Human Genome Diseases: Insights and Innovations
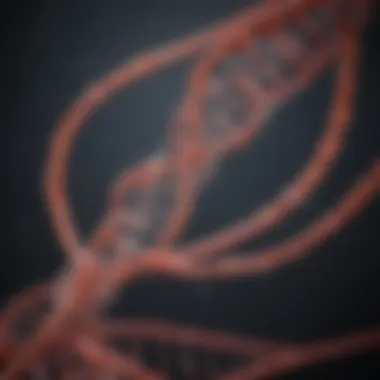
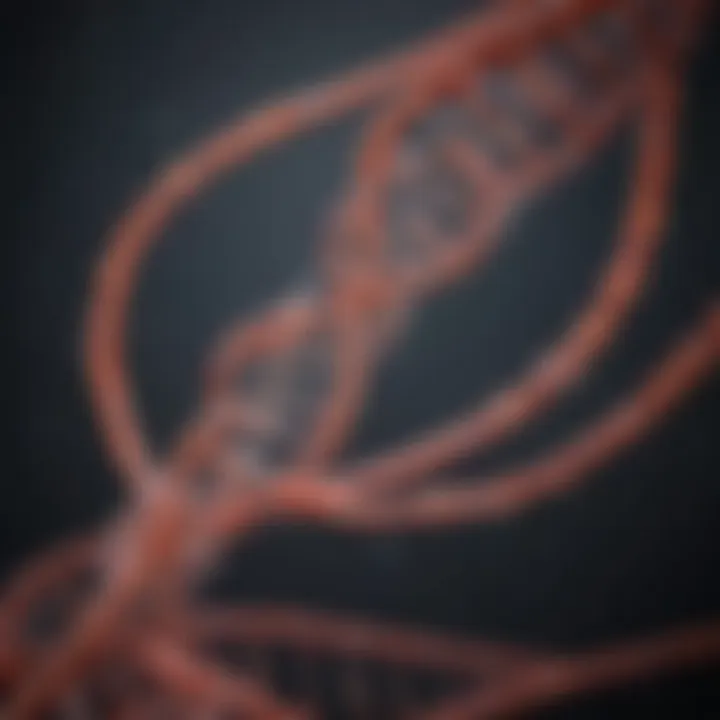
Intro
The human genome is a complex blueprint that underpins the biology of each individual. Understanding how this intricate structure relates to diseases has emerged as a critical area of study within genomics. Genetic disorders often stem from mutations or variations in the genome, leading to a wide array of health issues. This exploration not only enhances medical knowledge but also informs potential treatments, preventive measures, and ethical considerations surrounding genetic research. As we delve into this analysis, we will uncover how genomic variations impact various diseases, the significance of advancements in genomics, and the implications for future medical practices.
Recent Advances
Latest Discoveries
Recent findings in genomics have significantly reshaped our understanding of genetic diseases. For instance, researchers have identified specific gene mutations linked to conditions like cystic fibrosis and Huntingtonβs disease. Understanding these links allows for faster diagnosis and tailored treatment approaches.
Furthermore, studies show that non-coding regions of DNA, once thought to be "junk DNA," play crucial roles in gene regulation and disease susceptibility. These discoveries challenge previous perceptions and open new avenues for research.
Technological Innovations
Innovations in technology have propelled genomics forward. Next-generation sequencing (NGS) enables researchers to read entire genomes quickly and with impressive accuracy. This technology is essential for discovering new genetic variants associated with diseases.
Complementing NGS, CRISPR-Cas9 technology allows for precise editing of genes. This advance offers potential therapies for genetic disorders by correcting mutations directly at the DNA level. These innovations set the stage for revolutionary changes in how we approach diagnosis and treatment of diseases.
Methodology
Research Design
This comprehensive analysis relies on a multidisciplinary approach, combining insights from genetics, molecular biology, and bioinformatics. The research design involves reviewing existing literature and recent studies to provide a well-rounded perspective.
Data Collection Techniques
Data collection for this analysis encompasses a variety of techniques. Genetic databases such as dbSNP and ClinVar provide valuable information on genetic variants. Additionally, cohorts from genomic studies like the 100,000 Genomes Project contribute data on population-specific variations and their health impacts.
The integration of findings from clinical trials and observational studies enhances the robustness of the conclusions drawn.
Understanding the complex relationship between genomic variations and diseases is fundamental to advancing personalized medicine.
This article will synthesize the information presented throughout, offering insights that clarify the interplay between the human genome and various diseases while contemplating future directions in this vital field.
Prolusion to Human Genome Diseases
The exploration of human genome diseases is essential as it illuminates the intricate relationship between our genetic code and various health conditions. From commonplace disorders to rare diseases, understanding how genomic variations influence health is paramount. Such knowledge informs not only the diagnosis and treatment but also preventive strategies, thereby potentially reducing the burden of these diseases on individuals and healthcare systems.
Definition and Scope
Human genome diseases can be defined as disorders caused by abnormalities in the genome. These abnormalities can occur due to mutations, duplications, deletions, or other changes in the DNA sequence. The scope covers a vast array of conditions, from simple monogenic disorders like cystic fibrosis to complex multifactorial diseases such as diabetes and heart disease. Genomic research encompasses both these types of disorders, addressing how genetic factors interact with environmental elements, leading to the manifestation of a disease.
Importance of Genomic Research
The significance of genomic research cannot be overstated. This field furthers our understanding of fundamental biological processes. Here are some key points underscoring its importance:
- Advancement in Treatment: Research sheds light on targeted therapies, such as those used in cancer treatment. This allows a more personalized approach to healthcare.
- Early Diagnosis: With the advancement of genetic testing, many diseases can be diagnosed earlier, which can significantly improve outcomes.
- Preventive Strategies: Understanding genetic predispositions can support preventive measures, helping reduce the incidence of diseases.
- Ethical Perspectives: As the landscape of genomics grows, ethical considerations arise, pushing for policies that protect individuals' rights.
Through genomic research, we can not only address existing health challenges but also pave the way for innovative solutions in personalized medicine.
Genetic Disorders: An Overview
Understanding genetic disorders is fundamental in the context of human health and disease. This overview serves as a foundation for exploring various complexities within the human genome. Genetic disorders can span a spectrum, affecting an individual's health in different ways, thus highlighting the need for informed research in this field. When addressing genetic diseases, one must acknowledge their multifaceted nature, potential implications on populations, and influence on health policies.
Types of Genetic Disorders
Monogenic Disorders
Monogenic disorders stem from mutations in a single gene. This clear inheritance pattern distinguishes them from other types of genetic conditions. The simplicity related to a single-gene mutation facilitates targeted research and understanding of specific diseases. Examples include cystic fibrosis and sickle cell disease. Their deterministic nature allows for more direct correlations between genotype and phenotype.
The key characteristic of monogenic disorders is their straightforward genetic basis. This clarity allows researchers to pinpoint the exact mutations contributing to these diseases. For the discussion in this article, monogenic disorders are beneficial due to the wealth of research available, often leading to effective diagnostics and treatments. Yet, they can also be limiting; as they are inherently linked to specific genetic mutations, their scope is narrower compared to multifactorial disorders.
Multifactorial Disorders
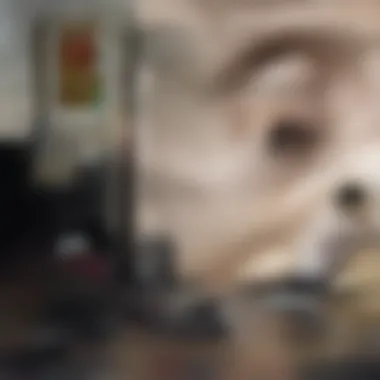
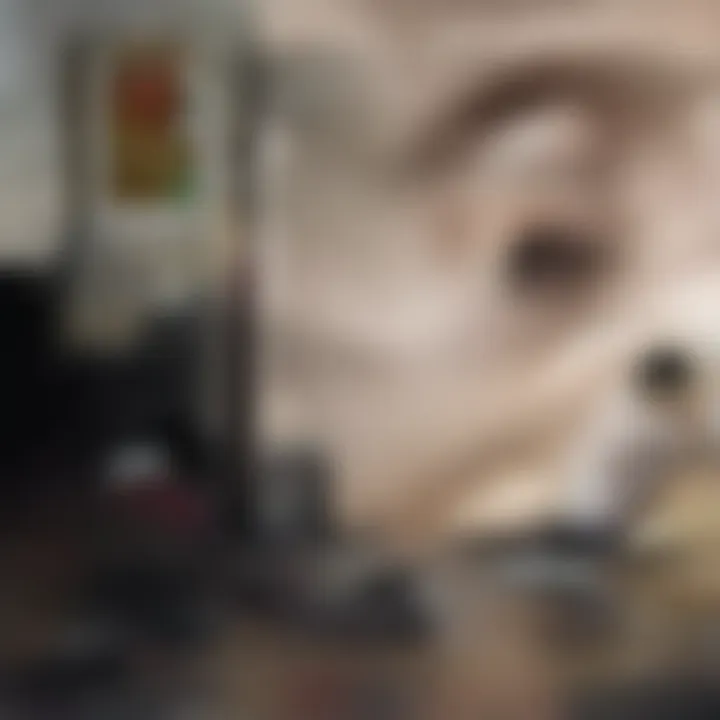
Multifactorial disorders involve multiple genes alongside environmental factors. Conditions such as diabetes and heart disease exemplify this category, reflecting the interaction between genetics and lifestyle or environmental impacts. This complex interplay makes multifactorial disorders particularly interesting yet challenging to study. The presence of multiple risk factors complicates the identification of precise genetic contributions.
This category's defining feature is its intricate etiology. Understanding multifactorial disorders contributes to a more comprehensive view of disease causation. Their complex nature underscores their relevance to public health discussions since they usually have broader impacts on populations. However, this complexity can also hinder targeted treatment approaches and complicate early diagnosis.
Mitochondrial Disorders
Mitochondrial disorders arise from defects in the mitochondria, the energy-producing structures in cells. Unique to these disorders is the maternal inheritance pattern, given that mitochondria are passed down from the mother. Diseases such as Leber's hereditary optic neuropathy showcase the implications of mitochondrial dysfunction.
The distinctive aspect of mitochondrial disorders is their systems-wide impact on energy metabolism. This feature reveals their significant implications for various organs and systems, often leading to diverse clinical manifestations. Mitochondrial disorders enrich the discourse on genetic diseases, as they illustrate how inherited genetic errors can profoundly affect cellular function. However, their varied expressions can pose challenges in both diagnosis and management, reflecting the need for continued research in therapeutic interventions.
Prevalence and Impact
The prevalence of genetic disorders varies considerably across populations and regions. An estimated 1 in 200 people have some form of genetic disorder. The impact on individuals, families, and healthcare systems is extensive, ranging from emotional strain to substantial financial burdens.
Genetic disorders have broad implications, not only for the individuals affected but also for healthcare infrastructure.
Moreover, understanding the prevalence and impact of these disorders facilitates public health efforts tailored to specific populations. Acknowledging the economic and social consequences further reinforces the necessity for genomic research and effective management strategies. This comprehensive awareness will assist in addressing genetic disorders promptly and effectively.
Mechanisms of Genetic Diseases
Understanding the mechanisms of genetic diseases is pivotal for comprehending how genome variations influence health. These mechanisms not only reveal the root causes of various hereditary conditions but also open avenues for targeted treatment strategies. Genetic disorders arise due to alterations in DNA, affecting single genes or interactions between multiple genes. This section will detail the types of mutations and mechanisms of inheritance, as well as the concept of genomic instability, each critical to genetic disease manifestations.
Mutations and Variations
Types of Mutations
Types of mutations define how genetic information changes within the human genome. They can be classified into various categories like point mutations, insertions, deletions, and duplications. Each of these types has distinct impacts on gene function.
- Point mutations involve the alteration of a single nucleotide base.
- Insertions add extra base pairs into a gene.
- Deletions remove some segments of DNA.
- Duplications replicate sections of DNA, increasing the gene dosage.
The critical aspect of types of mutations is their varying role in diseases. For example, a single point mutation in the HBB gene can lead to sickle cell anemia, showcasing how one small change can have significant consequences. Understanding these mutations is beneficial as it allows for precise diagnosis and tailored therapies. Each type presents unique challenges. While point mutations are often easy to identify, large deletions may evade standard detection methods.
Mechanisms of Inheritance
The mechanisms of inheritance are essential for understanding how genetic traits and disorders pass from parents to offspring. Classic Mendelian inheritance describes two primary patterns: dominant and recessive transmission. Each defines how a disorder might manifest based on the genetic makeup of family members.
- In dominant inheritance, only one mutated copy of a gene is sufficient for a trait or disorder to manifest.
- In recessive inheritance, two copies are necessary for the trait to be expressed.
This characteristic of inheritance shapes the counseling for families at risk and provides insight into potential outcomes for offspring. For instance, conditions like Huntington's disease follow dominant inheritance, thus often appearing in every generation. Understanding these patterns benefits genetic counseling and informs patients about their risks.
Genomic Instability
Genomic instability refers to an increased frequency of mutations within the genome. This phenomenon can lead to cancer and various heritable diseases. Factors contributing to genomic instability include environmental exposures, errors during DNA replication, and faulty repair mechanisms.
Genomic instability presents unique challenges in disease management and treatment. It poses risks for both the individual and future generations and adds complexity to existing genetic disorders. In various cancers, high levels of instability can result in significant tumor heterogeneity, complicating treatment regimens.
"Understanding genetic mechanisms provides foundational knowledge for innovations in both diagnosis and targeted therapies."
By emphasizing these mechanisms, the article illustrates the intricate relationship between genomic variations and disease outcomes. This framework assists in shaping future research directions and therapeutic innovations.
Advances in Genomic Research
Advancements in genomic research represent a cornerstone of contemporary medicine, allowing for a comprehensive approach to understanding human health and diseases. This section emphasizes the role of cutting-edge technologies and methodologies that have transformed the field. These advances enhance not only our understanding of genetic diseases but also their diagnosis and treatment, offering significant benefits to patients and healthcare providers alike.
Next-Generation Sequencing
Next-Generation Sequencing (NGS) is a revolutionary technology that has changed the landscape of genomics. It allows researchers to sequence millions of DNA strands simultaneously, dramatically increasing the speed and accuracy of genetic analyses. NGS enables the identification of genetic variations that contribute to diseases at an unprecedented scale.
The benefits of NGS are manifold:
- Cost-Effectiveness: Compared to traditional sequencing techniques, NGS reduces the cost of sequencing a complete genome.
- Comprehensive Data: Its capacity to provide detailed information about entire genomes or specific regions makes it invaluable for both rare and common diseases.
- Rapid Turnaround: Testing that once took weeks or months can now be completed in days, enhancing clinical decision-making.
Moreover, NGS facilitates personalized medicine. It allows for tailoring treatment strategies based on a patient's unique genetic profile, leading to improved outcomes. However, challenges remain, including data interpretation complexities and the ethical implications of genetic information management.
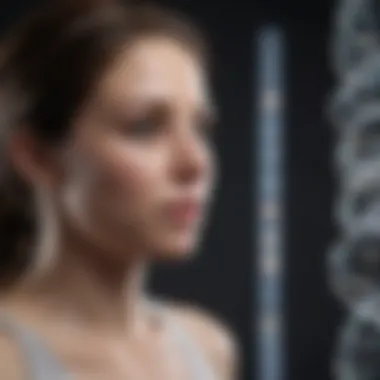
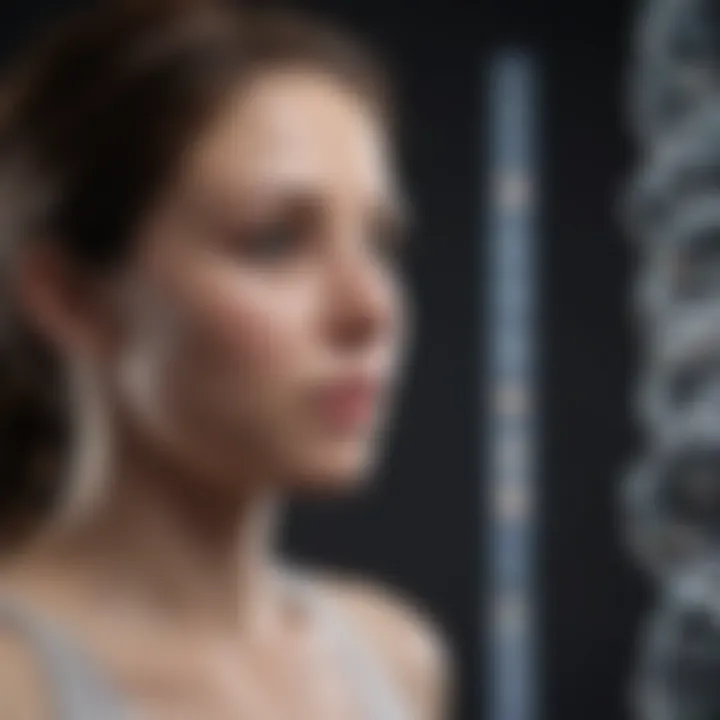
Genome-Wide Association Studies (GWAS)
Genome-Wide Association Studies (GWAS) provide insights into the genetic basis of traits and diseases by analyzing the entire genome for associations with specific conditions. These studies have identified numerous genetic loci associated with common diseases like diabetes, heart disease, and various cancers. GWAS contribute to our understanding of both the genetic predisposition to diseases and the biological mechanisms involved.
The key aspects of GWAS include:
- Large Cohorts: Studies typically involve large populations, increasing the reliability of results and ensuring robust conclusions.
- Discovery of Risk Factors: By pinpointing genetic variations linked to diseases, GWAS reveal potential biomarkers for risk assessment and early detection.
- Integration with Functional Studies: Findings from GWAS can guide further research by prompting functional validation studies, which investigate how identified variations affect biological processes.
However, GWAS are not without limitations. The complexity of polygenic traits means that identified associations may only explain a portion of the heritability of diseases. Furthermore, ethical considerations must be addressed, particularly concerning the handling and storage of genetic data.
Advances in genomic research, particularly through NGS and GWAS, provide a framework for understanding diseases and tailoring precise treatment options. Continued exploration in this field promises to enhance health outcomes and address critical public health challenges.
Diagnosis of Genetic Diseases
The diagnosis of genetic diseases is crucial for several reasons. Firstly, it enables accurate identification of disorders that arise from genetic anomalies, leading to targeted management strategies. Secondly, recognizing the presence of a genetic disease informs family planning decisions, especially for individuals with known hereditary conditions. Thirdly, being aware of genetic predispositions allows for early intervention and patient education, increasing the chances of effective treatment. Without proper diagnosis, patients may struggle with misdiagnoses, leading to inappropriate treatment regimens.
Genetic Testing Methods
Genetic testing plays a pivotal role in diagnosing genetic diseases. Several methods are prominent in this field, including targeted testing, carrier screening, and prenatal testing.
Targeted Testing
Targeted testing involves focusing on specific genes associated with particular disorders. This method primarily aims to confirm or rule out a suspected diagnosis based on clinical evaluations. The key characteristic of targeted testing is its high specificity, which significantly increases the likelihood of detecting mutations responsible for a disease. This focus makes it a popular choice for conditions where gene variants have been well-characterized, such as cystic fibrosis or Duchenne muscular dystrophy.
One unique feature of targeted testing is its ability to provide rapid results. This speed is advantageous in clinical scenarios where timely decisions are necessary. However, its limitation lies in that it only tests for known mutations, potentially overlooking other genetic factors that may contribute to the patient's condition.
Carrier Screening
Carrier screening aims to determine if an individual carries a gene for a specific inherited condition. This screening is particularly relevant for recessive disorders, where both parents must be carriers for the disease to manifest in offspring. The key characteristic of carrier screening is its preventive nature, allowing at-risk couples to make informed reproductive choices.
A unique feature of carrier screening is its capacity to test for multiple disorders at once, utilizing advanced technologies such as next-generation sequencing. This comprehensive approach makes it a beneficial method for individuals with a family history of genetic disorders. However, it comes with psychological implications, as discovering one's carrier status may affect emotional well-being and family dynamics.
Prenatal Testing
Prenatal testing facilitates the assessment of a fetus for specific genetic conditions before birth. The importance of this testing lies in its ability to detect disorders such as Down syndrome or spina bifida early in pregnancy. The key characteristic of prenatal testing is that it can inform expectant parents about potential health issues, enabling them to prepare for the possible challenges ahead.
One unique feature of prenatal testing is the option for non-invasive procedures, such as cell-free fetal DNA testing, which presents lower risks to both mother and child. However, the challenge with prenatal testing is deciding on the implications of the results. Parents must carefully consider their options, which can lead to complex emotional and ethical discussions about the future of their child.
Role of Bioinformatics
Bioinformatics plays a significant role in the diagnosis of genetic diseases. It provides the computational tools necessary for analyzing vast amounts of genomic data and transforming them into clinically relevant information. By employing bioinformatics, researchers can interpret genetic variations and link them to specific diseases. The integration of bioinformatics enhances accuracy in diagnosing genetic conditions, ultimately contributing to better patient outcomes.
Treatment and Management of Genetic Diseases
The treatment and management of genetic diseases represent a crucial area of focus within genomic medicine. These diseases often arise from complex interactions at the genomic level, which can affect individual health in profound ways. Understanding how to treat and manage these conditions can not only improve the quality of life for affected individuals but also reduce the burden on healthcare systems. The approaches employed can be diverse, depending on the underlying genetic disorder and its characteristics.
Conventional Treatments
Conventional treatments are methods rooted in established medical practices. These methods can include medication, surgery, or other interventions that aim to alleviate symptoms rather than cure the genetic disorder itself. For instance, conditions like cystic fibrosis can require therapies that help manage respiratory function and prevent infections.
Moreover, for metabolic disorders such as phenylketonuria, dietary restrictions and supplementation can significantly improve patient outcomes. Each treatment plan needs to be tailored to the individual's condition, considering factors such as age, severity of the disease, and overall health.
The effectiveness of these treatments can be influenced by various factors, including adherence to treatment protocols and early detection of the disorder. It's crucial to understand that while conventional methods may control symptoms, they often do not address the genetic root cause.
Gene Therapy Innovations
Gene therapy is an innovative approach focusing on correcting the underlying genetic defects responsible for a disease. This method holds promise for a range of genetic disorders, particularly those that are monogenic, where a single gene is involved. The understanding of CRISPR technology has significantly accelerated progress in this field, allowing for precise editing of genes.
One notable example of gene therapy success is the treatment for spinal muscular atrophy, which uses a modified virus to deliver a healthy copy of the SMN1 gene to patients. This has transformed the prognosis for many affected individuals, underscoring the potential of gene therapy.
Gene therapy is not without its challenges, though. One concern is the long-term effects and the possibility of unforeseen side effects from altering the genome. Regulatory frameworks and ethical considerations must be addressed to ensure the safety and efficacy of such innovations.
"Gene therapy is not just a treatment; it is a shift towards a future where we can potentially cure previously untreatable genetic conditions."
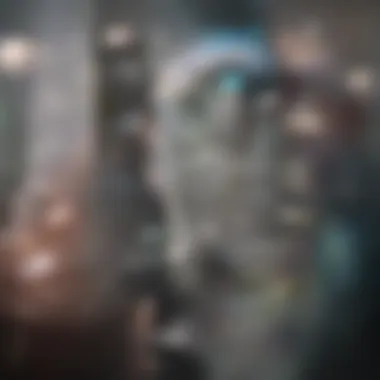
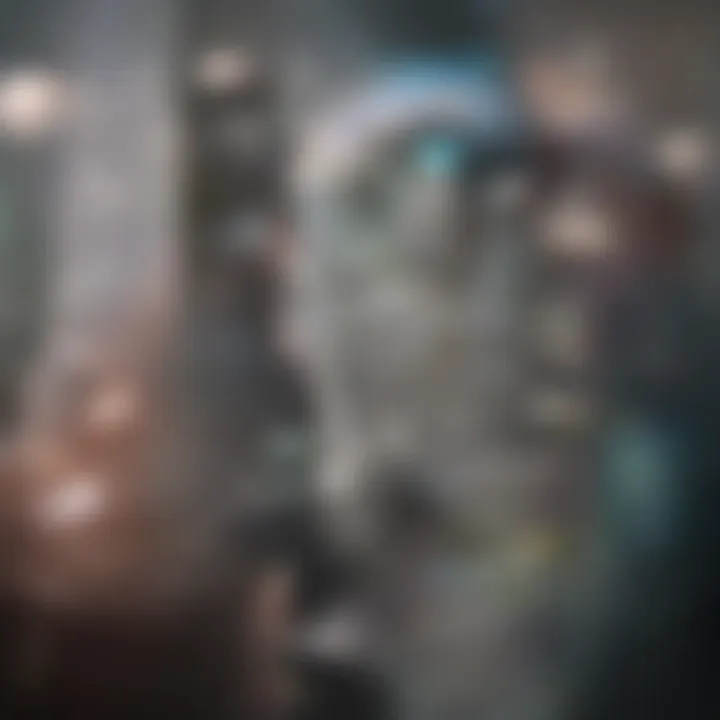
Ethical Considerations in Genomic Research
In the exploration of human genome diseases, ethical considerations play a vital role. As research advances in genomics, the implications for individuals and society become increasingly complex. Researchers must navigate a landscape that is charged with ethical dilemmas. These concerns arise mainly from the potential misuse of genomic data, the implications of genetic findings, and the overall impact on personal autonomy and societal structures.
One of the foremost ethical issues in genomic research involves the concept of informed consent. This process ensures that participants in genomic studies are fully aware of the implications of their involvement. It is essential for maintaining trust and integrity in research. Participants must understand how their genetic information will be used, the potential benefits, and risks involved. Strong informed consent processes contribute to ethical research practices, safeguarding participants' rights and welfare.
Another critical ethical concern is the privacy and confidentiality of genetic data. Genetic information is inherently personal and sensitive. Thus, it raises significant concerns about how such data is stored, shared, and protected. Researchers and institutions must implement robust measures to ensure that personal data remains secure and confidential. Failure to do so can lead to violations of individual privacy, stigmatization, and discrimination based on genetic predispositions.
Given the rapid advancements in genetic technology, it is imperative that researchers consider the broader implications of their work. The integration of ethical considerations in genomic research not only enhances scientific integrity, but also builds public confidence in genetic advancements.
Informed Consent
Informed consent serves as a cornerstone of ethical research in genomics. It underscores the necessity for researchers to clearly communicate with participants before they engage in any study. Key elements of informed consent include:
- Disclosure of information regarding the nature of the study, including potential risks and benefits.
- Comprehension to ensure that participants fully understand the information provided to them.
- Voluntariness, meaning that participants should have the freedom to choose without any coercion.
In genomics, the complexity of genetic data often complicates this process. Researchers must be adept at explaining intricate concepts and addressing any concerns, ensuring participants make well-informed decisions about their involvement.
"Informed consent is not merely a formality, but an agreement to uphold the participantβs autonomy and rights."
Privacy and Confidentiality
Privacy and confidentiality are paramount in genomic research. The sensitive nature of genetic data cannot be overstated. Any breach of confidentiality can have severe consequences for individuals, such as discrimination in employment or insurance. To help mitigate such risks, researchers must adhere to strict protocols for data handling.
Several strategies to enhance privacy and confidentiality include:
- Implementing anonymization protocols to separate personal identifiers from genetic data.
- Strengthening data security measures to prevent unauthorized access.
- Ensuring that participants are aware of how their data will be used and shared throughout the research process.
The ethical obligation to protect personal information is coupled with the responsibility to promote transparency about research practices. As genomic research progresses, addressing these ethical concerns is vital for fostering a trustworthy environment that encourages participation in groundbreaking studies.
Future Directions in Genomic Medicine
The field of genomic medicine holds significant promise for transforming healthcare. As technology advances, our understanding of the genome accelerates. This section discusses the innovative aspects that could shape the future of medical practice. We will explore personalized medicine and preventive strategies as two key pillars supporting the evolution of treatment methods.
Personalized Medicine
Personalized medicine aims to tailor healthcare to individual characteristics. It utilizes genomic information to predict disease risk, understand disease mechanisms, and select appropriate treatment options.
- Tailored Treatments: By analyzing a person's genetic makeup, healthcare providers can devise specific therapies that are more likely to produce favorable outcomes. For example, cancer treatments can now focus on individual tumor profiles, improving efficacy while minimizing side effects.
- Drug Development: Pharmaceutical companies increasingly consider genetic markers when developing new drugs. This approach looks for variations in genes that may affect drug metabolism or efficacy, allowing for a more targeted approach in treatment.
- Patient Engagement: Involving patients in the decision-making process regarding their care can lead to better adherence to treatment plans. When patients understand how their genetics impact their health, they are more likely to comply with recommendations.
The significance of personalized medicine cannot be overstated. It represents a departure from one-size-fits-all practices and introduces a more specific approach to managing health conditions. Integrating genomic data into everyday clinical practice enhances both the quality of care and patient outcomes.
Potential for Preventive Strategies
Preventive strategies in genomic medicine focus on reducing the incidence of diseases before they manifest. These strategies can lead to significant public health improvements.
- Risk Assessment: Genomic screening allows for efficient identification of individuals at high risk for certain diseases. For example, BRCA1 and BRCA2 genetic tests can inform women about their risk of breast and ovarian cancer, prompting proactive measures.
- Lifestyle Modifications: Genetic insights can guide lifestyle changes aimed at disease prevention. Individuals may tailor their diet, exercise, and other health behaviors based on their unique genetic predispositions.
- Public Health Programs: Knowledge gained from genomic research can inform community-level interventions. This includes tailored educational campaigns and screening programs that address genetic risks within specific populations.
The future of genomic medicine appears poised for tremendous advancements. As our knowledge expands, the capability to prevent diseases and personalize care is set to alter the landscape of healthcare profoundly. The emphasis on prevention and tailor-made strategies not only enhances individual patient care but also aims for broader impacts across entire populations.
"Genomic medicine can redefine the healthcare approach by shifting focus from reactive to proactive health management."
Inclusion of genomics in medical practice prepares healthcare systems for a future where precision and prevention take precedence. Ultimately, ongoing research and innovation will propel us towards a more health-conscious society.
Epilogue
In the realm of human genomics, the conclusion serves as a pivotal point that encapsulates the profound insights and implications gleaned from the exploration of genetic diseases. Understanding the complexities of how human genetic variations relate to health and illness is of paramount importance. It allows researchers, medical professionals, and bioethicists to navigate the intricate landscape of genetic disorders, therapies, and the ethical dilemmas presented by genomic technologies.
Summary of Key Insights
The analysis presented throughout this article underscores several key points regarding human genome diseases:
- Diversity of Genetic Disorders: The spectrum of genetic disorders is vast and includes monogenic, multifactorial, and mitochondrial disorders, each presenting unique challenges in diagnosis and treatment.
- Role of Genomic Research: Continuous advancements in genomic research, particularly through next-generation sequencing and genome-wide association studies, are critical in unraveling the genetic underpinnings of diseases.
- Ethical Considerations: As the field of genomics evolves, ethical considerations, including informed consent and privacy, become increasingly vital. The balance between the potential benefits of genomic research and ethical implications must be maintained.
- Future Directions: Personalized medicine represents a significant shift towards tailored health interventions based on individual genomic profiles, promising improved outcomes for patients.
Call for Continued Research
The realm of genomic medicine is still in its infancy, necessitating ongoing research to further unlock the potential of the human genome in addressing health issues. Key areas warranting focused exploration include:
- Longitudinal Studies: Extensive studies tracking individuals over time can shed light on how environmental factors interact with genetic predispositions to contribute to diseases.
- Innovative Therapeutics: Continued investment in gene therapy and precision medicine could revolutionize approaches to managing genetic disorders, making them more effective and accessible.
- Integration of AI: Leveraging artificial intelligence in genomic research holds promise for identifying patterns and predicting disease susceptibility based on genomic data.
- Public Engagement: Engaging the public on genomics can foster understanding and support for research initiatives while addressing ethical considerations head-on.
The future of human genome diseases is ripe with opportunities for innovation and improved health outcomes. As the scientific community fosters collaboration and knowledge-sharing, the journey toward understanding and addressing genetic diseases will undoubtedly progress. This pursuit not only holds benefits for individuals but also enriches our collective understanding of human genetics.