Interference Microscopy: A Detailed Exploration
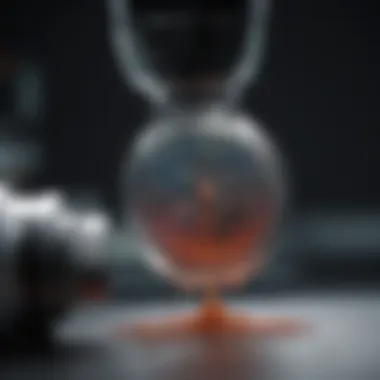
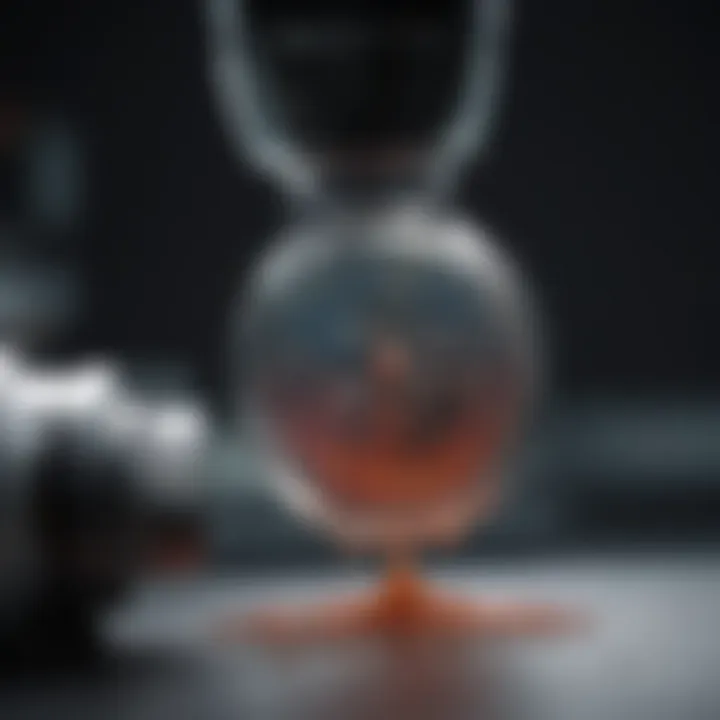
Intro
Interference microscopy stands at the forefront of optical imaging technology. It offers scientists a sophisticated method to visualize microscopic structures with remarkable precision. This technology leverages the principles of light interference to create contrast in samples that may be otherwise difficult to distinguish. By manipulating light waves, researchers can extract valuable information about the composition and arrangement of materials, making interference microscopy an essential tool across various disciplines.
As we explore this topic, we will unravel the fundamental principles that govern interference microscopy, examine significant historical milestones, and discuss recent advancements in techniques and applications. The insights provided here will enrich the knowledge of students, researchers, educators, and professionals who seek to understand both the theoretical and practical implications of this technology.
Understanding the role of interference microscopy in contemporary scientific research not only highlights its importance but also opens up possibilities for future innovations.
Fundamentals of Interference Microscopy
Understanding the fundamentals of interference microscopy is crucial as it forms the backbone of advanced optical imaging techniques. The fundamental principles guide the techniques and applications discussed in this article. Interference microscopy not only enhances resolution but also provides important insights into the optical properties of various samples, making it a versatile tool in many scientific fields.
Definition and Principle
Interference microscopy is an optical imaging technique that exploits the principles of light interference to enhance the contrast of microscopic samples. This method involves splitting light into two components, which then interact with the sample. The resulting interference pattern allows for the visualization of structures that are otherwise challenging to discern with standard microscopy methods.
The core principle is based on the superposition of light waves. When two or more waves overlap, they can reinforce or cancel each other out, producing bright and dark regions in the resulting image. This effect is particularly useful in observing transparent specimens or those with minimal inherent contrast. By analyzing the interference patterns, one can extract valuable quantitative data about the sample's thickness, refractive index, and morphology.
Historical Context
The development of interference microscopy traces back to the late 19th century, with significant contributions from scientists such as Ernst Abbe and Albert Michelson.
Abbe's work on optical resolution laid the groundwork for many modern microscopy techniques. Michelson's exploration of light interference led to the creation of the Michelson interferometer, a fundamental device that paved the way for various interference microscopy methods.
In the early 20th century, advancements continued with the introduction of phase-contrast microscopy by Frits Zernike, who was awarded the Nobel Prize in Physics in 1953 for his innovation. Zernike's technique allowed scientists to visualize live cells in greater detail, significantly impacting biology and medical research. The evolution of interference microscopy has since led to a diverse range of techniques used in microscopy today.
Types of Interference Microscopy Techniques
In the realm of optical imaging, understanding the different types of interference microscopy techniques is crucial. These methods provide unique insights into the microscopic world, allowing researchers to visualize structures that may otherwise remain hidden. Each technique has its own set of advantages and is suitable for specific applications. By appreciating these distinctions, one can select the most appropriate method for their research needs.
Phase Contrast Microscopy
Phase contrast microscopy is a revolutionary technique that enhances the contrast in transparent specimens. Utilizing phase shifts in light waves, it transforms phase differences into amplitude differences. This process allows for the visualization of cellular structures, such as organelles, without the need for staining or other invasive methods. This technique is particularly beneficial in biology and medical research, where live cells can be observed in their natural state.
Benefits of Phase Contrast Microscopy:
- Enables observation of living cells
- Maintains specimen integrity
- Provides high-contrast images of transparent samples
However, phase contrast microscopy comes with considerations. Not all specimens are suitable for this technique, and the results can be affected by optical aberrations. Therefore, understanding the limitations is vital for effective application.
Differential Interference Contrast () Microscopy
Differential Interference Contrast (DIC) microscopy builds upon the principles of phase contrast. This method adds complexity by employing polarized light to create a three-dimensional effect in images. DIC is particularly effective for visualizing the internal structures of biological samples and offers a more detailed view compared to standard phase contrast.
Advantages of Microscopy:
- Provides excellent optical sectioning
- Enhances depth perception of specimens
- Useful for observing fine details in thick samples
While DIC microscopy is versatile, it also has specific requirements for sample preparation, including the need for thin sections. Additionally, this technique may not be ideal for all types of samples due to the potential for birefringence affecting image quality.
Interference Reflection Microscopy
Interference reflection microscopy is another technique that has gained traction, particularly in material science. This method utilizes the interference of light reflected from a sample surface to produce high-resolution images. It can effectively visualize thin films and surface structures at the nanoscale.
Key Features of Interference Reflection Microscopy:
- High sensitivity to surface features
- Capable of imaging thin films and layers
- Non-invasive method for surface characterization
Nonetheless, this method may be limited in its ability to penetrate deeper into samples compared to other techniques. Thus, it is essential to choose this method based on the specific characteristics of the samples being studied.
In summary, each interference microscopy technique offers distinct advantages and limitations. The choice of method can significantly impact the outcome of experimental results, making it crucial for researchers to understand these nuances.
Instrument Components and Setup
Understanding the instrument components and setup is vital for effective use of interference microscopy. Each component plays a crucial role in achieving high-quality imaging and accurate results. When we set up an interference microscope, attention to detail in these components can make a substantial difference in performance and outcomes.
Light Sources
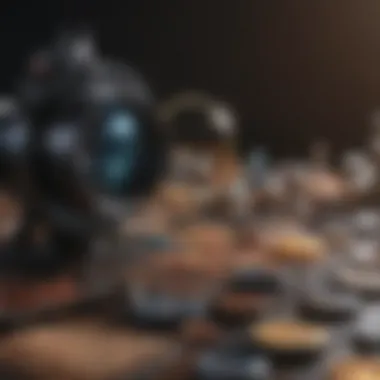
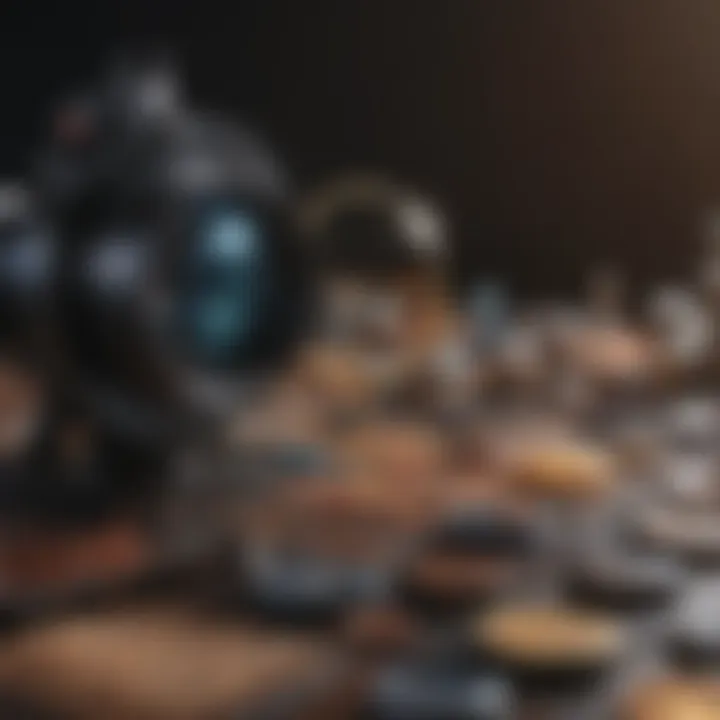
Light sources are foundational elements of interference microscopy. Various light sources produce the necessary illumination for the sample. Common options include LED sources, halogen lamps, and lasers. Each type of light source has unique qualities regarding wavelength, intensity, and stability. For example, lasers provide coherent light that significantly improves sensitivity and resolution in imaging.
In constructing a microscope setup, selecting the right light source is crucial for enhancing contrast and distinguishing fine details in samples. Users must consider the specific requirements of their samples. For many applications, a monochromatic light source improves the generation of distinct interference patterns, thus achieving better imaging quality.
Optical Filters
Optical filters are indispensable in interference microscopy, as they help to refine the light spectrum reaching the sample. These filters can either attenuate unwanted wavelengths or enhance specific bands of light. The use of bandpass filters is common, ensuring that only the desired wavelengths contribute to the imaging process.
The incorporation of optical filters allows researchers to reduce noise and enhance signal clarity. This can be particularly beneficial when working with fluorescent samples or in cases where background illumination may interfere with observations. When setting up, ensure to choose filters that align with the light source and sample characteristics to maximize imaging capability.
Image Acquisition Systems
An effective image acquisition system is the final piece of the instrument setup. These systems capture and digitize the images produced by the microscope for analysis and interpretation. Quality image acquisition systems convert light signals into digital data, allowing for advanced processing and visualization.
Modern setups often involve high-resolution cameras, such as CCD or CMOS sensors. They provide superior noise reduction and sensitivity compared to older systems. Also, the integration of software solutions for image processing can significantly enhance the clarity and utility of captured images, offering tools for quantitative analysis.
Overall, the careful selection and integration of these instrument components lead to a more robust understanding of the samples being observed. It is important to recognize how each element contributes to the overall performance of interference microscopy.
Remember, a well-setup microscope is critical for achieving reliable and high-quality results.
Mathematical Formulation
The mathematical formulation of interference microscopy is vital for understanding the underlying principles and quantifying the observed phenomena. This section lays out the theoretical framework necessary for grasping how interference microscopy operates, which is crucial for students and researchers aiming to utilize this technique effectively in various applications.
Interference Patterns
Interference patterns are the result of the superposition of light waves, and they play a key role in interference microscopy. When two or more coherent light waves meet, they interfere with each other. Depending on their phase relationship, they can either amplify or diminish the light intensity. This interference between waves leads to the formation of alternating bright and dark fringesβknown as fringe patternsβwhich provide significant information regarding the specimen being observed.
The clarity and detail of these interference patterns depend on several factors, such as the wavelength of light, the angle of incidence, and the optical path length differences between the waves. For example:
- Coherence: High coherence between the light sources is necessary for clear and stable patterns.
- Wavelength: Different wavelengths can generate various fringe spacings, affecting the resolution of the observed structures.
- Optical path differences: Variations in specimen thickness or refractive index lead to changes in light travel paths, thus influencing the interference outcomes.
The ability to analyze and interpret these interference patterns is fundamental, as they can indicate information about morphological and structural characteristics of the sample. This mathematical aspect enables researchers to quantitatively assess properties such as cell shape or the refractive index of materials with enhanced contrast.
Phase Shift and its Measurement
Phase shift refers to the difference in phase between two light waves that is induced by various optical conditions. In interference microscopy, measuring phase shifts is crucial for acquiring comprehensive data about the specimen. Phase contrasts are especially important in observing transparent or semi-transparent objects, where conventional contrast techniques may fail.
The phase shift can occur due to:
- Reflection: Light reflecting off surfaces can induce a phase change.
- Refraction: When light passes through materials of different refractive indices, it experiences a change in speed, leading to a phase shift.
To quantify phase shifts, researchers often use mathematical formulas derived from wave optics, such as:
- Heisenberg uncertainty principle, which aids in understanding the limits of accuracy in measurement, relevant to phase determinations.
- Fourier transforms, enabling the analysis of complex interference signals and distinguishing various components contributing to the observed phase shifts.
Accurate measurement of phase shifts ultimately allows for enhanced resolution of spatial structures and dynamics within the sample.
"Phase shifts in light waves are more than just factors in calculations; they are fundamental in revealing the hidden details of microscopic structures."
In summary, the mathematical formulation of interference patterns and phase shift offers a robust framework for analyzing microscopy results. This understanding equips scientists to push boundaries in various fields, leading to new discoveries and advances in research.
Sample Preparation Techniques
Sample preparation is a crucial aspect in the success of interference microscopy. It directly influences the quality of the images obtained and the accuracy of the observed data. Proper preparation techniques ensure that the samples are in optimal condition to minimize distortion and enhance the visibility of microscopic features. This section explores various sample preparation techniques applicable in interference microscopy, focusing on their significance, advantages, and considerations.
Mounting Methods
Mounting methods are foundational in the preparation of samples for interference microscopy. The purpose of these methods is to stabilize the sample and maintain its integrity during examination. One common technique involves the use of a medium, such as a mounting gel or resin, which is chosen based on the specific property of the sample. For instance, biotic samples often require a medium that preserves live cellular structures while avoiding toxicity.
A few significant mounting methods include:
- Coverslide Mounting: This method uses a cover slip to hold the sample in place, which is particularly useful for thin specimens.
- Embedding in Resin: This technique is beneficial for samples that require structural integrity, such as hard biological materials. The resin hardens and provides support during observation.
- Gel Mounting: This method is suitable for live samples, as certain gels can preserve cell viability while providing physical support.
Each of these methods comes with benefits, such as ease of use and improved durability. However, choosing the appropriate mounting technique requires thoughtful consideration of the sample type and observation goals.
Common Sample Types
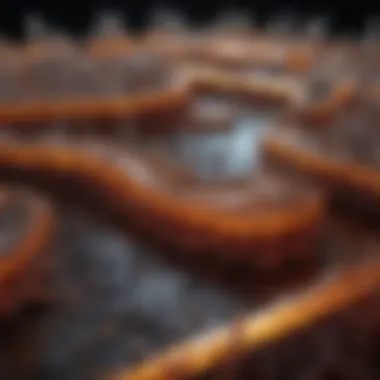
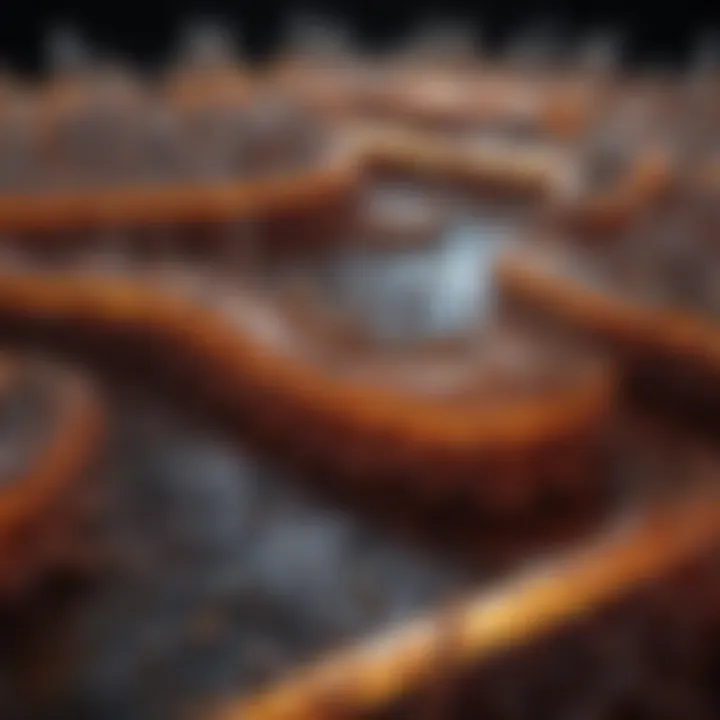
Understanding the common types of samples prepared for interference microscopy is essential for researchers in diverse fields. The sampling choices directly reflect the intended focus of the investigation. Some prevalent sample types include:
- Biological Samples: These may involve cells, tissues, or microorganisms, which are often assessed for structural and functional properties.
- Material Samples: This category covers metals, polymers, and composites, which might be investigated to understand properties like surface roughness or material defects.
- Nanostructured Materials: These samples are significant in nanotechnology, where the focus is on the intricate details of materials that can only be viewed at microscopic levels.
When preparing samples, consideration must be given to the specific requirements of interference microscopy. Factors such as the refractive index of the sample and the mounting medium can significantly affect the results.
Properly prepared samples lead to clear imaging and reliable data, thus influencing the entire research outcome.
Applications in Scientific Research
Interference microscopy has made significant contributions to various fields of science. Its ability to provide detailed imaging without the need for fluorescent labeling or heavy sample preparation is essential. Applications extend from biological studies to materials research, showcasing the versatility of this technique. In this section, we will explore its role in biological and cellular imaging, material science investigations, and the characterization of nanotechnology. The insights gained from these applications highlight the importance of interference microscopy in advancing scientific understanding.
Biology and Cellular Imaging
In biology, understanding cellular structures and functions is crucial. Interference microscopy enables researchers to visualize live cells in real-time without causing damage. This non-invasive approach allows for the study of physiological processes with minimal interference from external factors. Researchers can observe cell movements, division, and other dynamic activities. Such real-time imaging is valuable in developmental biology and cancer research, as it reveals how cells interact in their natural environment.
Some key benefits of using interference microscopy in biology include:
- Non-destructive imaging: Cells can be observed without the need for staining or fixation, preserving their natural state.
- Enhanced contrast: The technique provides better contrast than traditional bright-field microscopy, aiding in the differentiation of structures within cells.
- Live-cell imaging: This method allows for the observation of biological processes as they happen, offering valuable insights into cellular dynamics.
Material Science Investigations
Material science relies on precise characterization of materials to innovate and improve properties. Interference microscopy has become an indispensable tool in this domain. It helps in analyzing thin films, coatings, and surface textures. Researchers use this technique to gauge surface roughness and to examine microstructures at a high resolution.
Some important aspects include:
- Surface profiling: Interference microscopy can measure surface features and variations effectively, providing data on the material's topology.
- Quality control: In manufacturing processes, this technique assists in ensuring that materials meet specific quality standards by detecting defects that may not be visible by other means.
- Advanced imaging capabilities: It allows the analysis of optical properties such as refractive indices, contributing to better material design.
Nanotechnology Characterization
As nanotechnology continues to evolve, characterization methods must advance to keep pace. Interference microscopy provides a non-invasive way to assess nanomaterials, such as nanoparticles and nanostructured surfaces. By analyzing these materials, researchers gain insights into their properties, which are paramount for applications in electronics, medicine, and photonics.
Key points related to nanotechnology characterization include:
- High-resolution imaging: This technology can reveal fine details of nanostructures that are essential for understanding their behavior and interactions.
- Scalable analysis: It is adaptable for analyzing both small-scale laboratory samples and larger specimen sizes, providing flexibility in experimental setups.
- Interdisciplinary relevance: The ability to characterize nanomaterials is critical across multiple industries, promoting innovations in fields like drug delivery systems and nanoelectronics.
The ability of interference microscopy to bridge theoretical concepts with practical applications underscores its significance in scientific research.
In summary, the applications of interference microscopy in scientific research are vast and deeply impactful. From observing live cells to analyzing materials at the nanoscale, this technique is integral to modern advancements in multiple fields.
Comparison with Other Microscopy Techniques
Understanding how interference microscopy relates to other microscopy techniques is key to appreciating its unique capabilities. In scientific research, the choice of microscopy methods heavily influences the quality and type of data that can be obtained. Hence, it is important to consider not only how interference microscopy excels but also how it compares with techniques like fluorescence microscopy and scanning electron microscopy (SEM).
Fluorescence Microscopy
Fluorescence microscopy uses fluorescent stains to highlight specific components of biological samples. This technique relies on the natural ability of certain molecules to absorb light at a specific wavelength and then emit it at a longer wavelength. The primary advantage of fluorescence microscopy is its capability to visualize structures and processes in living cells in real-time. This allows researchers to observe dynamic cellular processes within their natural environment.
However, it has significant limitations. The resolution is often restricted by factors like photobleaching, where the fluorescent signal diminishes over time, and background fluorescence, which can obscure important details. Interference microscopy, on the other hand, does not rely on fluorescence. Instead, it enhances contrast without staining, thus preserving the natural state of the sample. This makes it suitable for visualizing structures that would otherwise be difficult to observe under fluorescence techniques, particularly in thick specimens.
Scanning Electron Microscopy (SEM)
Scanning electron microscopy provides remarkable detailed images of the surfaces of samples. SEM generates high-resolution, three-dimensional images by scanning a focused beam of electrons over the specimen. The interaction between the electron beam and the sample produces information that can be detected and transformed into images with impressive topographical details.
Despite its strengths, SEM has notable drawbacks. Firstly, it requires samples to be dehydrated and plated with a conductive coating, which can alter the natural morphology of biological samples. The preparation process can also introduce artifacts.
In contrast to SEM, interference microscopy can visualize samples in a near-native state without extensive preparation. This property is especially advantageous for biological specimens, where maintaining physiological conditions is crucial.
Interference microscopy provides the ability to obtain high contrast images of samples with a resolution that is adequate for many biological and material science applications. Its compatibility with live-cell imaging offers an important advantage where dynamic processes are of interest.
Both fluorescence microscopy and SEM serve important roles in specific contexts, yet interference microscopy's unique features and capabilities expand the toolbox available to researchers. It shines particularly in applications requiring the observation of live samples or in scenarios where sample preparation can be a significant obstacle. Understanding these distinctions helps in selecting the appropriate method for given research objectives.
Limitations and Challenges
Understanding the limitations and challenges of interference microscopy is crucial for researchers and users. While the technique offers remarkable imaging capabilities, it is not without its drawbacks. Researchers must navigate these challenges to draw accurate conclusions in their work. This section explores two primary challenges: resolution limitations and sample compatibility issues.
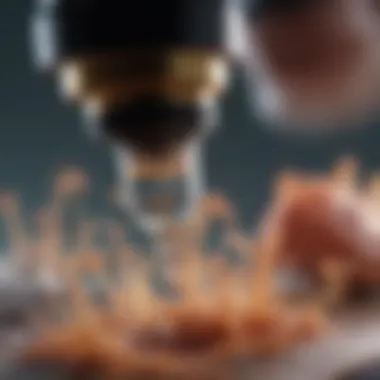
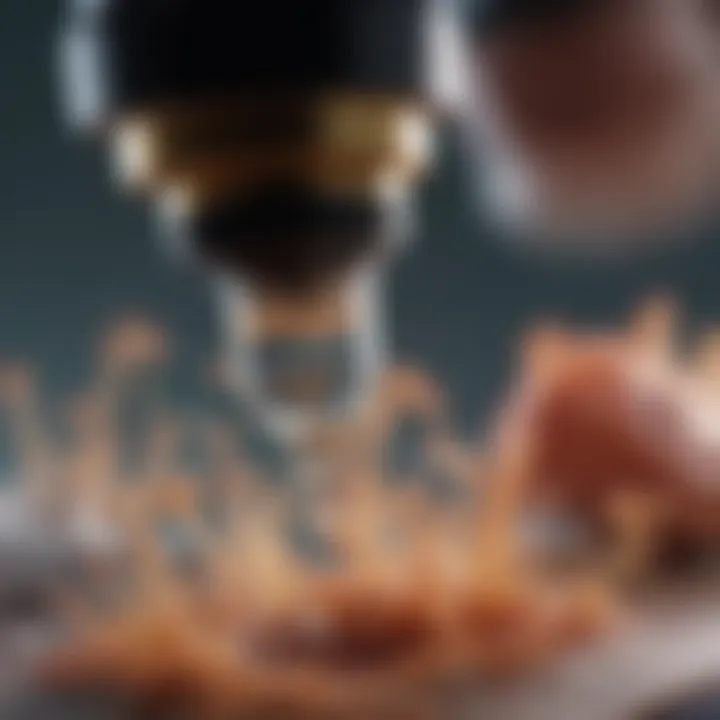
Resolution Limitations
Resolution limitations in interference microscopy are centered around the inherent capabilities of the optical system. The resolution defines the smallest detail that the system can distinguish. Interference microscopy utilizes light waves to form images, but diffraction limits play a significant role. Simply put, when light waves pass through small apertures or around small objects, they spread, which can create blurriness in the images. This can impose constraints, particularly when examining fine biological structures or materials at the nanoscale.
Key aspects to consider include:
- Wavelength Dependence: The wavelength of light used in the microscopy impacts resolution. Shorter wavelengths can yield better resolution, but in practical situations, there are limitations on the light sources available.
- Optical Aberrations: Imperfections in lens systems can further degrade image quality. These aberrations can lead to inaccuracies in measurements or evaluations of structures.
- Quantitative Analysis: When it comes to quantitative metrics derived from images, resolution constraints can reduce the reliability of results. This limitation can negatively affect studies requiring precise data.
Sample Compatibility Issues
Sample compatibility is another significant challenge in interference microscopy. Not all samples can be prepared or imaged effectively using this method. The success of interference microscopy is heavily dependent on the optical properties of the sample, specifically the refractive index contrast between the sample and its surroundings.
Consider the following factors:
- Refractive Index Variability: Samples with low contrast refractive indexes with the mounting media can lead to poor image quality. Similar indices can make it difficult for the interference effects to be discerned, limiting the method's applicability.
- Preparation Constraints: Some samples require specific treatments or mounting methods that may alter their natural state. This alteration can diminish the authenticity of the findings and hinder reproducibility in experiments.
- Thickness Limitations: Thick samples present challenges in imaging due to light scattering and attenuation. This can obscure critical details or lead to incorrect interpretations of morphology.
"Understanding these limitations not only influences experimental design but also guides the interpretation of results in research and development domains."
In summary, while interference microscopy is a potent tool for imaging, it carries inherent limitations in resolution and sample compatibility. A profound awareness of these challenges aids researchers in navigating the intricate landscape of microscopic analysis.
Future Directions in Interference Microscopy
The landscape of interference microscopy is evolving rapidly. As researchers delve deeper into the applications and refinements of this imaging technology, it becomes crucial to explore the future potential. Focusing on technological innovations and new applications will reveal the direction in which the field is heading. This exploration not only informs the scientific community but also sets the stage for transformative advancements in various domains.
Technological Innovations
Technological innovations in interference microscopy are key to enhancing its capabilities and usability. One notable trend is the development of improved optical components. Advances in optical filters and high-resolution cameras contribute significantly to image quality. These innovations allow for clearer and more detailed visualization of microscopic structures.
Furthermore, new algorithms for image processing are facilitating analysis at an unprecedented scale. Tools that utilize artificial intelligence and machine learning are becoming increasingly integrated into microscopy workflows. This enables researchers to analyze complex data sets more efficiently. Modern systems also emphasize automation. Automated microscopy platforms reduce human error and improve throughput.
In addition, the integration of multimodal imaging approaches is also on the rise. This allows scientists to combine interference microscopy with techniques like fluorescence and electron microscopy, enhancing their research's depth. Such innovations can expand interference microscopy's overall applicability.
New Applications on the Horizon
As the technological infrastructure evolves, so too do the potential applications of interference microscopy. Areas such as the biomedical field are particularly promising. Researchers are currently utilizing interference microscopy to investigate cellular processes in real time. This includes tracking live cell dynamics and studying cellular interactions at a microscopic level.
Moreover, material science is witnessing new implementations. Interference microscopy is being applied to study thin films and nanostructures, providing insights into material properties. Such applications are vital for developing novel materials with tailored characteristics, applicable in electronics and nanotechnology.
Additionally, there is interest in environmental science, where interference microscopy can help analyze biological samples and pollutants in water. Such applications demonstrate the importance of this microscopy technique in addressing real-world issues.
The future of interference microscopy is not just about refining existing techniques; it is about exploring uncharted territories and harnessing the technology for a broad spectrum of scientific inquiries.
End and Implications
The discussion around interference microscopy is not merely academic; it holds significant implications for various scientific fields. This technology elevates our capacity to visualize microscopic structures with clarity and precision. It is vital for researchers and students to grasp how interference microscopy enhances the understanding of complex biological and material systems. The article has outlined various techniques, their applications, and the importance of proper setup and sample preparation.
This comprehension deepens the utilization of interference microscopy in practical scenarios, fostering advancements in research and education. Its limitations, while noteworthy, are not insurmountable.
"Interference microscopy serves as a bridge between theoretical concepts and practical applications, empowering enhanced analysis of microstructures."
Thinking about the future of interference microscopy prompts a conversation about technological innovations. Integrating modern technologies is essential to overcoming present limitations. As we have seen, advancements in imaging techniques, such as digital image processing and automation, can enhance research outcomes remarkably.
Moreover, with the ongoing exploration of new applications, the potential for interference microscopy to impact scientific research remains vast. By recognizing its relevance, researchers can leverage this tool in innovative ways, driving future discoveries across multiple disciplines.
As students and educators engage with this technology, it is critical to encourage a mindset of exploration and inquiry. The role of interference microscopy is not static; its evolution invites challenges and opportunities that could define the future of optical imaging.
Summary of Key Insights
In summary, interference microscopy is a pivotal tool that has transformed optical imaging. The article discussed its fundamental principles, historical context, and various techniques, along with the importance of proper setup and sample preparation.
Key points include:
- Enhanced Visualization: Interference microscopy provides greater clarity in imaging, revealing intricate details of specimens.
- Diverse Applications: Its usefulness spans various fields, notably in biology and material sciences.
- Technological Advancements: Recent innovations shape the future, suggesting that this field is far from stagnant.
- Challenges: Limitations exist, but they can be addressed through innovative solutions that enhance compatibility and resolution.
The Role of Interference Microscopy in Scientific Advancement
Interference microscopy plays an integral role in scientific advancement. Its ability to visualize complex structures at a microscopic level provides researchers with critical insights. These insights are essential for advancing knowledge in biology, materials science, and nanotechnology.
The techniques discussed allow for non-invasive imaging, preserving sample integrity while gathering data. In biological research, for instance, phase contrast and differential interference contrast microscopy facilitate the study of live cells without staining or altering the specimen. This capability is vital for understanding physiological processes in real-time.
Furthermore, material scientists utilize interference microscopy to examine the properties of materials at the nanoscale, enabling advancements in technology and engineering. This cross-disciplinary impact underscores the significance of mastering interference microscopy for future scientific explorations.
As researchers continue to push the boundaries, the intersection of technological innovation and traditional microscopy techniques will yield even deeper insights, affirming the importance of interference microscopy in the continuum of scientific progress.