Infrared Spectroscopy Machines: Principles and Innovations
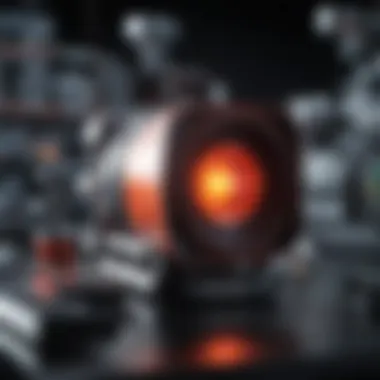
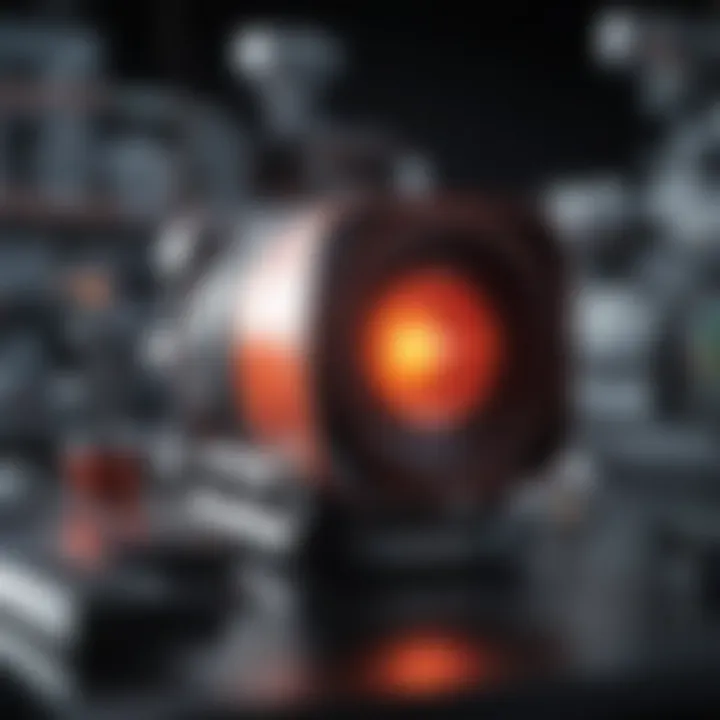
Intro
Infrared spectroscopy machines stand as pivotal instruments in the realm of scientific inquiry, bridging the gap between theoretical knowledge and practical application. By harnessing the interaction between infrared light and matter, these machines unveil a treasure trove of information regarding molecular structures, functional groups, and the dynamics of various compounds. As we venture into this exploration, it’s crucial to understand both the foundational principles that underscore their operation and the myriad of contexts in which they thrive, such as chemistry, biology, and material science.
Infrared spectroscopy involves measuring the wavelengths of light absorbed by a sample, giving insights into its molecular composition. This basic principle has remained relevant since the inception of the technology but has undergone various refinements and advances over time. In the modern context, these machines have evolved to encompass a wide range of functionalities, empowering researchers and professionals alike to produce more accurate and meaningful analyses. It is a window into the molecular world, shedding light on interactions that were once invisibly shrouded in complexity.
As we delve deeper into this article, we will uncover
- Recent advances in infrared spectroscopy machine technology,
- The methodologies that underpin research involving these machines,
- Various applications that showcase their far-reaching utilities,
- Innovations enhancing their capabilities.
By synthesizing these elements, we aim to provide a robust framework for understanding how infrared spectroscopy machines catalyze progress and discovery across multiple fields of study.
Fundamentals of Infrared Spectroscopy
Understanding the fundamentals of infrared spectroscopy is akin to having the key to a treasure chest full of scientific insights. This section serves as a cornerstone, revealing the essential concepts that underpin the operation of infrared spectroscopy machines and the insights they can provide across various scientific fields.
Principle of Infrared Spectroscopy
At its core, infrared spectroscopy is based on the interaction of infrared radiation with matter. When infrared light strikes a sample, specific wavelengths are absorbed by the sample's molecular vibrations, which causes transitions between energy levels. This process generates a unique spectrum, much like a fingerprint, for each substance examined. The principle of absorption is key here; molecules absorb light at particular frequencies corresponding to their vibrational energy levels. Thus, by studying the resulting spectrum, we can deduce both the chemical identity and concentration of different components within a sample.
It’s worth noting that this technique can apply to gases, liquids, or solids, making it vastly versatile. The interplay between the sample’s molecular structure and the infrared light provides deep insights into its chemical composition. Since each material has distinct absorbance patterns, identifying unknowns becomes a systematic endeavor, rooted in these fundamental principles.
Types of Infrared Radiation
Infrared radiation is categorized mainly into three regions: near-infrared, mid-infrared, and far-infrared.
- Near-Infrared (NIR): Ranges from 0.75 to 2.5 micrometers. This region is often useful in analyzing overtones and combination modes of molecular vibrations, pivotal in agricultural and pharmaceutical sectors.
- Mid-Infrared (MIR): Spanning from 2.5 to 25 micrometers, this range is where fundamental vibrations occur, accessible for organic and inorganic materials analysis.
- Far-Infrared (FIR): Extends from 25 to 1000 micrometers. Commonly explored in solid-state physics and material science, FIR aids in studying lattice vibrations in solids.
Different applications depend on these types, where selecting the appropriate infrared range can make all the difference in obtaining accurate data.
Interaction of Infrared Light with Matter
The interaction of infrared light with matter is a fascinating topic that delves into the fundamental nature of materials. When an infrared beam encounters a substance, several outcomes are possible: absorption, transmission, reflection, or scattering.
- Absorption: This is where the infrared light's energy is taken up by the molecules, leading to vibrational excitation. It produces the characteristic peaks in an infrared spectrum.
- Transmission: Some light passes through the sample without interacting; this can provide insights into the sample’s characteristics when comparing spectra.
- Reflection: Infrared light can bounce off a sample, providing vital clues, especially concerning surface properties.
- Scattering: This occurs when the light is deflected off the surface or particles, which could affect the absorption readings if not properly accounted for.
Understanding these interactions is essential as it lays the foundation for refining techniques and methods used in infrared spectroscopy today.
"Infrared spectroscopy serves as a bridge connecting theory with real-world applications, offering solutions across numerous scientific domains."
In summary, the fundamentals discussed here provide a solid backdrop for the subsequent exploration of infrared spectroscopy in academic and professional settings. Recognizing how infrared spectroscopy harnesses these basic principles enables deeper comprehension of its capabilities, significantly impacting research and development in various fields.
Components of an Infrared Spectroscopy Machine
Understanding the components of an infrared spectroscopy machine is crucial, as each part plays a specific role in the overall effectiveness and accuracy of the spectral analysis. These machines, often seen as the workhorse of analytical laboratories, are designed to execute complex procedures that measure the absorption or emission of infrared light by chemical substances. The efficiency and precision of an infrared spectroscopy machine heavily rely on its individual components. Let's break down these integral elements to establish a clearer picture of their respective functions and significance.
Light Source
The light source is the beating heart of an infrared spectroscopy machine. Without it, the whole operation wouldn't even be a non-starter. Typically, the source emits infrared radiation required for probing the sample. Common sources include Nernst glowers for powdered samples and tungsten-halogen lamps for liquids. The type of source chosen impacts the intensity and range of wavelengths available for analysis. A good-quality light source not only ensures higher sensitivity but also enhances the signal-to-noise ratio, making it easier to interpret data accurately.
Sample Holder
The sample holder might seem unassuming, but don't let its modest appearance fool you. Its main task is to securely hold the sample in the correct position during spectral analysis. Depending on the type of sample—solid, liquid, or gas—the holder can vary significantly in design and materials used. For instance, solid samples could be placed in KBr pellets, while liquids often need dedicated cuvettes. An effective sample holder minimizes scattering and allows for consistent results, ensuring that any external factors aren’t skewing the interpretation of spectral data.
Detectors
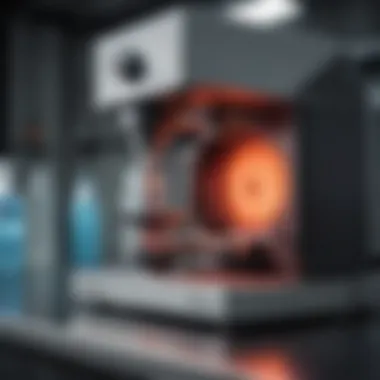
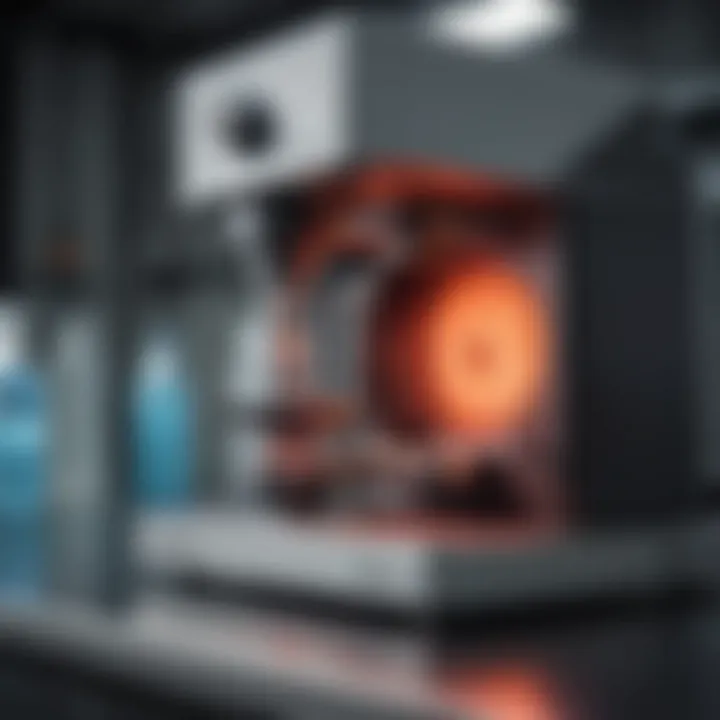
Detectors are like the audience members at a concert, eagerly waiting to absorb the sounds. In infrared spectroscopy, they collect the light that passes through or reflects off the sample. Various types of detectors are used—thermocouples, pyroelectric detectors, and photo-detectors are common choices. These detectors convert the incoming infrared light into an electrical signal, which is then analyzed. A subtle point to consider is that the choice of detector affects the machine's sensitivity and response times. Additionally, the compatibility of the detector with the light source is a core factor influencing the overall performance of the instrument.
Optical Components
The optical components of a spectroscopy machine can be likened to a finely-tuned orchestra, where each part must work in harmony to achieve the desired result. These include mirrors, beam splitters, and lenses, which all contribute to directing and focusing the infrared light onto the sample. An often-overlooked aspect, the quality of these components can have a profound effect on resolution and throughput. Even the slightest imperfection can lead to significant distortions in the spectral data, underscoring the need for precision in materials and construction.
Each part of the machine—light source, sample holder, detector, and optical components—contributes to the overall efficacy of infrared spectroscopy. To achieve optimal results, each component should be carefully selected and precisely aligned.
In summary, the components of an infrared spectroscopy machine work collectively to form a comprehensive system for accurate spectral analysis. Understanding how each part interacts and contributes to the function of the machine can empower researchers and technicians to make more informed decisions on their use, modifications, or upgrades. This knowledge can, in turn, enhance the capabilities and outcomes of their scientific investigations.
Operation of Infrared Spectroscopy Machines
The operation of infrared spectroscopy machines is the heartbeat behind their mysterious workings, translating invisible light into meaningful data. This experience encapsulates the meticulous journey from sample preparation to data interpretation, revealing the essential processes that make this analytical technique so powerful. Understanding these processes is crucial for students, researchers, educators, and professionals aiming to apply or further develop infrared spectroscopy in their work.
A well-executed operation hinges on several key elements, each playing a specific role. Notably, the quality and accuracy of the results depend on the rigorous adherence to operational protocols. Let’s delve deeper into the three critical components of this topic: sample preparation techniques, spectral data acquisition processes, and the crucial aspect of data analysis and interpretation.
Sample Preparation Techniques
Preparing a sample for infrared spectroscopy is akin to setting the stage for a grand performance. It requires meticulous attention to detail, with the aim of ensuring that the sample adequately represents the substance being studied. There are multiple methods of sample preparation, and the choice often rests on the nature of the sample itself.
- Solid Samples: For solid samples, grinding the material into a fine powder and mixing it with an inert material, like potassium bromide, is common. This ensures homogeneity and allows for clear spectral data to be collected.
- Liquid Samples: When working with liquid samples, using a suitable solvent is key. Solvents should not absorb infrared radiation in the region of interest, so careful selection is paramount. Common choices include carbon tetrachloride or chloroform, although one must be mindful of health and environmental considerations.
- Gaseous Samples: Gases require specific attention regarding cell design. Using a gas cell with appropriate path length helps enhance sensitivity and allows for overlapping spectra to be resolved.
Inadequate preparation can lead to misleading results, so understanding these techniques is vital.
Spectral Data Acquisition Process
When it comes to the spectral data acquisition process, timing and precision are everything. This process primarily involves the collection of raw data, which is converted into a spectrum representing the infrared-active vibrations present in the sample. This chain of events can make or break the reliability of analytical results.
Some pivotal considerations in the spectral data acquisition process are:
- Instrument Calibration: Calibrating the instrument ensures accuracy, allowing for reliable spectral readings.
- Scan Parameters: Defining the scan range and resolution is crucial. A narrower range with fine resolution may reveal more peaks but at the cost of longer acquisition times.
- Signal-to-Noise Ratio: Higher signal strength relative to noise can significantly improve data quality. Ensuring optimal conditions, such as avoiding ambient light and temperature fluctuations, can aid in achieving this.
This acquisition step is not just about capturing data; it’s about finding the balance between speed and precision.
Data Analysis and Interpretation
Having acquired spectral data sets the stage for the next crucial operation: data analysis and interpretation. This phase involves converting complex spectra into actionable insights, driving forward scientific knowledge or industrial applications. In this intricate web of data, the key aspects include:
- Spectral Peak Identification: Patterns within the collected spectra often indicate specific chemical bonds or functional groups present in the sample. Identifying these peaks leads to qualitative analysis.
- Quantitative Analysis: This translates to measuring the concentration of compounds within the sample, using established calibration curves from known standards.
- Software Tools: Modern infrared spectroscopy heavily relies on sophisticated software algorithms for data interpretation. These tools can help in deconvoluting overlapping spectra and thus enhance the understanding of complex mixtures.
The ability to decode spectral information also calls for a good grasp of various confounding factors that may influence result interpretation, such as sample purity and the instrument's inherent limitations.
The intricate dance of preparation, acquisition, and interpretation coalesces into a detailed picture that not only reveals the nature of samples but also propels scientific inquiry and advances in various industries.
Applications in Different Scientific Fields
The practical applications of infrared spectroscopy machines extend across various scientific fields, underpinning many research endeavors and industrial processes. This section elaborates on how infrared spectroscopy serves significant roles in chemical analysis, biological studies, and material characterization. Each application not only highlights the versatility of these machines but also underscores the advantages they provide in obtaining precise data that propel research forward. Understanding these applications gives insight into how infrared spectroscopy machines are integral to advancing knowledge in diverse domains.
Chemical Analysis
In the domain of chemical analysis, infrared spectroscopy stands out for its capacity to identify molecular structures and chemical compositions. By measuring the absorption of infrared radiation, scientists can determine the functional groups in a compound. This process is crucial for several reasons:
- Structure Identification: By analyzing the spectral data, researchers can deduce the structures of unknown compounds.
- Quality Control: In industries like pharmaceuticals, ensuring the purity of substances is paramount. Infrared spectroscopy provides an effective method for monitoring quality and consistency.
- Reaction Monitoring: During chemical reactions, infrared spectroscopy enables real-time observation of reactants and products, assisting chemists in optimizing reaction conditions.
For instance, a pharmaceutical company may utilize infrared spectroscopy to check that the active ingredients in their products meet specified criteria, thus maintaining the integrity of their formulations. This adaptability to different chemical contexts makes infrared spectroscopy an indispensable tool in labs worldwide.
Biological Studies
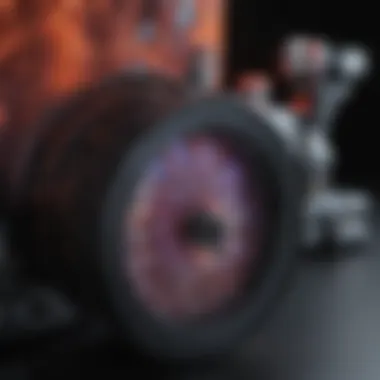
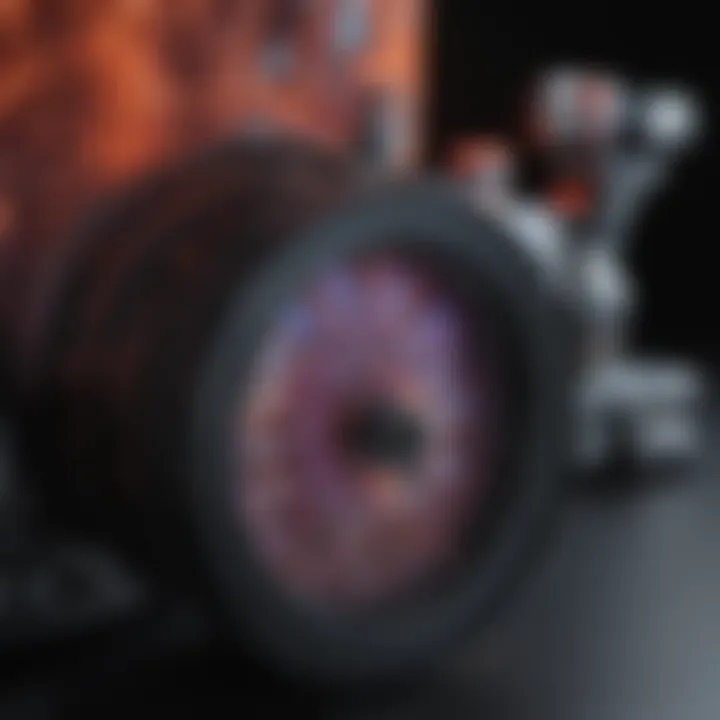
The utilization of infrared spectroscopy in biological studies reveals the intricacies of life sciences. This method has carved a niche in understanding cellular components and functions. Here’s why it’s gaining traction:
- Non-Destructive Analysis: Infrared spectroscopy allows for the analysis of biological samples without destruction, making it suitable for delicate specimens.
- Tissue Characterization: By examining the spectral signatures of various tissues, researchers can differentiate between healthy and diseased cells, aiding in medical diagnoses.
- Biodiversity Studies: In ecology, scientists employ infrared spectroscopy to analyze plant and animal samples, enhancing communication in environmental science.
An example here involves cancer research, where scientists might analyze tumor tissue to identify specific biochemical markers through infrared absorption patterns. Such application not only advances diagnosis but also aids in tailoring personalized treatment plans.
Material Characterization
The field of material characterization is another pillar that supports the applications of infrared spectroscopy. This technology is especially beneficial in assessing the properties of various materials, revealing critical insights:
- Polymer Analysis: Infrared spectroscopy is extensively used in determining the chemical structure of polymers, essential in the development of new materials.
- Surface Studies: By examining the surface chemistry of metals and coatings, researchers can determine corrosion resistance and adhesion properties.
- Nanomaterials: As the demand for nanotechnology increases, infrared spectroscopy plays a crucial role in characterizing nanostructured materials, revealing their unique vibrational properties.
Take the example of an automotive company investigating the durability of new polymer composites for vehicle manufacturing. The precise data obtained through infrared spectroscopy helps engineers refine material formulations, ultimately leading to enhanced product performance.
"Infrared spectroscopy provides a lens through which scientists can peer into the molecular world. It reveals not just the composition, but the very essence of materials, bio-systems, and chemical reactions."
Each of these applications emphasizes the vital role of infrared spectroscopy in scientific research. By advancing our understanding in chemistry, biology, and materials science, infrared technology supports progress across multiple scientific disciplines and industries.
Recent Innovations in Infrared Spectroscopy
The field of infrared spectroscopy has seen a remarkable evolution in recent years, significantly improving the capabilities and applications of this analytical technique. These innovations are not just about keeping up with modern technology; they mark a paradigm shift in how researchers and industries utilize infrared spectroscopy to derive insights from complex data. As we delve into the specific areas of advancement—detector technology, software algorithms, and integration of techniques—we will uncover their far-reaching implications for chemistry, biology, and materials science.
Advancements in Detector Technology
One of the cornerstones of modern infrared spectroscopy is its detectors. In the past, detector technology often limited the precision and speed of data collection, but recent strides have changed the game. For instance, developments like quantum cascade lasers and microbolometer-based systems provide remarkably enhanced sensitivity. These detectors can pick up even the faintest signals, which is particularly crucial in fields dealing with minute quantities such as pharmaceuticals and environmental monitoring.
The choice of detector can significantly influence the overall performance of an infrared spectrometer. Devices that can operate across a wider range of wavelengths yet maintain high resolution offer scientists richer datasets. These detectors are often described as the eyes of the instruments, capturing the intricate details of molecular vibrations, making them indispensable.
"The ability to detect smaller concentrations of analytes can lead to breakthroughs in drug development and disease diagnostics, showcasing the truly transformative nature of these advancements in detector technology."
Improvements in Software Algorithms
The software that processes spectral data is just as critical as the physical components of the machine itself. Recent innovations have led to significant improvements in algorithms used for spectral analysis. These sophisticated algorithms allow for better peak identification, noise reduction, and resolution enhancement. Moreover, advancements in machine learning techniques are being harnessed to interpret complex spectral data more accurately.
These algorithms don’t just make data processing faster; they enable automated interpretations that can analyze larger datasets than humanly possible. For instance, software now can identify subtle spectral shifts or overlapping peaks that would normally require meticulous manual analysis. Such capabilities not only save time but also reduce the potential for human error, which is a significant issue in high-stakes areas like clinical diagnostics.
Integration with Other Analytical Techniques
The integration of infrared spectroscopy with other analytical methods has blossomed, creating powerful multimodal platforms. For example, coupling infrared spectroscopy with techniques like mass spectrometry or chromatography enhances the analysis of complex samples. This multidisciplinary approach is instrumental in fields where understanding material properties at a molecular level is key.
Such integration allows for a holistic view of the sample being studied. It can reveal a wealth of information about not only the chemical structure and composition but also the functional properties of materials in real-time. The collaborative nature of these innovations is indicative of a trend towards more comprehensive analytical protocols in scientific research.
With infrared spectroscopy witnessing such transformative changes, its future remains bright. The ongoing developments lays down the groundwork for exciting applications in not just research settings, but also industries such as pharmaceuticals, environmental science, and materials engineering. Through continued exploration and technological advancements, infrared spectroscopy will undoubtedly further solidify its role as a pivotal tool in scientific innovation.
Challenges and Limitations
The realm of infrared spectroscopy machines is certainly expansive, but like any technology, it doesn’t come without its hurdles. A clear understanding of these challenges is crucial not only for developing better systems but also when interpreting results and integrating these machines into various settings. Acknowledging the limitations helps delineate the advantages, guiding researchers and professionals in making informed choices regarding spectral analysis.
Environmental Factors Affecting Results
One of the significant issues that can impact infrared spectroscopy results is the environment in which the analysis takes place. Variations in temperature, humidity, and even air quality can skew data considerably. For instance, moisture in the air can absorb infrared light, leading to erroneous readings. When conducting experiments, it becomes paramount to
- Control temperature fluctuations
- Monitor humidity levels
- Maintain a clean environment free from external interferences
By establishing rigorous protocols for sample analysis, researchers can minimize these influences, but it can often complicate the practical deployment of IR spectroscopy machines, particularly in field studies or industrial settings.
Complexity of Sample Matrix
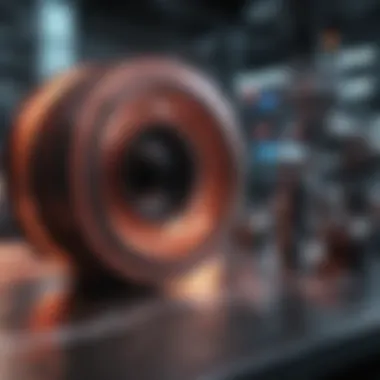
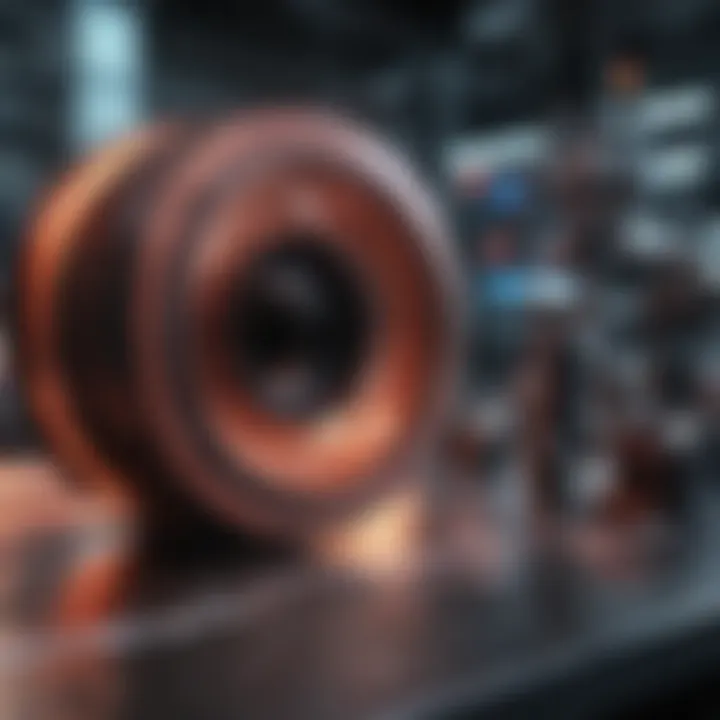
Another challenge worth unpacking is the complexity of the sample matrix itself. Non-homogeneous mixtures can produce overlapping spectral bands, making it a daunting task to extract precise information. For instance, in biological analyses, a single sample could consist of various components—proteins, lipids, carbohydrates—and each of these can absorb infrared light differently. This variability necessitates advanced data processing algorithms and sometimes requires expert spectral interpretation.
To tackle this issue, various approaches can be undertaken:
- Pre-sample treatment: Techniques like filtration or centrifugation might help to isolate specific components, thereby simplifying the spectral analysis.
- Use of chemometrics: By employing statistical analysis, researchers can better predict the outcomes and enhance the interpretive capacity of the data obtained from complex mixtures.
Cost of Advanced Systems
Lastly, the cost involved in procuring advanced infrared spectroscopy systems represents a barrier that many institutions face. High-end machines offer unrivaled sensitivity and specificity but come with a hefty price tag. The investment in such technology can be difficult for small labs or educational institutions with limited funding.
To navigate this barrier, institutions often consider
- Funding opportunities: Grants and partnerships with industry can alleviate some financial constraints.
- Shared resources: Collaborating with other institutions can make advanced systems accessible by sharing usage and maintenance costs.
Ultimately, while the challenges of environmental factors, complex sample matrices, and high costs are significant, they also push for greater innovation in the field. As researchers produce better, cost-effective methods of IR spectroscopy, the limitations can become stepping stones toward advancements rather than mere obstacles.
Future Directions in Infrared Spectroscopy
The realm of infrared spectroscopy is continuously evolving, which makes its future both promising and intriguing. As technology progresses, the potential for new applications, enhanced accuracy, and integration with other technologies grows. This section explores some noteworthy directions that infrared spectroscopy is taking, highlighting emerging applications, automation possibilities, and the expanding scope of research.
Emerging Applications in Industry
Infrared spectroscopy has found solid footing across various industries, but new applications are continually being researched. For instance, in the field of pharmaceuticals, the technology is increasingly employed for real-time monitoring of drug composition and purity. This ensures that medications produced are not only safe but effective for consumers. Furthermore, industries dealing with materials science are utilizing infrared spectroscopy to develop advanced polymers with tailored properties. The versatility of this technique means it can adapt to different environments and needs.
- Food Industry: In quality control, infrared spectroscopy can assess the moisture content and overall composition of food products, ensuring standards are met.
- Environmental Monitoring: It's being used to detect pollutants in air and water, which is crucial for safeguarding public health.
- Forensic Science: The application in crime scene investigations to analyze substances quickly has shown significant promise.
Potential for Automation and AI integration
As industries strive for efficiency, the integration of automation and AI with infrared spectroscopy represents a significant leap forward. Automated machines can conduct analyses with speed and precision that far exceeds manual methods. This revolutionizes data acquisition, allowing practitioners to handle more samples with reduced human error. Moreover, AI algorithms could enhance spectral data interpretation, learning from previous data to provide quicker and more accurate assessments. This ability to adapt and improve could lead to revolutionary changes in both academia and industry. The promise of reduced costs and increased throughput is a clear benefit.
"The intersection of AI and infrared technology can redefine our approach to analysis, harnessing the power of intelligence to interpret complex data patterns."
Expanding Research Opportunities
The future of infrared spectroscopy holds numerous avenues for research. As scientific inquiries delve into the molecular intricacies of materials, new methods and improvements in existing techniques are always in demand. For instance, studies are being conducted on combining infrared spectroscopy with imaging techniques to gain a more detailed understanding of sample morphology at the microscale. This richer data set could have vast implications in material science and biology, enabling researchers to visualize interactions at the molecular level.
- Interdisciplinary Research: The potential for convergence with fields like nanotechnology and biotechnology opens the doors for novel applications, such as in drug delivery systems.
- Sustainability: With a global emphasis on sustainability, infrared spectroscopy can also be pivotal in analyzing renewable resources and improving waste management processes.
- Custom Development: Researchers are exploring tailored solutions specific to industrial needs, which can lead to breakthrough innovations in various sectors.
The future trajectory of infrared spectroscopy is lined with opportunities that require not only industry adaptation but also academic engagement to grasp its full potential. The blending of established principles with cutting-edge innovations creates a fertile ground for exploration and discovery.
Ending
In wrapping up our discussion on infrared spectroscopy machines, it's crucial to highlight their far-reaching implications in a multitude of scientific fields. These machines epitomize a blend of innovation and functionality, proving instrumental in both academic research and practical applications in various industries. Ultimately, understanding the nuances of these tools not only enhances our grasp of scientific inquiry but also elevates the quality of decision-making processes in chemistry, biology, and materials science.
Summary of Key Points
To solidify the insights gained, let’s quickly recap the key elements covered in this article:
- Fundamental Principles: Infrared spectroscopy operates on the principle that certain molecules absorb infrared light at specific wavelengths, revealing invaluable information about their structure and composition.
- Machine Components: Critical parts such as light sources, detectors, and sample holders work in harmony to generate and interpret spectra.
- Operational Mechanisms: A thorough understanding of sample preparation and spectral data acquisition ensures accuracy and efficiency in data analysis.
- Diverse Applications: From chemical analyses to biological investigations, the range of applications underscores the machine’s versatility.
- Innovative Advancements: Recent technological improvements, including advanced detectors and software enhancements, are expanding the potential of infrared spectroscopy.
- Challenges: Awareness of limitations such as environmental variables and sample complexities can guide researchers in mitigating errors in results.
- Future Directions: The integration of AI and automation promises to transform how infrared spectroscopy is utilized, making it more accessible and efficient.
This comprehensive view not only lays out the present state of infrared spectroscopy but also hints at promising avenues for future exploration.
Impact on Scientific Research
The significance of infrared spectroscopy machines in scientific research cannot be overstated. They facilitate a deeper understanding of material properties, leading to enhanced methodologies in various disciplines. For example, in chemistry, they enable the precise identification of chemical substances, which is crucial in drug formulation processes. In biological settings, these machines aid in analyzing biomolecules, thus advancing knowledge in healthcare and therapeutics.
Moreover, the ability to conduct non-destructive testing means that valuable samples can be analyzed without compromising their integrity. The implications for sustainability and efficiency in research are substantial, as researchers can make informed decisions based on accurate data without wasting resources.
"The role of infrared spectroscopy in research is akin to a compass in the vast ocean of scientific discovery—guiding the way forward with clarity and precision."
Furthermore, as newer applications emerge, such as in environmental monitoring and food safety, the relevance of these machines continues to grow. In a world increasingly driven by data and analytics, the insights provided by infrared spectroscopy play a pivotal role in enlightening the path of scientific advancement. The integration of innovative technologies alongside these machines ensures they will remain at the forefront of research endeavors for years to come.
Thus, by recognizing their importance, we also affirm our commitment to enhancing research and exploring new horizons in various scientific fields.