Exploring Inducible shRNA Lentivirus: Mechanisms, Applications, and Future Directions
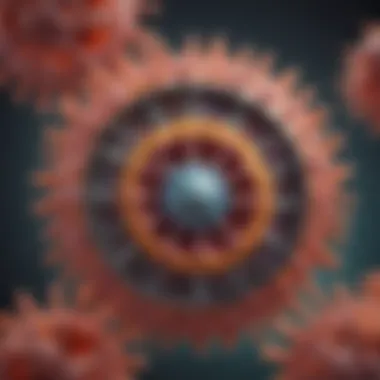
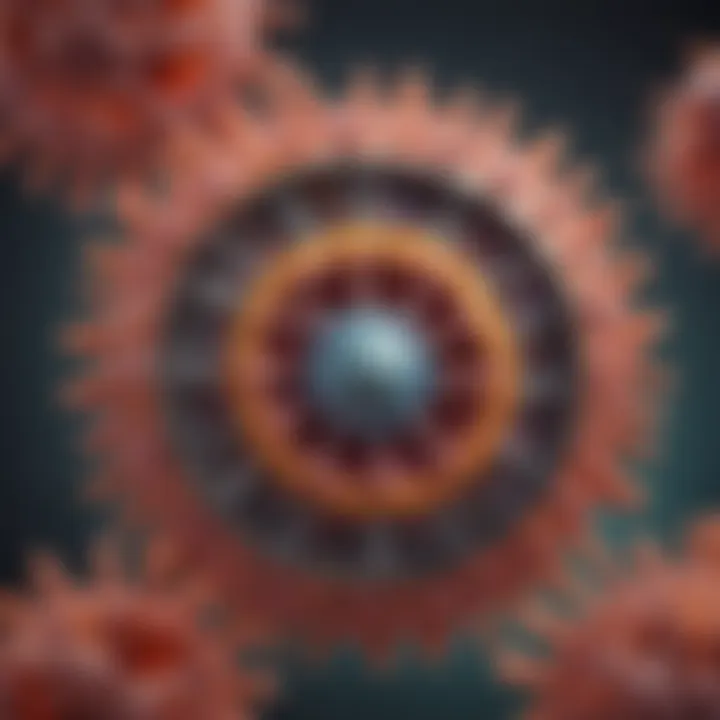
Intro
Inducible shRNA lentiviruses represent a significant advancement in the field of gene silencing and therapeutic interventions. Their design allows for controlled expression of short hairpin RNA (shRNA), which is crucial for targeting specific genes at chosen times. Understanding how these systems operate is vital for scientists seeking to manipulate gene expression in various biological contexts.
The relevance of inducible shRNA lentiviruses extends across multiple disciplines, including cancer research, genetics, and neurobiology. This article serves as a comprehensive guide to their mechanisms, applications, and future prospects.
Recent Advances
Latest Discoveries
Recent research has led to crucial insights regarding the efficacy and specificity of inducible shRNA lentivirus systems. Studies have demonstrated their ability to facilitate gene knockdown while minimizing off-target effects. These advances are critical, particularly in the context of disease model development, where precision is paramount.
Technological Innovations
Innovations in viral vector engineering have improved the delivery and expression control of shRNA. For example, the incorporation of doxycycline-responsive elements enhances temporal control over shRNA expression. Such innovations allow researchers to induce gene silencing at specific developmental stages or in response to experimental conditions. This level of control is essential for dissecting complex biological pathways.
Methodology
Research Design
The research design for studies utilizing inducible shRNA lentiviruses often includes both in vitro and in vivo experiments. Cell lines are typically manipulated to express the lentivirus, followed by gene expression analysis upon shRNA induction. For in vivo studies, animal models can provide insights into how inducible shRNA influences biological processes within a living organism.
Data Collection Techniques
Data collection in this research typically involves quantitative PCR to measure gene expression levels. Additionally, Western blotting can confirm the reduction of target protein levels. These techniques ensure that the impact of shRNA expression on both transcript and protein levels is thoroughly assessed, providing a complete picture of the system’s functionality.
The systematic approach to collecting and analyzing data allows for rigorous evaluation of the efficiency and reliability of inducible shRNA lentiviruses, informing future applications and developments in the field.
Understanding the Basics of RNA Interference
The topic of RNA interference (RNAi) is fundamental to the understanding of gene regulation and practical applications in molecular biology. In recent years, RNAi has emerged as a powerful tool in gene silencing. The exploration of RNA interference mechanisms provides insight into how specific genes can be targeted and controlled. The ability to silence genes effectively offers significant advantages for both research and therapeutic uses.
The Mechanism of RNA Interference
RNA interference primarily involves the use of small RNA molecules to silence specific genes. This process starts with double-stranded RNA or long hairpin RNA being recognized by an enzyme called Dicer, which then cleaves the longer strands into smaller fragments known as small interfering RNA (siRNA). These siRNAs are subsequently incorporated into a protein complex known as the RNA-induced silencing complex (RISC). Once within RISC, the siRNA guides the complex to complementary messenger RNA (mRNA) targets, leading to degradation of these mRNA strands. By doing so, gene expression is inhibited, ultimately affecting the protein levels within a cell. Understanding this mechanism highlights the profound impact RNA interference can have on regulating gene expression.
Types of RNA Molecules in Gene Silencing
The landscape of gene silencing is populated by various types of RNA molecules, each contributing uniquely to the process. This section outlines the three main types: siRNA, shRNA, and miRNA.
siRNA
The small interfering RNA (siRNA) is crucial for initiating the process of RNA interference. Its characteristic feature lies in its short length, typically 20-25 nucleotides. This makes siRNAs easily recognized by the Dicer enzyme, aiding in their ability to trigger gene silencing. siRNAs are beneficial because they provide specific targeting, allowing for precise manipulation of gene expression. However, the short lifespan of siRNAs within cells can be a limitation, as they may not persist long enough for prolonged research or therapeutic effects.
shRNA
Short hairpin RNA (shRNA) serves as another pivotal component in gene silencing mechanisms. shRNAs are designed with a loop structure that allows them to mimic the natural formation of siRNA. Their key advantage is the ability to be expressed from a DNA vector, enabling persistent expression of the silencing effect. This makes shRNA a popular choice in many applications within genomic research settings. Despite their advantages, shRNAs can face challenges such as potential off-target effects, which can complicate the specific targeting of genes.
miRNA
MicroRNA (miRNA) molecules play a slightly different role in gene silencing. Unlike siRNA and shRNA, miRNAs are involved in the regulation of gene expression at a broader level, targeting multiple mRNAs simultaneously. They are initially transcribed as longer precursors, eventually processed into functional miRNAs. Due to their capacity for finely tuning gene expression and involvement in diverse regulatory networks, miRNAs save a significant place in various biological processes and disease mechanisms. The potential downside includes their less precise nature when targeting genes, which may lead to unintended consequences.
In summary, understanding these molecules' characteristics lays the groundwork for comprehending the complexities of inducible shRNA lentivirus mechanisms.
Foreword to Lentiviral Vectors
Lentiviral vectors have emerged as essential tools in genetic engineering and modern biomedicine. Their capacity to deliver genetic material into a wide variety of cell types makes them invaluable for research and therapeutic applications. Understanding these vectors is critical in the context of inducible shRNA systems, as they enable precise control over gene expression to study gene function and potential treatments for various diseases.
One of the fundamental aspects of using lentiviral vectors is their ability to integrate into the host genome efficiently. This property allows for long-term expression of the delivered gene or shRNA, making it particularly useful for experiments that require sustained gene knockdown. Moreover, compared to other viral systems, lentiviruses exhibit a broader host range, including both dividing and non-dividing cells.
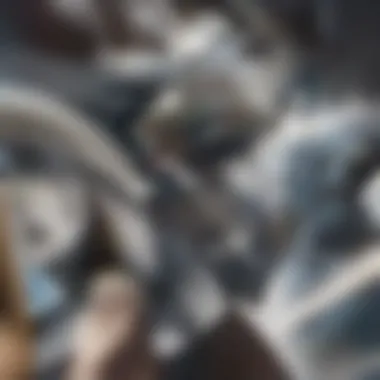
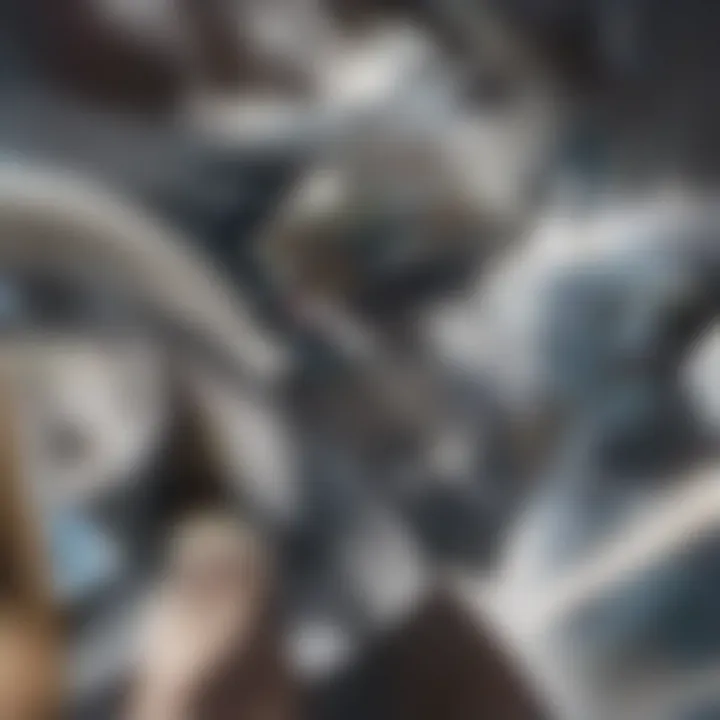
Key elements that make lentiviral vectors significant include:
- Their stable integration into the genome.
- Ability to infect non-dividing cells, which many other viruses cannot.
- Capacity for high levels of expression from transgenes.
The careful design of lentiviral vectors has various considerations, including safety, efficiency of transduction, and the potential for off-target effects. Understanding these factors is vital when developing inducible systems aimed at controlling gene expression.
Characteristics of Lentiviral Vectors
Lentiviral vectors originate from the lentivirus family, specifically HIV, and have been modified to enhance safety and efficacy for research applications. Some of the defining characteristics include:
- Self-Assembly: Lentiviral vectors can be generated through a simple process whereby plasmids are co-transfected into producer cell lines, leading to the generation of infectious viral particles.
- Packaged RNA Genome: The lentiviral vector includes a modified version of the viral genome, which facilitates the expression of desired genes.
- Envelope Protein Selection: By modifying envelope proteins, researchers can alter the cell specificity of the lentiviral vector, allowing for tailored approaches in different cell types.
These characteristics allow for a high level of versatility in experiments ranging from basic research to therapeutic applications.
Advantages of Using Lentiviral Vectors
Lentiviral vectors confer several advantages that make them preferable for many applications in molecular biology and medicine. These benefits include:
- Long-term Transgene Expression: Since lentiviruses integrate into the host genome, they can provide stable and long-lasting expression of the transgene or shRNA.
- Multi-Cell Type Targeting: They are capable of infecting a broad range of cell types, thus expanding the potential experimental subjects. This property is crucial for studies that require diverse biological contexts.
- High Efficiency of Gene Delivery: Lentiviral vectors achieve high transduction efficiency, resulting in consistent outcomes in gene expression studies.
- Ability to Accommodate Large Inserts: Lentiviral vectors can carry larger genetic payloads compared to some other gene delivery systems, allowing for a more complex genetic manipulation.
"Lentiviral vectors stand out due to their potent ability to mediate stable transgene expression across a variety of cell types, a feature that distinguishes them from other vector systems."
In summary, the integration of inducible shRNA systems with lentiviral vectors provides a robust framework for gene silencing studies and opens the door for innovative therapeutic approaches in various diseases.
Inducible shRNA: Concept and Significance
Inducible shRNA (short hairpin RNA) systems represent a significant advancement in the realm of gene silencing technologies. Their primary purpose is to facilitate a controlled suppression of gene expression, achieved by the use of lentiviral vectors that carry inducible shRNA sequences. Such systems allow researchers to activate or deactivate RNA interference in response to specific stimuli. This flexibility is particularly beneficial in complex biological studies, as it enables the manipulation of gene expression with temporal precision.
Overview of Inducible Systems
Inducible shRNA systems are often engineered using various regulatory elements that can respond to specific compounds or environmental conditions. The most common methods of control include drug-inducible systems, such as the Tet-On and Tet-Off regulatory systems. In these systems, the presence of a tetracycline derivative allows for the quick and reversible activation or deactivation of shRNA expression.
Another common mechanism involves the use of synthetic promoters that respond to specific stimuli like light or heat. This precision makes inducible shRNA systems powerful tools in functional genomics and therapeutic applications. Dealing with complex cellular environments, it becomes crucial to correctly tune gene silencing effects without enduring off-target consequences.
Importance of Inducibility in Gene Silencing
The significance of inducibility in gene silencing cannot be overstated. Traditional shRNA systems often lead to permanent gene silencing, which may disrupt normal cellular processes and obscure the functional relevance of the targeted gene. Inducible shRNA systems mitigate this risk by allowing the controlled expression of shRNA, thus minimizing unwanted side effects.
Moreover, the ability to temporally modulate gene silencing opens up new avenues in disease modeling and therapeutic development. Researchers can study the effects of gene silencing in real-time, providing insights into developmental processes, cancer progression, and response to drug treatments. This capability equips scientists with a broader understanding of gene function dynamics, ultimately fostering more effective therapeutic strategies.
"Inducible systems allow researchers to dissect complex biological processes by enabling selective and reversible gene silencing, which improves the robustness of experimental findings."
In summary, the concept of inducible shRNA is not merely of academic interest. It represents a fundamental shift towards more sophisticated and controlled gene regulation strategies. As researchers explore these systems, the insights gained will enhance our ability to understand and manipulate gene expression, paving the way for significant advancements in medicine and biotechnology.
Designing Inducible shRNA Lentivirus
Designing inducible shRNA lentivirus is a critical aspect of utilizing this powerful tool in both research and therapeutic settings. The primary goal of this design is to ensure effective gene silencing in a controlled manner. This controlled expression allows researchers to observe the effects of gene knockdown at specific times, providing deeper insights into biological processes and disease states.
By optimizing the design of the inducible shRNA lentivirus, several benefits can be achieved. Firstly, there is enhanced specificity in targeting the desired genes. A well-constructed shRNA sequence can minimize off-target effects, which are often a significant concern. Secondly, the use of inducible systems enables temporal control over gene silencing. This can be especially valuable in temporal studies of gene function or in pathological contexts where rapid changes in gene expression need to be traced.
While designing these systems, understanding the various elements involved is essential. Factors such as the choice of shRNA sequence, integration sites, as well as the type of promoter utilized must be carefully considered. All these elements play a role in how effectively the shRNA can silence its target gene and how reliably it can be induced.
Constructing the shRNA Sequence
Constructing the shRNA sequence is perhaps one of the most pivotal steps in the design of inducible systems. The sequence should ideally form a perfect stem-loop structure, as this configuration is crucial for efficient processing by the cellular machinery. The guide strand of the shRNA must be complementary to the target mRNA to ensure specific binding and subsequent degradation.
A good approach for shRNA design involves:
- Identifying the Target Gene: The selection of the specific target mRNA is the first step. This requires thorough knowledge of the gene’s sequence and function.
- Designing Unique shRNA: Tools such as RNAfold or online design software can assist in designing unique shRNA sequences that minimize off-target interactions.
- Testing and Validation: Once constructed, the shRNA sequences should be empirically tested in vitro to confirm their knockdown efficiency before being incorporated into a lentiviral vector.
Following a rigorous design and testing phase enables researchers to generate robust and effective shRNA sequences that are necessary for compelling experimental outcomes.
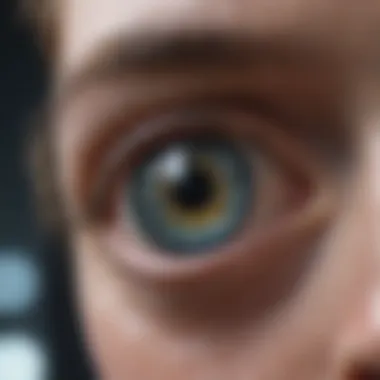
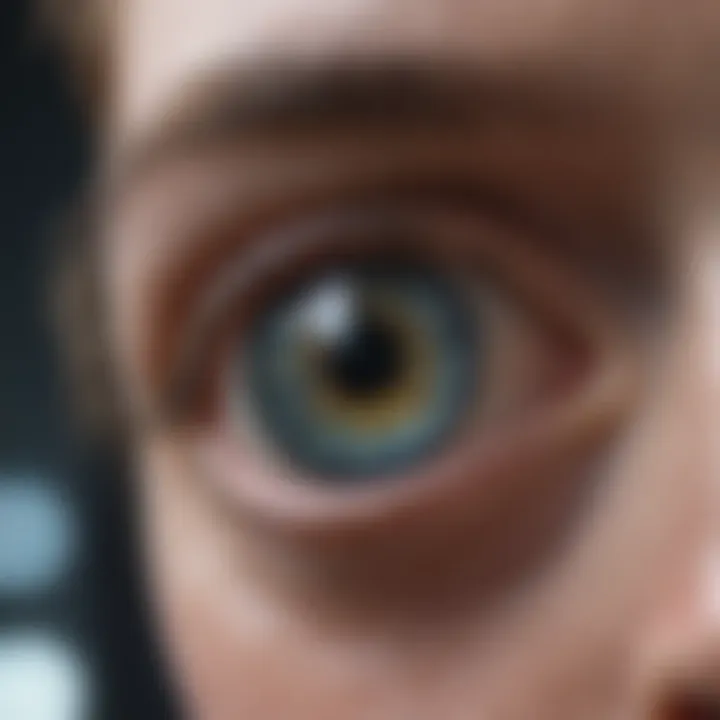
Selecting Appropriate Promoters
Selecting appropriate promoters for inducible shRNA lentivirus is another significant consideration in the design phase. The promoter controls when and how much shRNA is produced within the host cell. Different promoters allow for variations in expression levels and inducibility, which is essential for precise experimental outcomes.
Commonly used inducible promoters include the tetracycline-responsive element and the IPTG-inducible promoter. These promoters have distinct characteristics that make them suitable for various applications:
- Tetracycline-Responsive Promoter: This promoter allows for immediate control over shRNA expression upon the addition of tetracycline or doxycycline.
- IPTG-Inducible Promoter: This promoter is used in bacterial systems and can be exploited for easier manipulation in certain contexts, especially when recombinant proteins are involved in the research.
Choosing the right promoter is critical for ensuring the timing and level of shRNA expression is aligned with experimental requirements. In many cases, researchers may need to assess several promoters to determine which produces the optimal balance between expression control and silencing efficiency.
Methods of Generating Inducible shRNA Lentivirus
Generating inducible shRNA lentivirus requires careful consideration of various methodologies that influence the overall efficacy and usability of the resultant viral particles. This process is essential in ensuring precise control over gene silencing, which is crucial for elucidating biological processes and developing therapeutic strategies. The design and execution of these methods directly impact the success of experiments and applications in research.
Transfection Techniques
Transfection is the process of introducing nucleic acids into cells. This is a vital first step in generating inducible shRNA lentivirus. Various techniques exist for achieving successful transfection, each with distinct advantages and limitations. The most commonly employed methods include:
- Lipofection: This method utilizes lipid-based reagents to form complexes with nucleic acids. These complexes facilitate the fusion with the cell membrane, allowing for the entry of shRNA into the target cells. It is often chosen for its simplicity and effectiveness, particularly with adherent cell lines.
- Electroporation: This technique involves applying an electrical field to cells to create temporary pores in their membranes. This allows nucleic acids to enter the cells more efficiently. Though it can be effective for various cell types, careful optimization is required to prevent cell death due to high voltages.
- Viral Transduction: Utilizing lentiviral vectors for transduction is a robust approach for generating stable cell lines that express inducible shRNA. Here, the lentivirus carrying the shRNA sequence is introduced to target cells, allowing for effective integration into the host genome.
"Choosing the right transfection technique is pivotal for producing high-titer lentiviruses suitable for downstream applications."
In practice, the choice of transfection technique often depends on cell type, desired efficiency, and whether transient or stable expression is required. Each method necessitates optimization to achieve maximum transfection efficiency while minimizing cytotoxicity.
Virus Production and Purification
After successful transfection, the next phase is the production and purification of the lentivirus. This involves several systematic steps:
- Cell Culture: Following transfection, it is crucial to maintain optimal growth conditions for the producer cells. Typically, 293T cells are used for their high transfection efficiency and ability to produce large amounts of virus.
- Harvesting: The culture medium containing the viral particles should be collected at specific time points post-transfection. It is common to harvest the supernatant at 48 and 72 hours to maximize yield.
- Concentration: Purification methods like ultracentrifugation or filtration through 0.45 µm filters can provide a concentrated viral preparation. This step is important for removing cellular debris and concentrating the virus for subsequent experiments.
- Characterization: Following purification, the viral preparation must be characterized. This is often done by measuring the titer of the viral stock using techniques such as quantitative PCR or a functional assay to determine its ability to transduce target cells.
Purified virions are essential for experiments in gene silencing and therapeutic applications. The efficiency of virus production significantly impacts the success of subsequent applications in functional genomics or therapeutic approaches.
Applications of Inducible shRNA Lentivirus in Research
The advent of inducible shRNA lentiviruses has brought significant advancements in research methodologies. These systems allow for precise control of gene expression, making them crucial tools for investigating the workings of genes and their associated pathways. Their applications span various fields, including functional genomics, disease modeling, and target validation in therapeutic research. Thus, understanding their role in these areas is of paramount importance.
Functional Genomics
Inducible shRNA lentiviruses serve as vital instruments in functional genomics. They enable researchers to identify gene function through stable knockdown of specific genes. This process is essential for delineating the roles of genes in cellular processes and diseases.
One major benefit is how these lentiviral vectors facilitate the examination of gene interactions without the permanent gene silencing that traditional methods impose. For instance, researchers can induce gene knockdown in response to specific stimuli or at defined developmental stages. This flexibility allows for a more nuanced understanding of gene function in dynamic biological contexts.
Moreover, by employing specific promoters that respond to various inducers, such as doxycycline, scientists can achieve temporal control. This method helps minimize off-target effects and unintended cellular responses often associated with constant gene silencing. The ability to toggle gene expression provides a clearer picture of gene function, enhancing the overall understanding of biological pathways.
Disease Models
Inducible shRNA lentivirus systems prove valuable in the construction of disease models, particularly for studying complex conditions like cancer, neurodegenerative disorders, and infections. By allowing controlled knockdown of genes implicated in these diseases, researchers can closely monitor the progression and effects of the disease.
For example, in cancer research, specific oncogenes or tumor suppressor genes can be silenced in cell lines or animal models, enabling the study of tumor biology and the effects on cellular signaling pathways. This methodological approach helps in identifying novel therapeutic targets and could lead to breakthroughs in treatment strategies.
Additionally, modeling diseases with inducible systems brings about an ethical advantage, reducing the need for animal experiments by allowing in vitro analysis alongside in vivo approaches. Researchers can thus generate more ethically sound and reproducible data.
Target Validation
Target validation is critical in the drug discovery process, and inducible shRNA lentivirus plays a prominent role in this aspect as well. After identifying potential drug targets, it's essential to confirm that silencing these targets affects the desired biological pathway. This is where inducible shRNA lentiviral systems shine.
By using these systems, scientists can induce knockdown of target genes and subsequently assess changes in cellular responses or pathways. Such an approach offers clear insights into the viability of target genes as candidates for therapeutic intervention. It allows the evaluation of whether inhibiting a specific gene leads to the desired phenotypic changes, either in cellular assays or in live models.
In summary, the applications of inducible shRNA lentivirus in research are vast and critical to advancing our understanding of gene function, disease mechanisms, and potential therapeutic targets. Their precision and flexibility make them indispensable tools in the modern molecular biology toolkit.
Therapeutic Potential of Inducible shRNA Lentivirus
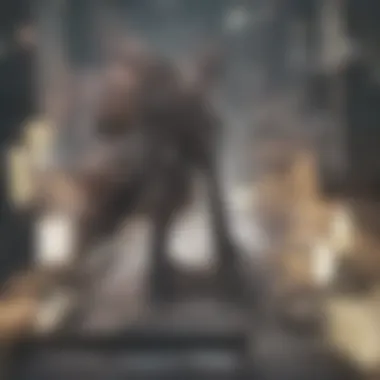
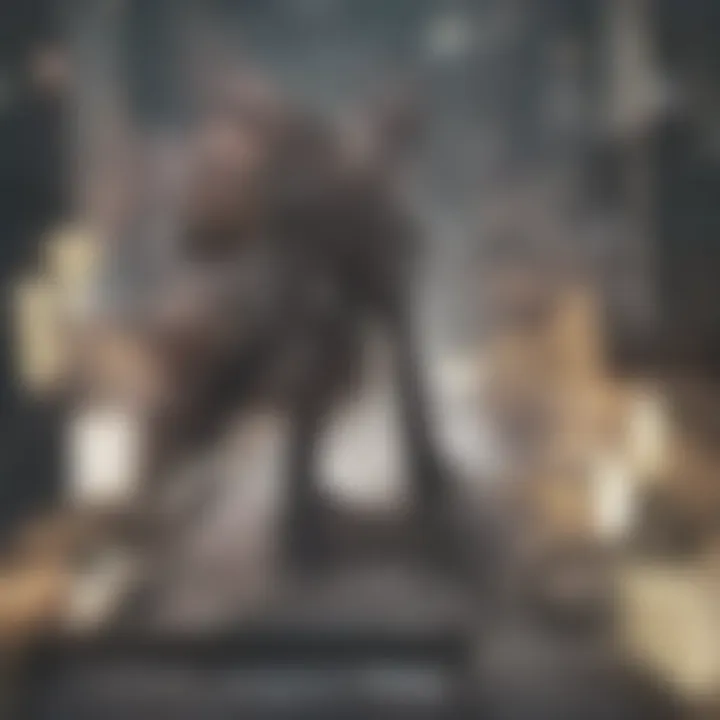
Inducible shRNA lentiviruses present a transformative approach in the realm of gene therapy. They offer a highly versatile tool for selectively silencing gene expression, giving researchers the ability to modulate biological processes in real-time. This capability is crucial for therapeutic applications, particularly in complex diseases where gene regulation plays a significant role.
Inducible systems allow for the temporal control of gene silencing. This means that researchers can turn the gene silencing effects on or off depending on experimental needs, which is particularly advantageous in therapeutic settings. For instance, in treating diseases like cancer, where overexpression of certain genes drives tumor growth, it is necessary to carefully regulate the silencing of those genes without affecting normal cellular functions.
Gene Therapy Applications
The utility of inducible shRNA lentiviruses in gene therapy is profound. By facilitating the silencing of malfunctioning genes, they hold promise for various genetic disorders where a targeted gene contributes to pathogenesis. Notably:
- Targeted Gene Silencing: Inducible shRNA provides specificity in targeting genes associated with specific diseases. This precision reduces the likelihood of off-target effects common in traditional gene editing techniques.
- Temporal Control: Researchers can regulate when and how much of a gene is silenced, which is critical in delicate situations like managing doses for overactive genes.
- Clinical Relevance: Preclinical studies have shown that inducible shRNA can effectively reduce the expression of genes implicated in conditions such as cystic fibrosis and muscular dystrophy, leading to a more favorable outcome compared to permanent knockdown methods.
Cancer Research and Treatment
Inducible shRNA lentiviruses also play a vital role in cancer research and treatment. The ability to silence oncogenes temporarily can lead to significant breakthroughs in targeted cancer therapies. Some key points include:
- Oncogene Silencing: Many cancers are driven by the aberrant expression of specific oncogenes. Using inducible shRNA, researchers can explore the effects of silencing these oncogenes on tumor progression.
- Resistance Mechanisms: By silencing resistance-related genes, inducible shRNA facilitates a deeper understanding of how certain tumors evade treatments. This knowledge is crucial for developing effective combination therapies.
- Therapeutic Angle: Clinical trials have begun to assess the efficacy and safety of using inducible shRNA in actual patients, particularly in hematological malignancies. These studies aim to determine how well these systems can improve treatment responses and overall survival rates.
The adaptability and precision of inducible shRNA lentiviral systems mark a significant advancement in targeted therapies, paving the way for innovative approaches in both research and clinical applications.
The exploration of inducible shRNA lentiviruses highlights an evolving frontier in gene therapy. Their potential not only addresses immediate therapeutic needs but also sets the stage for future innovations in genetic research. As the technology matures, the understanding of gene functions, disease mechanisms, and therapeutic outcomes will likely enhance, providing more holistic approaches to complex health issues.
Challenges in Implementing Inducible shRNA Lentivirus
The use of inducible shRNA lentivirus offers notable advantages for gene silencing, yet there are challenges that must be addressed for effective implementation. Understanding these challenges is essential for researchers and practitioners who aim to utilize this technology in various applications. Key aspects include ensuring precision in targeting, maintaining vector stability, and navigating potential regulatory constraints.
Off-target Effects
One of the significant concerns associated with shRNA technology is off-target effects. These occur when the shRNA inadvertently silences unintended genes, leading to potentially confounding results. The specificity of shRNA is less than that of other RNA interference methods such as siRNA. This lower specificity can result in unintended phenotypic outcomes, complicating the interpretation of experiments. Thus, it is critical to design shRNA with a high degree of target specificity.
To mitigate off-target effects, several strategies can be employed:
- Careful Sequence Design: Utilizing computational tools to predict and avoid potential off-target sites before shRNA expression.
- Validation with Multiple Constructs: Testing different shRNA constructs targeting the same mRNA to determine which yields the expected phenotype with minimal off-target activity.
An understanding of the inherent properties of the target mRNA, such as its abundance and structure, can also assist in minimizing these effects. Although eliminating off-targeting completely might be impossible, reduction strategies significantly enhance experimental integrity.
Vector Stability and Integration
The stability of the lentiviral vector is another crucial consideration in implementing inducible shRNA. Integration of the viral genome into the host cell's DNA can vary in efficiency and stability. One primary concern is the potential for insertional mutagenesis, where random integration disrupts essential host genes or regulatory elements, leading to adverse cellular effects.
To address these risks, researchers should consider the following elements:
- Quality of Virus Production: High-quality lentivirus production enhances the efficiency of transduction, leading to more stable expression of the shRNA. Adopting standardized protocols can improve reliability in generating vectors.
- Design of the LTR Regions: The long terminal repeats (LTR) can strongly influence integration efficiency. Optimizing these regions could enhance both integration rates and stability.
Moreover, evaluating the sites of integration during the experimental design phase is essential. Techniques like next-generation sequencing can be applied to analyze the integration sites more comprehensively.
"Balancing efficacy and safety is a complex but necessary endeavor when employing inducible shRNA lentivirus technology."
In summary, while inducible shRNA lentivirus presents exciting possibilities for gene silencing in research and therapeutic contexts, the challenges related to off-target effects and vector stability warrant careful consideration. Addressing these challenges effectively ensures that the full potential of this gene-silencing approach can be realized.
Future Directions in shRNA Lentivirus Research
Research in shRNA lentiviruses is evolving rapidly. This section explores the future directions that are likely to shape the landscape of gene silencing and therapeutic applications. Focusing on advancements in delivery mechanisms and regulatory considerations is crucial. Both elements can enhance the effectiveness and safety of shRNA therapies. Modern research aims to bridge gaps in our current understanding of these topics. Understanding these future directions will help researchers develop more efficient and accessible tools for gene modulation.
Advancements in Delivery Systems
The delivery of shRNA lentivirus is a significant aspect of its efficacy. As technology progresses, new strategies are being developed to improve how these vectors are introduced into target cells. Some key advancements in delivery systems include:
- Nanoparticle Technology: Utilizing nanoparticles can enhance the stability and uptake of shRNA. By encapsulating the virus, researchers can improve cellular delivery without compromising the integrity of the RNA.
- Viral Tropism: Modifying the envelope proteins of lentiviral vectors can help increase their ability to target specific cell types. This specificity not only improves delivery efficiency but also reduces potential off-target effects.
- In Vivo Delivery Techniques: Techniques such as ultrasound or electroporation are being explored for improving the in vivo delivery of lentiviral vectors. These methods can facilitate better penetration through tissues and enhance cell transduction rates.
Ultimately, these advancements may lead to more precise interventions, minimizing collateral damage in non-target tissues, and enhancing overall safety profiles.
Regulatory Considerations
As the applications of shRNA lentivirus technologies expand, regulatory frameworks must adapt accordingly. Scientists and industry professionals should be aware of the implications of regulatory considerations affecting the development and use of these vectors. Important considerations include:
- Safety Profiles: The introduction of any new therapeutic agent requires thorough evaluation of its safety. Regulators focus on potential off-target effects and long-term consequences of gene silencing strategies.
- Bioethics: As with any biotechnological advancement, ethical considerations are paramount. Discussions about the implications of gene editing and silencing are critical for public acceptance.
- Clinical Trials: The pathway for clinical trials involving shRNA lentivirus must adhere to stringent guidelines to ensure that the results are reproducible and valid. This process includes detailed reporting of methods and outcomes.
"Regulatory scrutiny is essential to ensure patient safety while fostering innovation in gene therapy."