionals alike, as these calculations underpin the functionality and design of transformers across numerous contexts.
This guide aims to break down the complexities of electrical transformer calculations, starting with basic principles and working its way through intricate configurations and performance optimization strategies. It is structured to provide clarity and depth, allowing readers to expand their knowledge and improve their practical skills in this vital area of study.
Recent Advances
Latest Discoveries
Recent research has shed light on new methodologies for enhancing transformer efficiency. For example, advancements in materials science have led to the development of core materials that drastically reduce energy losses. These innovations are not only beneficial from an engineering perspective but also contribute to reducing the carbon footprint associated with electrical energy transmission.
One particularly noteworthy area of study is the application of nanotechnology in transformers. By engineering materials at the molecular level, researchers can create more efficient magnetic properties, which allows for better operation under load conditions.
Technological Innovations
Transformers have increasingly integrated technology that enables smarter operation. Monitoring systems equipped with IoT (Internet of Things) capabilities allow for real-time data collection and diagnostic capabilities. This technological leap provides valuable insight into how transformers are functioning under various loads and helps in preemptively addressing maintenance needs.
For example, systems that analyze temperature and vibration data can alert managers about potential malfunctioning before they escalate into serious issues. This not only ensures reliability but also extends the lifespan of the equipment.
Methodology
Research Design
The methodology for understanding transformer calculations involves both theoretical and practical approaches. By balancing academic research with hands-on experiments, one can grasp the core principles while validating them through real-world applications.
Data Collection Techniques
Data collection for transformer performance can take various forms. Some techniques include:
- Simulation Software: Programs can simulate electrical circuits and the behavior of transformers under different conditions.
- Field Testing: Gathering data directly from operating transformers helps in understanding real-world performance and inefficiencies.
- Literature Review: Existing studies provide a wealth of information regarding calculation methods and modern trends in transformer technology.
Prologue to Electrical Transformers
Electrical transformers hold a pivotal role in the realm of power distribution and energy management. They not only facilitate the transfer of electrical energy between circuits but also serve to manage voltage levels across vast networks. This significance becomes especially clear when we consider modern society's reliance on electricity—from the simplest of household devices to complex industrial machinery. Understanding transformers allows professionals and students alike to enhance their knowledge of electrical systems, leading to improved efficiency and application.
The importance of transformers can be viewed through multiple lenses. For one, they are key to maintaining energy quality, minimizing losses during transmission, and providing the necessary adaptability to varying load requirements. Furthermore, various configurations of transformers allow for specific applications, whether in renewable energy integration or conventional grid systems.
In this exploration, we will uncover the definition, purpose, historical context, and a range of applied concepts surrounding transformers. By engaging deeply with these topics, readers will appreciate the foundational elements that contribute to both the theoretical and practical dimensions of transformer technology.
Definition and Purpose of Transformers
Transformers are electrical devices that adjust the voltage of alternating current (AC) in power systems. Their primary purpose is to step up or step down voltage levels while conserving energy rather than generating it anew. This operation is based on the principle of electromagnetic induction. When an electric current passes through the primary coil of a transformer, it generates a magnetic field that induces a voltage in the secondary coil.
There are a few key terms that often come up when discussing transformers:
- Primary coil: The winding in which the input voltage is applied.
- Secondary coil: The winding from which the output voltage is taken.
- Turn ratio: The ratio of the number of turns in the primary coil to those in the secondary coil, which helps to determine the voltage transformation.
"Transformers are a crucial element in the functioning of modern electrical systems, playing a significant role in effective energy distribution and load management."
The ability to adjust voltage levels means transformers can increase voltage for long-distance transmission—reducing energy loss—or lower it for safe and efficient use in homes and businesses. Additionally, they can isolate parts of a circuit, enhancing system safety.
Historical Development and Applications
The development of electrical transformers can be traced back to the late 19th century, a time of rapid advancements in electrical engineering. The invention of the transformer is attributed to several key figures, most notably the German engineer Otto von Guericke and later, the American inventor William Stanley. Stanley’s work led to the creation of the first practical transformer in 1885, which paved the way for the widespread adoption of electricity.
As electricity became integral to everyday life, transformers found numerous applications across industries. Not only are they commonly found in electrical substations, but they also serve industries like manufacturing, transport, and renewables. Moreover, transformers form a critical part of the growing wind and solar energy sectors. Here’s a quick look at their prominent applications:
- Power distribution: Ensuring the efficient transmission of electricity over long distances.
- Industrial processes: Adapting voltage levels to operate machinery and equipment.
- Renewable energy: Bridging generation and grid systems.
Understanding the historical evolution and applications of transformers is essential for recognizing their value in today's interconnected grid systems. As we delve into more technical calculations and concepts, we will see how they have adapted to meet emerging energy challenges.
Fundamental Principles of Transformer Operation
Understanding the fundamental principles of transformer operation is key to mastering the art of electrical transformer calculations. These principles underpin the efficiency and effectiveness of how transformers function, impacting various applications in the electrical engineering field. Mastery of these concepts allows students, researchers, and professionals to delve deeper into more complex transformer characteristics and computations.
Electromagnetic Induction
Electromagnetic induction is the cornerstone of transformer operation. This phenomenon, first researched by Michael Faraday, involves generating an electric current in a coil of wire through the presence of a changing magnetic field. In the context of transformers, this is typically realized through two coils wound around a magnetic core. When an alternating current flows through the primary coil, it creates a varying magnetic flux within the core.
The importance of this process cannot be overstated:
- Energy Transfer: The magnetic field generated around the primary coil can induce a voltage in the secondary coil through its changing barrier, enabling efficient energy transfer between different voltages.
- Isolation: It provides galvanic isolation between the high-voltage and low-voltage circuits, enhancing safety in electrical systems.
A practical example to illustrate this can be found when a transformer is utilized in a power distribution network, where high-voltage electricity is generated but needs to be converted to a safer voltage for residential usage. The electromagnetic induction within the transformer ensures this transition happens seamlessly.
The Role of Magnetic Flux
Magnetic flux is another crucial element in the operation of transformers. It refers to the amount of magnetic field passing through a given surface area. In transformers, it is vital because it dictates how effectively the energy is transferred between the primary and secondary coils.
Here’s how magnetic flux plays its role:
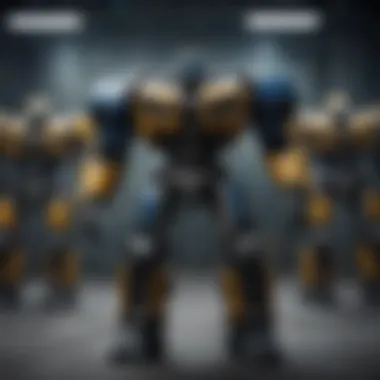
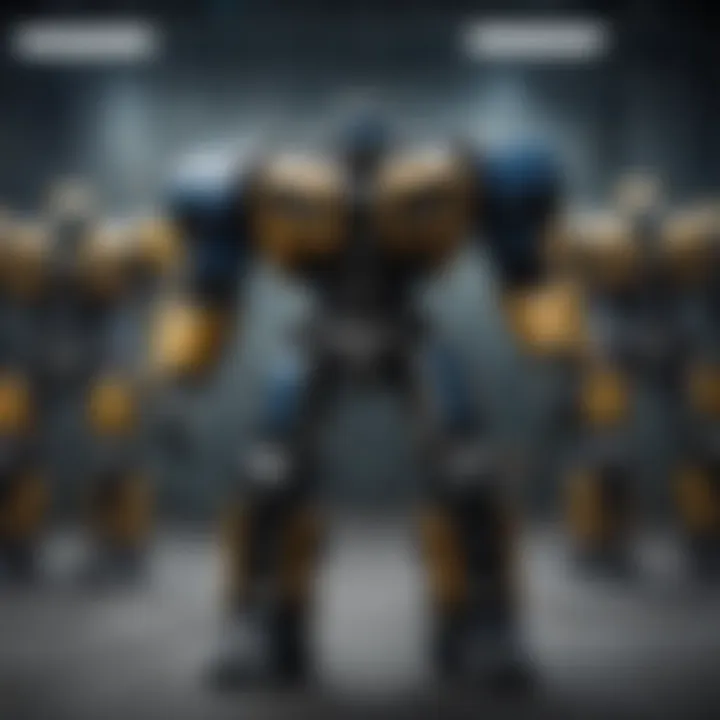
- Magnetic Core: The core, typically made from silicon steel or ferrite, guides the magnetic flux from the primary to the secondary coil. The configuration and material of the core can significantly affect transformer efficiency.
- Flux Linkage: For maximum efficiency, the magnetic flux should link as much as possible with the secondary winding. Any stray magnetic flux results in energy loss.
- Calculating Induced Voltage: The amount of magnetic flux directly influences the voltage induced in the secondary coil, proportional to the turns ratio of the coils.
An insightful observation is the relation of magnetic flux to losses in transformers. The better the flux management, the lower the energy losses through heat and other phenomena. This relationship emphasizes the meticulous engineering that goes into transformer design—an intricate dance between magnetic materials, coil turns, and operational conditions.
“Understanding electromagnetic induction and magnetic flux is like holding the keys to the engine room of electrical transformers. Without these, you’re merelylooking at the facade.”
Transformer Types and Configurations
Understanding transformer types and configurations is crucial for anyone delving into the complexities of electrical transformers. Different configurations serve distinct purposes, impacting everything from efficiency to transformer longevity. By grasping these various types, one can make informed decisions that enhance system performance and reliability.
Step-Up and Step-Down Transformers
Step-up and step-down transformers are the backbone of electrical power systems. These transformers adjust voltage levels to ensure that electrical energy can be transmitted efficiently over distances. A step-up transformer increases voltage from primary to secondary, while a step-down transformer does the opposite, reducing voltage to safer, usable levels.
The choice between step-up and step-down transformers can impact operational costs and safety. For instance, in a renewable energy scenario, you often require a step-up transformer to elevate voltage levels for transmission. Without this transformation, it would be challenging to deliver power over long distances without substantial losses. In contrast, when using electrical machinery, a step-down transformer is essential to bring high-voltage electricity down to a level that is safe for operation.
"Choosing the right transformer configuration is like picking the right tool for a job; it fundamentally affects your outcomes."
Single-Phase versus Three-Phase Transformers
Next, we consider single-phase and three-phase transformers. Each has its specific applications and characteristics that make them suitable for different scenarios. Single-phase transformers are often employed in residential or small commercial setups, mainly because they are simpler and generally less costly. On the flip side, three-phase transformers are more common in industrial settings where large amounts of power need to be transmitted efficiently.
The efficiency of a three-phase system is significantly better than that of single-phase systems, providing a smoother power flow and reducing losses. Hence, industries that require high loads tend to rely on three-phase transformers to improve their operations.
Autotransformers
Autotransformers represent another layer of complexity in transformer discussions. Unlike standard transformers, autotransformers share part of their winding between the primary and secondary circuits, allowing them to be more compact and efficient for certain tasks. They are particularly useful when the voltage difference between input and output is small.
For example, autotransformers often find their niches in applications like motor starting and voltage regulation. However, while they offer significant benefits like reduced material costs, the electrical isolation between circuits is less than that found in conventional transformers. Hence, careful consideration of these aspects is paramount when selecting an autotransformer for a particular application.
Basic Transformer Calculations
Understanding basic transformer calculations is fundamental for anyone involved in electrical engineering. This aspect of transformer technology lays the groundwork for analyzing how transformers operate, ensuring they meet the required specifications for various applications. This knowledge isn’t just academic; it’s crucial in real-world scenarios where reliability and efficiency are paramount. Basic calculations can aid in choosing the right transformer size, ensuring that it fulfills the load requirements without unnecessary excess, thus optimizing performance and energy usage.
Transformer Turn Ratio
The transformer turn ratio is a vital calculation in transformer operations. It refers to the ratio of the number of turns in the primary coil to the number of turns in the secondary coil. A simple formula captures this:
[ \textTurn Ratio = \fracN_pN_s ]\
Where:
- ( N_p ) = Number of turns in the primary coil
- ( N_s ) = Number of turns in the secondary coil
This ratio directly influences the voltage transformation between input and output. For instance, if a transformer has a turn ratio of 2:1, it means the voltage will be halved from primary to secondary. In practice, this is essential for applications like power distribution where voltages need to be stepped down for safety and usability. Miscalculating this can lead to equipment damage or ineffective operation.
Voltage and Current Relationships
When discussing transformers, the relationships between voltage and current bear significant importance. The key principle at play is that while voltage can change, the power remains constant (ideally, neglecting losses). The relevant formulas breakdown as follows:
[ P = V_p \cdot I_p = V_s \cdot I_s ]\
Where:
- ( P ) = Power
- ( V ) = Voltage
- ( I ) = Current
- Subscripts ( p ) and ( s ) denote primary and secondary respectively.
This means that if the voltage increases, the current must decrease accordingly to maintain the same power level. This interplay is crucial in designing circuits and ensuring that the transformer can handle required loads without overheating or sustaining damage.
Power Measurements
Power measurements in transformers are essential to quantify and verify the energy efficiency and operational effectiveness of the system. The real power, reactive power, and apparent power all come into play here, often represented by the formulas:
[ S = V imes I^* ]
Where ( S ) is the apparent power which is seen in volt-amperes (VA).
In practical applications, understanding these measurements helps engineers design systems that resist theft, waste, and enhance performance overall. With the goal of achieving maximum efficiency in every transformer deployment, power calculations become not just necessary but essential.
"An understanding of basic transformer calculations is not just academic; it is the foundation of reliability and performance in electrical engineering."
Additionally, knowing how to utilize power factor corrections and other metrics keeps systems running smoothly, bolstering the overall efficacy of electrical installations.
By grasping these basic fundamentals, professionals can tackle more advanced transformer concepts with confidence. Topics like load balancing, efficiency metrics, and system design ultimately hinge on a firm understanding of these preliminary calculations.
Efficiency and Losses in Transformers
When it comes to the operational prowess of transformers, efficiency is the name of the game. Understanding how transformer efficiency affects overall performance is pivotal, not just for engineers but for anyone involved in electrical systems. Transformers must function to reduce energy losses as much as possible because inefficiency can lead to heat generation and subsequent damage. Moreover, analyzing these losses allows for the selection of more suitable transformers for specific applications, optimizing both cost and longevity.
A high-efficiency transformer can not only save energy, reducing operational costs but also decrease environmental impact. In contexts where large amounts of power are transferred, even a slight increase in efficiency can translate to significant savings over time. Thus, evaluating transformer efficiency and understanding where losses occur is crucial.
Types of Losses: Copper and Core Losses
In the world of transformers, losses primarily boil down to two categories: copper losses and core losses.
- Copper Losses: These losses occur due to the resistance of the windings when current flows through them. Essentially, it’s like water flowing through a pipe; the narrower the pipe, the more pressure drops. In electrical terms, the amount of heat generated depends on the load.'Copper losses' can be calculated using the formula:[ P_cu = I^2 R ]Where:Taking steps to use better conductors with lower resistance can significantly mitigate these losses.
- Core Losses: Contrarily, core losses are somewhat of a silent killer in transformer inefficiency. They stem from the alternating magnetic field present in the core. This involves two components: hysteresis loss and eddy current loss.
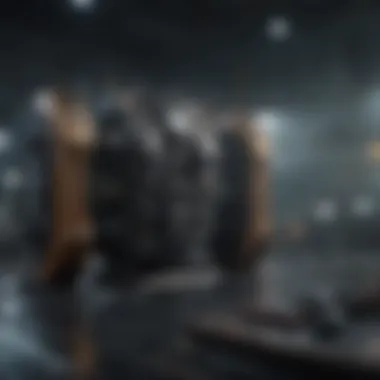
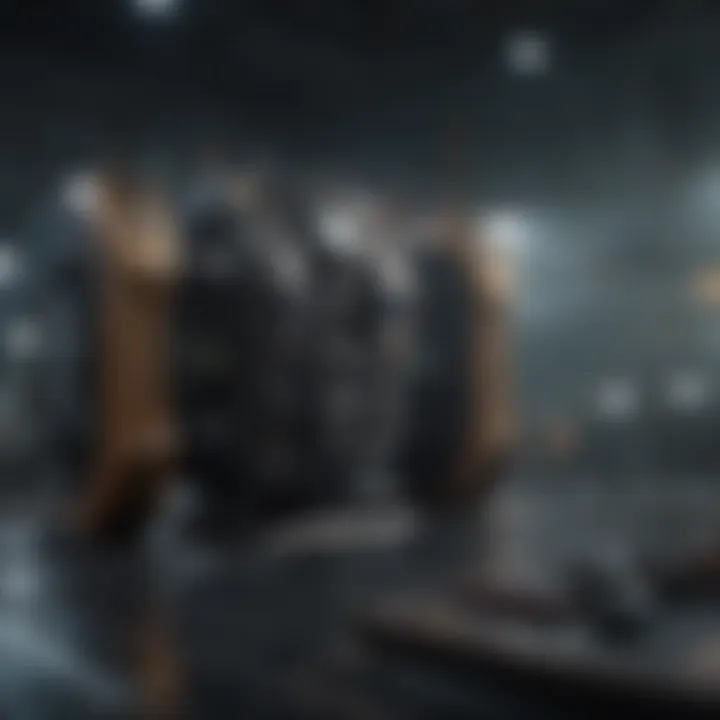
- ( P_cu ) = Power loss due to resistance
- ( I ) = Current
- ( R ) = Resistance of the winding
- Hysteresis Loss: This occurs due to the constant magnetization and demagnetization of the core materials as the current alternates. The loss directly correlates with the frequency of the current and the properties of the core material.
- Eddy Current Loss: Every transformer core experiences this as well. The alternating magnetic field induces currents within the core itself, which then generate heat. Using laminated core structures can help to minimize these eddy currents, making the design more efficient.
Understanding these two loss types and their contributions to the overall inefficiency of the transformer sets the stage for calculating actual operational effectiveness.
Calculating Efficiency
Calculating transformer efficiency isn't just a box to check; it's a necessary practice for ensuring optimal system design and profitability. The efficiency of a transformer can be expressed by the following formula:
Where:
- ( P_out ) = Output power (useful)
- ( P_in ) = Input power (total)In practical terms, efficiency can be visually represented in a loss curve, showing how losses vary with load. Typically, you can expect transformers to operate at optimal efficiency levels between 75% and 98%. Therefore, knowing exactly where your transformer sits on that line is crucial in applications, such as substations and renewable energy setups, where balancing load and performance is essential.
An efficient transformer can mean the difference between energy wasted and energy optimally utilized.
To improve efficiency, several strategies can be employed:
- Use higher quality materials with better magnetic properties.
- Implement modern designs tailored to specific requirements.
- Regularly perform maintenance checks to assess operational health.
By grasping the mechanisms of efficiency and losses in transformers, one can make informed decisions that enhance system reliability and performance while also being cost-effective.
Load Calculations
Load calculations are a critical component in transformer design and analysis. Understanding load requirements ensures that transformers function optimally and meet the demands of electrical systems. It is crucial to properly assess potential loads to maintain performance, prevent overheating, and extend the lifetime of the transformer.
Accurate load calculations allow designers to anticipate changes in power usage, which is especially important in dynamic environments such as industrial applications or large commercial buildings. By evaluating load requirements, engineers can select appropriate transformer specifications that not only address current demands but also provide room for future growth.
Determining Load Requirements
Determining load requirements involves analyzing the total electrical load that the transformer will need to support. This analysis should consider various factors:
- Type of Load: Loads can be classified into resistive, inductive, and capacitive types. Each type has different characteristics and impacts on transformer performance.
- Peak Demand: This is the highest level of load anticipated over a specific time period. Understanding peak demand helps ensure that the transformer can handle maximum loads without strain.
- Load Factor: This is a measure of the actual load divided by the peak load during a specified period. It indicates how heavily the system is used over time.
Employing these factors in calculations can give a comprehensive view of load behavior, essential for making informed decisions about transformer sizing and selection.
Sizing Transformers for Load
The process of sizing transformers is directly related to the load requirements identified earlier. An undersized transformer can lead to overheating and premature failure, while an oversized unit may operate inefficiently, increasing operational costs.
When sizing a transformer, several key considerations come into play:
- Calculate the Total Load: Sum the wattages of all devices that the transformer will supply. This gives you the baseline capacity needed.
- Add Safety Margins: Common practice suggests adding a safety margin of 20-30% to account for future expansion or unexpected load increases.
- Consider Continuous and Intermittent Loads: Ensure to distinguish between continuous loads and those that are intermittent. Continuous loads are the ones that are present most of the time, while intermittent loads may spike occasionally.
By effectively sizing transformers based on thorough load calculations, one can not only optimize performance but also ensure energy efficiency and sustainability in electrical systems. An investment in diligent analysis during the design phase often paves the way for smoother operations and cost savings down the line.
Short-Circuit and Open-Circuit Tests
Importance of Testing
In the realm of electrical transformers, the importance of rigorous testing cannot be overstated. Both short-circuit and open-circuit tests are pivotal for assessing the performance and reliability of transformers. These tests help engineers ensure that transformers function efficiently and safely under expected operating conditions.
Why do we test?
- Performance Validation: Through testing, technicians validate the transformer's ability to handle specified loads without overheating or failing.
- Safety Assurance: Identifying any potential failure modes in a controlled environment prevents accidents and enhances operational safety.
- Efficiency Measurement: By comparing the input to the output under various conditions, one can deduce the transformer's efficiency and make necessary adjustments.
By conducting these tests, engineers gain valuable insights, ensuring that the transformers not only perform as expected but also adhere to the relevant standards and regulations.
Conducting Short-Circuit Tests
Short-circuit tests provide a mechanism for assessing a transformer's short-circuit impedance and stray losses. In essence, this test involves connecting the primary winding of the transformer to a power source while the secondary winding is shorted. The main goal here is to measure the current flowing through the winding when subjected to a short circuit.
Here's a stepwise approach for conducting this test:
- Setup: Assemble the transformer with appropriate safety equipment. Ensure the area is clear and that you’re using suitable voltage ratings.
- Shorting: Connect the secondary side of the transformer to a conductive material to create a short circuit.
- Power Input: Gradually apply voltage to the primary winding while monitoring the current.
- Measurement: Record the values of voltage and current. This data helps in calculating the short-circuit impedance.
- Analysis: Analyze the data to assess losses, ensuring it aligns with manufacturer specifications.
This method not only helps verify that the transformer meets design specifications but also helps in identifying any irregularities that could indicate underlying issues.
Conducting Open-Circuit Tests
Open-circuit tests are equally crucial and typically performed on transformers when the secondary winding is left open, and only the primary winding is energized. This method helps determine core losses and no-load current, offering a clear picture of the transformer's operational efficiency.
Here's how the open-circuit test procedure generally plays out:
- Prepare the Test Bed: Similar to short-circuit tests, set up the transformer safely and ensure clear working conditions.
- Open Secondary: Ensure the secondary winding is not connected to any external load or closed circuit.
- Apply Voltage: Connect voltage to the primary winding.
- Measurements: Carefully take note of the voltage, current, and wattage readings at this stage. This data is critical for calculating core losses and determining the transformer's efficiency at idle.
- Evaluative Steps: Analyze this data to glean insights about the core’s magnetic properties and to check for any potential magnetic saturation issues.
Testing transforms performance and aids in early detection of possible complications, something especially critical in high-stakes environments such as power stations and industrial plants.
"Thorough testing is not just a box to tick; it's a foundation for reliable energy management."
Thus, both short-circuit and open-circuit tests are integral components in the lifecycle of transformer evaluation, ensuring they function optimally while mitigating risks associated with electrical failures.
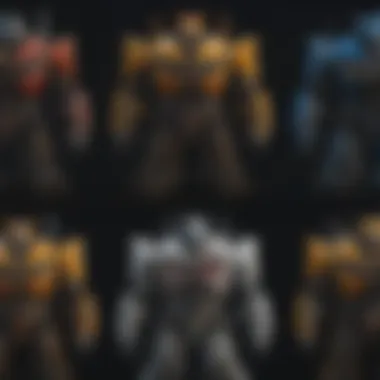
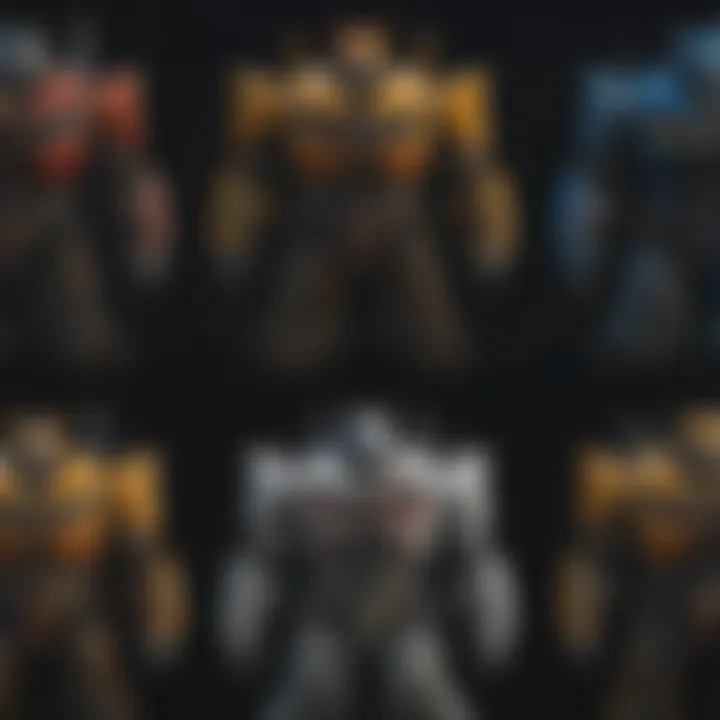
Real-World Applications of Transformer Calculations
The relevance of transformer calculations transcends academic settings, making their real-world applications essential for various industries. Understanding these practical uses not only enhances engineers’ and technicians’ ability to design and implement effective electrical systems, but also contributes to efficiency in power distribution and various technological advancements. Let’s uncover these real-world scenarios in detail.
Substation Design Considerations
Designing a substation is more than just assembling transformers; it involves a meticulous approach to calculations that ensure reliability and efficiency. Here, transformers are pivotal. Selecting the right transformer necessitates an acute understanding of load calculations, as transformers must meet specific voltage and current requirements. Moreover, the application of transformer turn ratios impacts the distribution system's effectiveness.
Substation designers must consider several aspects:
- Load Requirements: Estimating the total load that the substation will support helps in choosing the appropriate transformer size, avoiding overloading or underutilization.
- Voltage Levels: Decisions about nominal voltage levels are pivotal, informing the design calculations for transformers to ensure a seamless transformation of voltages.
- Short-Circuit Ratings: Transformers must withstand potential fault currents, thus calculations determining thermal and mechanical stresses are crucial.
Incorporating these calculations not only aids in enhancing the substation’s performance but also ensures safety and longevity of equipment. As one expert noted, "A well-designed substation is like a well-tuned engine; precision leads to performance."
Renewable Energy Integration
The rise of renewable energy sources brings a new layer of complexity to transformer calculations. As solar panels and wind turbines become ubiquitous, transformers play a crucial role in integrating these alternative energy sources into the existing grid. The fluctuating nature of renewable energy generation necessitates precise calculations to adapt transformer operations.
Key considerations include:
- Voltage Regulation: Proper transformer selection and calculating reactive power are essential to maintain voltage stability and support intermittent renewable sources.
- Interconnection Costs: Here, calculating the transformer sizes based on generation capacity and future expansions is vital.
- Harmonic Distortion: Renewable sources can introduce harmonics, which affect transformer efficiency. Evaluating transformers for harmonic mitigation is crucial for optimal system performance.
Transformers effectively enable smoother transitions between renewable and conventional energy, significantly enhancing grid reliability. The proper integration of renewable energy through accurate calculations helps the shift towards sustainable energy, benefiting both consumers and the environment.
Industrial Applications
Industrial settings utilize transformers for a plethora of functions, where calculations customarily translate to enhanced productivity and safety. From process plants to manufacturing facilities, transformers are central to various operations.
Some prominent applications include:
- Voltage Transformation: Industrial operations often require step-down transformers. For example, machinery running on lower voltages needs a transformer to ensure compatibility and efficiency.
- Load Balancing: In heavy industrial applications, transformers are employed to manage load distribution across three-phase systems, preventing overloads and ensuring equipment reliability.
- Arc Furnaces: In steel and metal production, specialized transformers are used to supply the significant power demands of electric arc furnaces, where precise calculations determine their operational efficiency.
Conclusively, the successful integration of transformers in industrial applications relies heavily on thorough and meticulous calculations.Transformers not only enhance operational efficiency but also ensure that industrial sectors meet production demands safely and effectively.
No doubt, the realm of transformer calculations stretches far beyond theory. Their implications in designing substations, accommodating renewable energy, and optimizing industrial processes highlight the need for precision in every calculation.
"In engineering, it's the careful calculations that pave the way for innovation."
Through a deeper understanding of these real-world applications, professionals can better navigate the complexities involved, leading to smarter, more efficient electrical systems.
Advanced Concepts in Transformer Calculations
In the realm of electrical transformers, the discussion naturally gravitates towards more intricate and nuanced ideas that have a profound effect on the overall functionality and efficiency of these devices. Advanced concepts serve to bridge the gap between basic operational principles and the real-world challenges faced in engineering applications. Understanding these concepts, such as harmonics and transient phenomena, arms engineers and scholars with the tools necessary to optimize transformer performance, reduce losses, and mitigate issues associated with power quality.
The significance of delving into these advanced calculations lies not only in theoretical understanding but also in practical implications. Engineers tasked with designing systems must account for how various electrical disturbances affect transformer operation. Moreover, as the integration of renewable energy sources increases, the complexity of these interactions becomes even more critical. Thus, mastering advanced transformer concepts isn't just important for academic advancement; it's essential for crafting efficient, reliable electrical systems.
Harmonics and Their Impact
Harmonics represent a critical challenge in power systems, often arising from non-linear loads such as LED lighting and variable frequency drives. These higher frequency components can distort the voltage and current waveforms in electrical systems, leading to inefficiencies and potential equipment damage.
The impact of harmonics on transformers is multifaceted:
- Heating: Transformers are susceptible to excessive heating due to harmonic currents. This can lead to premature aging of insulation and reduced lifespan.
- Losses: It has been established that harmonic currents lead to increased losses, hence decreasing the overall efficiency.
- Resonance: There is a risk of resonance phenomena that can amplify voltage levels dangerously high.
To grasp the impact of harmonics, the method of harmonic analysis is implemented, often employing Fourier series to breakdown complex waveforms into their fundamental frequencies and their harmonics. Understanding the Total Harmonic Distortion (THD) is crucial here. A lower THD typically indicates better power quality, which aids in reducing operational costs.
By utilizing simulation software, such as MATLAB, engineers can model the effects of harmonics and design transformers capable of mitigating these harmful impacts.
Transient Analysis
Transient analysis zeroes in on the behavior of transformers during sudden changes in operating conditions—such as switching events or faults. This concept is pivotal for safeguarding transformer integrity and ensuring stability in power systems.
Transients can manifest as voltage spikes or current surges, and their analysis involves evaluating:
- Inrush Currents: Upon energization, transformers can draw current that is several times greater than the nominal rating, potentially leading to mechanical stress and overheating.
- Fault Conditions: Understanding how transformers react during short circuits helps engineers design protective measures to limit damage.
- Response Time: Evaluating the speed with which transformers return to steady operation after changes is critical for system design.
Mathematically, transient behavior is addressed using differential equations that describe the electrical dynamics of transformers. Engineers often utilize software to visualize these transients and develop strategies to mitigate their effects.
"Mastery of advanced transformer calculations isn't merely academic; it’s about crafting tomorrow's reliable electrical systems."
By integrating harmonics and transient analysis into transformer design, engineers can devise systems that are not only robust but also capable of adapting to the ever-evolving landscape of modern electricity demands. An awareness of these concepts opens the door to innovations in transformer technology. They become stepping stones for advancing efficiency and enhancing the reliability of power systems.
Finale
The conclusion serves as a pivotal wrap-up of the themes and discussions presented in this article about electrical transformer calculations. It underscores the immense significance of transformers in both theoretical studies and practical applications. Understanding the mechanics of transformers—including their design, performance, and operational principles—is not just for the realm of academia but extends to real-life applications impacting various sectors, from energy distribution to industrial processes.
By evaluating the essential calculations and conducting tests, engineers and technicians can effectively ensure that transformers operate safely and efficiently. The complexities involved can be daunting; yet grasping these concepts can yield substantial benefits in engineering outcomes.
Summary of Key Points
In reviewing the entirety of this guide, several key points emerge, which are paramount for readers:
- Definition and Functionality: Electrical transformers function by transferring electrical energy between circuits through electromagnetic induction.
- Types of Transformers: A range of transformers exist, each suited for specific applications like step-up, step-down, and autotransformers.
- Key Calculations: Understanding turn ratios, voltage and current relationships, and power measurements is essential for effective transformer design and implementation.
- Load Calculations: Proper load assessment and transformer sizing are critical in ensuring optimal performance.
- Testing Procedures: Implementing short-circuit and open-circuit tests can reveal valuable information about the transformer's operational health.
- Efficiency Factors: Recognizing and calculating losses helps in enhancing transformer efficiency, crucial for reducing operational costs.
- Advanced Considerations: Topics such as harmonics and transient phenomena significantly influence transformer performance, necessitating sophisticated analysis.
Future Trends in Transformer Technology
The advancements in transformer technology are not standing still. Several emerging trends indicate where the industry is headed:
- Smart Transformers: The rise of digitalization has led to the development of smart transformers equipped with IoT technology, providing real-time data and remote monitoring capabilities.
- Integration with Renewables: There's a growing focus on creating transformers capable of efficiently handling the fluctuating nature of renewable energy sources, like solar and wind.
- Sustainability Efforts: As the world pivot towards greener technologies, innovations aimed at minimizing the environmental impact of transformer production and operation are gaining traction.
- High-Temperature Superconductors: Research into superconducting transformers could revolutionize efficiency, allowing for significant reduction of energy losses compared to conventional transformers.
- Electromagnetic Compatibility: Future designs are increasingly concerned with electromagnetic interference, necessitating designs that sidestep adverse effects on sensitive electronics.
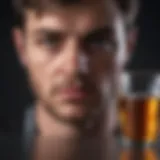
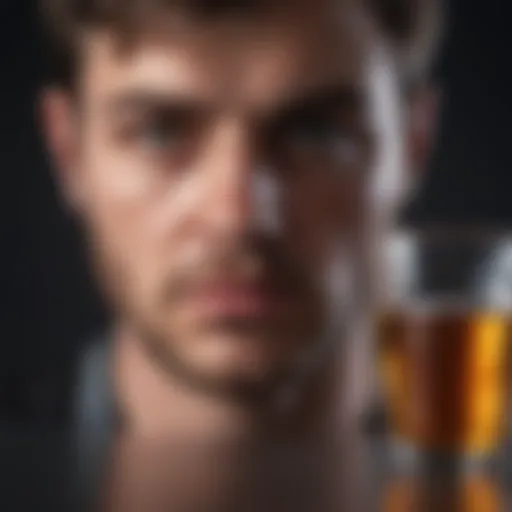