Isolating DNA: Comprehensive Techniques and Applications
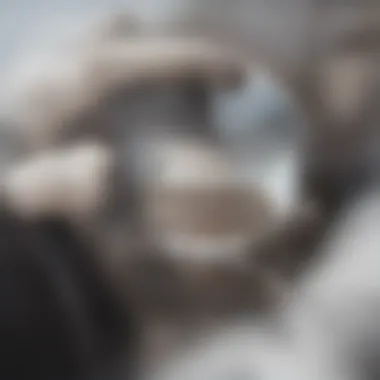
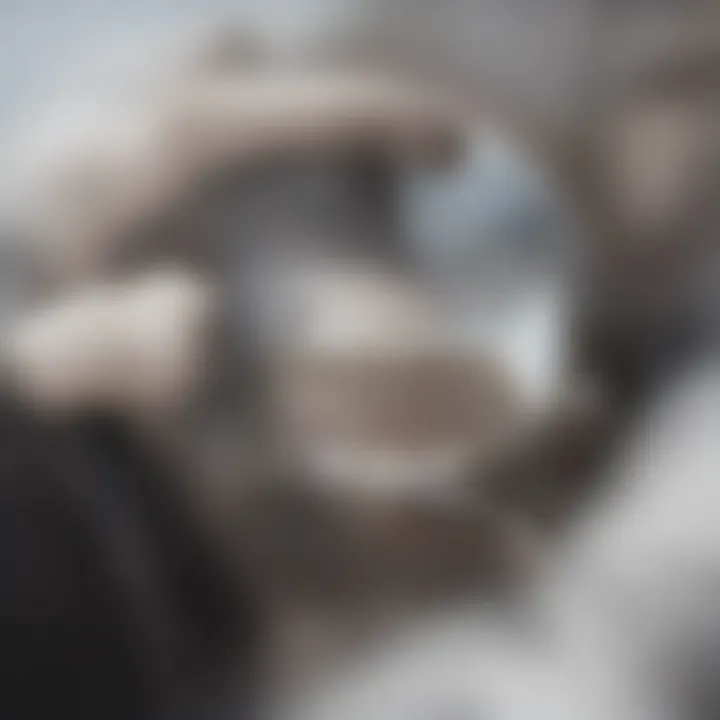
Intro
The isolation of DNA stands as a cornerstone in the realm of molecular biology. This process, integral to various scientific fields, enables researchers to manipulate and analyze genetic material with precision. Through discussing the isolation procedures, techniques, and their diverse applications, this article aims to clarify the significance of DNA extraction in both academic and real-world scenarios.
Understanding the steps to effectively isolate DNA is fundamental for genetic studies, biotechnology, forensic science, and even medical diagnostics. The methods outlined will provide insight into the underlying principles of DNA extraction, covering various sources from which DNA can be obtained—including plants, animals, and microorganisms.
This section will navigate through the relevant advances in the techniques used for DNA isolation, elucidating the remarkable progress that has transformed this critical procedure.
Intro to DNA Isolation
DNA isolation is a fundamental procedure in the field of molecular biology, serving as the backbone for various genetic analyses and applications. It involves extracting DNA from cells or tissues for further study, manipulation, or application in diverse scientific research and practical fields. This introduction aims to elaborate on the significance of DNA isolation, highlighting its central role in advancing genetics and biotechnology.
Definition of DNA Isolation
DNA isolation refers to the extraction of DNA from biological samples such as blood, plant tissues, or microbial cultures. The primary objective is to obtain a pure DNA sample that is free from proteins, lipids, and other cellular contaminants. Several methods exist for this process, each tailored to the type of sample and required purity.
One standard approach involves mechanical or chemical lysis, which breaks down cell membranes. This is followed by purification steps, which may include precipitating the DNA with alcohol or using silica-based methods to bind and wash the DNA, ultimately yielding a clean sample for analysis or experimental use.
Importance of DNA Isolation
The importance of DNA isolation cannot be understated. It is integral to many scientific disciplines—genetics, forensics, medical diagnostics, and conservation biology. Here are several key benefits of DNA isolation:
- Research and Analysis: Isolated DNA allows researchers to study genetic material, aiding in understanding genetic diseases, evolutionary biology, and species diversity.
- Forensic Science: In forensic investigations, DNA isolation is crucial for identifying individuals from biological samples, playing a fundamental role in criminal justice.
- Medical Applications: In diagnostics, isolating DNA enables the identification of pathogens or genetic markers associated with diseases, improving patient care and treatment responses.
- Biotechnological Developments: From genetic engineering to synthetic biology, isolated DNA is essential for creating genetically modified organisms and developing new bioproducts.
The reliability and accuracy of DNA isolation methods significantly impact the outcomes of downstream applications and research initiatives.
In summary, understanding DNA isolation is vital as it not only facilitates the fundamental research but also enhances practical applications that benefit society. By isolating DNA effectively, scientists can unlock answers to complex biological questions and contribute to technological advancements.
Historical Context of DNA Isolation
The historical context of DNA isolation is essential to understand the evolution of molecular biology and its significant impact on genetic research. Knowledge of how DNA was isolated has guided scientific progress in various fields, particularly in genetics and biotechnology. Through these developments, we learn how early discoveries laid the foundation for modern techniques and how advancements have broadened our understanding of DNA.
Early Techniques and Discoveries
The journey of isolating DNA began in the 19th century with the initial discoveries of the molecule itself. In 1869, Friedrich Miescher first isolated nucleic acid from pus cells, calling it "nuclein." However, it was not until the mid-20th century that DNA became a focus of intense study.
The first practical methods for extracting DNA were developed in the late 1940s and early 1950s. One notable method employed in early studies utilized simple detergents to lyse cells, allowing researchers to purify DNA. These techniques were rudimentary compared to today's standards but were crucial steps in identifying DNA as the material responsible for genetic inheritance.
In 1953, James Watson and Francis Crick famously elucidated the double-helix structure of DNA, a revelation that sparked widespread interest in genetic research. This structure was also a catalyst for improving extraction methods, enabling scientists to work more efficiently with DNA. Hence, early techniques for isolating DNA played a pivotal role in molding modern molecular biology.
Advancements in Molecular Biology
The latter half of the 20th century witnessed substantial advancements in molecular biology techniques. With the advent of polymers and more sophisticated solvents, the purity and yield of isolated DNA improved significantly. Techniques such as phenol-chloroform extraction became common, offering higher quality DNA suitable for various applications, including cloning and sequencing.
In the 1980s, the introduction of the polymerase chain reaction (PCR) revolutionized the field. PCR allowed for the amplification of specific DNA sequences from minimal starting material, demanding new, more efficient isolation methods. Additionally, the development of silica-based column purification methods facilitated the rapid and straightforward isolation of DNA, further pushing the boundaries of genetic research.
These advancements advanced not only the technical capabilities of DNA isolation but also facilitated broader applications in fields like forensics, medicine, and agriculture. The historical development of DNA isolation techniques highlights how foundational research can lead to groundbreaking innovations.
"The understanding and manipulation of DNA has changed the landscape of science, offering new insights into biology and medicine."
As methods continue to evolve, the historical context serves as a reminder of where DNA isolation began and how it has shaped contemporary scientific practices. Recognizing these historical milestones enriches the appreciation of current and future advancements in DNA isolation technology.
Fundamental Principles of DNA Chemistry
Understanding the fundamental principles of DNA chemistry is essential for anyone involved in molecular biology. These principles lay the groundwork for various techniques utilized in DNA isolation. A grasp of DNA structure and its chemical properties is indispensable not only for the effectiveness of extraction methods but also for ensuring the integrity of the isolated DNA.
Structure of DNA
The structure of DNA is pivotal for its function and isolation. DNA, or deoxyribonucleic acid, is a double helix formed by two long strands of nucleotides. Each nucleotide comprises three components: a phosphate group, a deoxyribose sugar, and a nitrogenous base. There are four types of bases—adenine, thymine, cytosine, and guanine.
The pairing of these bases occurs through hydrogen bonds, where adenine pairs with thymine, and cytosine pairs with guanine. This pairing leads to complementary strands, which are vital during DNA replication and isolation procedures.
The helical structure of DNA allows for compact storage of genetic material, while the sequence of its bases encodes the information necessary for protein synthesis and other cellular functions. When isolating DNA, it is crucial to maintain this structure to ensure that it retains its functional properties.
Chemical Properties Relevant to Isolation
Several chemical properties of DNA play significant roles in its isolation. DNA is a polyacid, meaning it carries a negative charge due to its phosphate backbone. This charge is crucial during the isolation process as it affects the interaction of DNA with various reagents.
- Solubility in Alcohols: One of the primary techniques for precipitating DNA involves the use of alcohol, typically ethanol or isopropanol. The addition of alcohol causes DNA to precipitate because its solubility decreases in these solvents. This process is a key step in obtaining pure DNA.
- Denaturation: During cell lysis, the application of heat or chemicals can lead to denaturation, where the hydrogen bonds between base pairs are disrupted. This denaturing process can be useful for breaking open the structure of the DNA, allowing for easier access during extraction.
- Affinity Interactions: Certain materials, such as silica, have a strong affinity for DNA. Techniques like silica gel membrane methods exploit this property, allowing for effective binding and separation during the purification stages.
Understanding these chemical properties is vital for developing robust methods of DNA isolation. Each property influences the efficiency of extraction and the quality of the purified DNA, impacting subsequent applications in various fields.
"The efficiency of DNA isolation techniques largely depends on fundamental chemical principles."
Grasping the principles of DNA chemistry equips researchers and technicians with the necessary knowledge to adjust and optimize protocols for successful DNA isolation.
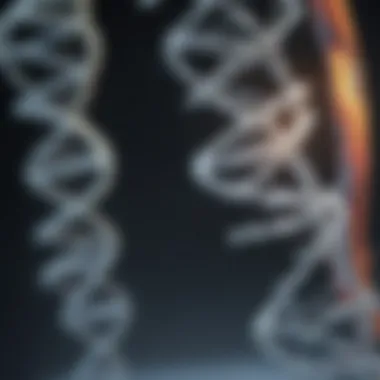
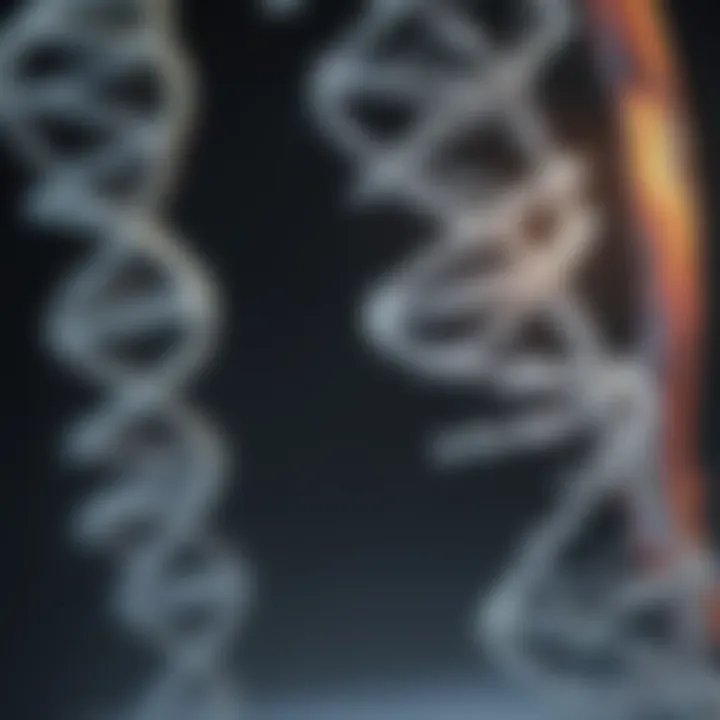
Sources of DNA for Isolation
Understanding the sources of DNA is critical in the process of DNA isolation. Different sources offer varying types of DNA, which can influence downstream applications. The selection process for the DNA source is pivotal, as it can affect the yield, quality, and purity of the isolated DNA. Furthermore, considerations regarding ethical implications, availability, and extraction techniques are essential.
Plant DNA Sources
Plant DNA isolation is an important area due to the abundance of plant genetic resources available worldwide. Plants provide a rich source of DNA, as they form the basis of various research and applications in genetics and biotechnology. The methods for extracting DNA from plants often require specific protocols to overcome the challenges posed by cell wall rigidity and the presence of secondary metabolites like polyphenols and polysaccharides that can interfere with DNA yield and quality.
- Common Sources: Leaf tissues and seeds are the most commonly used plant tissues for DNA extraction.
- Challenges: The presence of specific compounds can lead to contamination in the extracts, necessitating additional purification steps.
To isolate DNA from plants, methods like CTAB extraction or modified phenol-chloroform extraction are typically employed. These methods enhance the efficiency of breaking down cellular structures, ensuring maximum recovery of nucleic acids.
Animal DNA Sources
Animal DNA isolation is fundamental in numerous fields, including veterinary medicine, forensic science, and genetic research. Animal tissues provide a variety of DNA types depending on the source, such as blood, saliva, or tissue biopsies. The protocols for extracting DNA from animals differ substantially based on the type of tissue and the preservation method used prior to extraction.
- Common Sources: Blood and tissue samples (muscle, liver) are popular starting materials.
- Considerations: The presence of proteins and other contaminants in animal tissues can severely impact the DNA extraction process, requiring meticulous approaches to achieve consistent results.
Techniques such as the use of commercial kits based on silica membrane technology have streamlined DNA isolation from animal sources, providing researchers with reliable and efficient methods while minimizing contaminants.
Microbial DNA Sources
Microbial DNA isolation presents unique opportunities in the study of soil, water, and various environments' microbial communities. Microbes, including bacteria and fungi, contribute significantly to ecological and biological research. The methods used for isolating DNA from microbial sources can widely vary, considering the diversity of microbial structures and environmental conditions.
- Common Sources: Soil samples and cultured microbial strains are frequently used for DNA studies.
- Challenges: The presence of inhibitors such as humic substances in environmental samples can affect the PCR amplification of extracted DNA.
Advancements in methods like bead-beating and enzymatic lysis have improved the efficiency of extracting high-quality DNA from microbial sources. Proper protocols are essential to ensure the obtained DNA reflects the microbial complexity and diversity present in the original sample.
Common Techniques for DNA Isolation
Understanding the common techniques for DNA isolation is fundamental in molecular biology. These methods are vital because accurate DNA extraction is crucial for various applications, including genetic analysis, forensic science, and medical diagnostics. Scientific precision requires reliable techniques to ensure the purity and yield of isolated DNA.
Different methodologies each come with specific advantages and limitations. By knowing the characteristics of each technique, researchers can select the most suitable option based on the type of sample, desired purity, and subsequent application.
Moreover, these techniques often overlap in usage or can be modified for specific needs, which enhances their versatility in the laboratory setting. Below, we outline the most common DNA isolation methods employed in scientific research.
Phenol-Chloroform Extraction
Phenol-chloroform extraction is a classical method used for isolating DNA from biological samples. This technique relies on the partitioning between aqueous and organic phases, allowing for effective separation of nucleic acids from proteins and lipids. The procedure involves mixing the biological sample with phenol and chloroform, leading to the formation of two distinct layers: an upper aqueous layer containing the DNA and a lower organic layer with proteins and other contaminants.
- Benefits:
- Considerations:
- High purity of isolated DNA.
- Effectiveness in removing proteins and RNA.
- Use of hazardous chemicals requires careful handling and appropriate safety measures.
- Time-consuming compared to newer methods.
This method is a go-to for many researchers who require high-quality DNA for subsequent analyses, such as PCR amplifications or cloning.
Silica Gel Membrane Methods
Silica gel membrane techniques offer a more modern approach to DNA isolation. These methods exploit the binding properties of silica under specific conditions. When a lysate is passed through a silica column, DNA binds to the silica surface while contaminants are washed away. After thorough washing, DNA is eluted in a buffer solution.
- Benefits:
- Considerations:
- Quick and efficient, often yielding results within a few hours.
- Suitable for various sample types, including blood, tissue, and microbial lysates.
- Requires the purchase of specific kits, which can be costly.
- Binding conditions must be optimized for different sample types.
Silica gel membrane methods are prevalent in laboratories earning a balance between speed and efficiency, making them ideal for high-throughput environments.
Column Purification Techniques
Column purification techniques are similar to silica gel methods but can include various materials and strategies for DNA extraction. These techniques often utilize columns packed with materials that selectively bind DNA based on size or charge. The choice of matrix may vary, including silica, resin, or activated charcoal.
- Benefits:
- Considerations:
- High throughput applications are feasible due to easy scaling of the process.
- Offers flexibility to choose the appropriate column matrix based on the sample type.
- Quality of the isolated DNA can vary based on the binding conditions and materials used.
- Initial costs may be high, similar to other kit-based methods.
Column purification has become increasingly popular for both routine and specialized DNA isolation tasks, providing reliable results across a range of biological materials.
In summary, each technique for DNA isolation brings unique benefits and limitations, requiring a thoughtful approach to select the most suitable method for specific research needs.
Step-by-Step Protocol for DNA Isolation
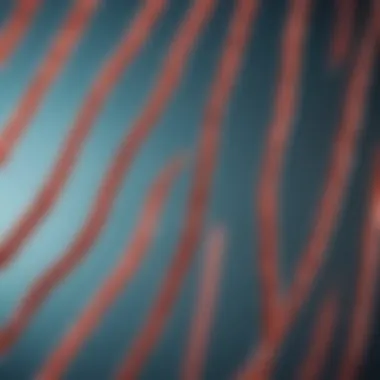
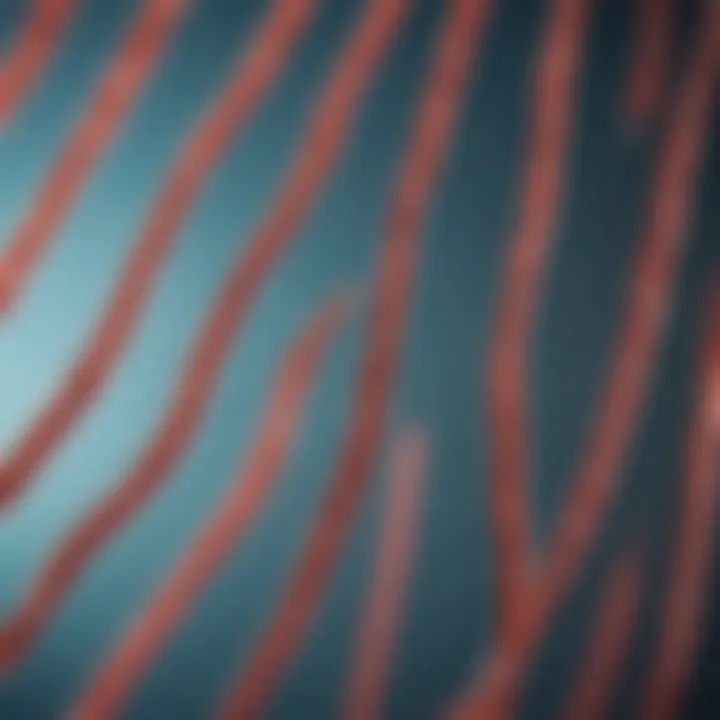
The step-by-step protocol for DNA isolation is central to our understanding of the processes involved. This section lays out the practical application of theory, serving as a scientific roadmap. Through precise and systematic methods, researchers can extract DNA efficiently from various sources while ensuring its integrity. Each phase of this protocol is vital. Neglecting even a minor detail can compromise the quality of isolated DNA, affecting subsequent applications such as cloning or PCR amplification. Therefore, comprehending the nuances of the protocol contributes not only to success in the lab but also advances in biological research.
Sample Preparation
Sample preparation is the first critical step in the DNA isolation protocol. It involves collecting biological material suitable for DNA extraction. The choice of source—be it blood, tissue, or plant—is critical. Proper storage and handling are crucial to prevent degradation of DNA.
- Types of samples:
- Animal sources: Commonly blood, saliva, or tissue.
- Plant sources: Leaves or seeds are often used.
- Microbial sources: Bacterial cultures are a typical source within lab settings.
The success of subsequent steps hinges on the quality of the prepared sample. It is common practice to minimize contamination by using sterile techniques and high-purity materials during this phase. Preparing samples correctly ensures that the extracted DNA is representative of the initial biological material.
Cell Lysis Process
After sample preparation, the next step is the cell lysis process. Lysis involves breaking open the cells to release DNA into solution. This process typically uses lysis buffers containing detergents that disrupt cellular membranes. Furthermore, enzymes might be employed to digest proteins and other cellular components that could contaminate the DNA.
Common reagents include:
- Sodium dodecyl sulfate (SDS): A strong detergent for breaking cell membranes.
- Proteinase K: An enzyme that digests proteins and frees DNA.
- Heating: Often, samples are incubated at elevated temperatures to enhance lysis.
Maintaining temperature and duration during this step is crucial. Inadequate lysis time can result in poor yields while excessive heating may lead to DNA degradation. Thus, careful monitoring ensures maximum recovery of high-quality DNA.
DNA Precipitation and Purification
The final phase in the step-by-step protocol involves DNA precipitation and purification. Once lysis is complete, the solution often contains proteins, lipids, and other cellular debris. Precipitation separates DNA from these contaminants. Alcohols, such as ethanol or isopropanol, facilitate this step.
- Process:
- Add cold alcohol: Typically, 70% ethanol is added to the solution.
- Centrifugation: The sample is spun down to form a pellet containing DNA.
- Wash the pellet: This step removes any remaining impurities.
- Dissolve DNA: Finally, the DNA pellet is resuspended in a buffer or water for storage.
This process drastically increases the purity and integrity of the isolated DNA, making it suitable for various downstream applications. Ultimately, a thorough understanding of these procedures is essential for researchers aiming to optimize the DNA isolation process, paving the way for innovative studies in genetics and molecular biology.
"The quality of isolated DNA can heavily influence experimental outcomes, especially in sensitive applications like NGS (Next Generation Sequencing)."
By adhering to structured protocols, scientists can maintain high standards in their research and ensure valid results.
Challenges in DNA Isolation
DNA isolation is a critical process in molecular biology, however, several challenges can hinder its effectiveness. Understanding these obstacles is essential for achieving high-quality DNA preparations. Each challenge not only impacts the quality and quantity of the isolated DNA but also influences downstream applications such as cloning, sequencing, and PCR. This section discusses the main challenges, emphasizing the need for careful attention to detail in laboratory procedures.
Contamination Issues
Contamination is one of the foremost challenges during DNA isolation. Sources of contamination can range from environmental particles to cross-contamination between samples. Contaminants can degrade DNA or interfere with analytical procedures, leading to unreliable results. Ensuring the integrity of DNA means keeping the workspace sterile, using disposable materials when possible, and regularly decontaminating surfaces and equipment.
To minimize contamination risks, researchers should adopt the following practices:
- Use sterilized tools and reagents, ideally pre-packaged.
- Conduct work in a cleanroom or laminar airflow hood to limit exposure to airborne contaminants.
- Implement proper waste disposal protocols to avoid accidental contamination of other samples.
- Maintain a clear separation between different samples to ensure no cross-sample interference.
Yield and Quality Optimization
Achieving optimal yield and quality of DNA is crucial for the success of various applications. Several factors affect both the yield and the integrity of isolated DNA. These include the type of biological material used, the extraction method employed, and the accuracy of each step within the isolation protocol.
Common strategies to improve yield and quality include:
- Sample Selection: The source of the DNA significantly affects the outcome. For instance, animal tissues often yield more DNA than more challenging plant tissues.
- Extraction Techniques: Different methods suit different types of samples. Silica gel methods might provide higher purity for certain tissues, whereas phenol-chloroform extraction may yield more DNA from others.
- Optimization of Protocols: Adjusting lysis buffer composition, incubation times, and temperatures are critical steps that directly impact both yield and quality. Trial and error may be necessary to find the best conditions for specific samples.
"Optimizing DNA yield is both an art and a science; understanding the nuances of each sample type can lead to substantial improvements in results."
Awareness of these challenges gives researchers the foundation needed to develop effective protocols for DNA isolation. By addressing contamination and optimizing yield, they can significantly impact the outcomes of their research.
Applications of Isolated DNA
Isolated DNA plays a pivotal role across various scientific and practical fields. Given its unique properties, DNA extracted from different organisms has vast implications in research and industry. Understanding the applications of DNA isolation is vital for students, researchers, educators, and professionals in molecular biology, genetics, and biotechnology.
Among the main applications are genetic engineering, forensic analysis, and medical diagnostics. Each of these areas leverages the properties of DNA to address specific problems or enhance existing processes and technologies.
Genetic Engineering
Genetic engineering involves manipulating the genetic material of organisms to achieve desired traits or produce specific biological products. The isolated DNA serves as the foundation for these modifications. By utilizing techniques such as recombinant DNA technology, scientists can insert, delete, or alter genes in an organism’s genome.
The benefits of genetic engineering are extensive. For example, it allows for the development of genetically modified organisms (GMOs) which can enhance agricultural efficiency and produce crops resistant to pests. Moreover, genetically engineered microbes can produce insulin and other critical pharmaceuticals, streamlining production methods and reducing costs.
Forensic Analysis
In forensic science, isolated DNA is crucial for identifying individuals in criminal investigations. DNA profiling can provide solid evidence linking a suspect to a crime scene or exonerating the innocent. The process typically involves collecting samples from the scene, isolating DNA, and then performing analyses to compare genetic markers.
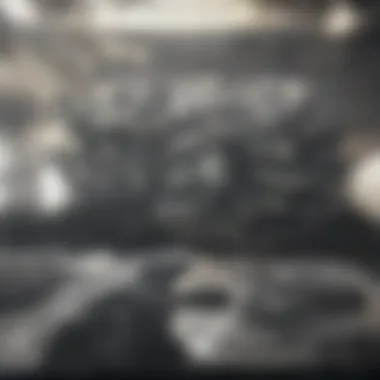
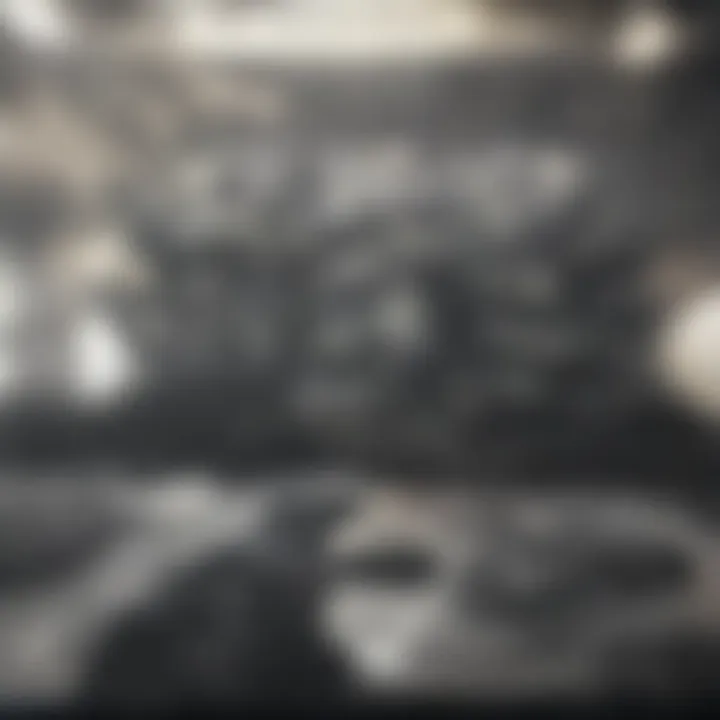
Furthermore, the precision of DNA analysis has significantly advanced investigative procedures. This field employs techniques like short tandem repeat (STR) analysis and mitochondrial DNA sequencing to improve accuracy in identification. These advancements fostered higher confidence in forensic results, leading to improved judicial outcomes.
Medical Diagnostics
In medical diagnostics, isolated DNA is integral to various testing methods, including genetic screening and disease identification. Techniques such as polymerase chain reaction (PCR) allow clinicians to detect genetic disorders and infectious diseases by amplifying specific DNA segments.
The rapid evolution of DNA diagnostics provides numerous benefits, including early detection of diseases, personalized treatment plans, and improved patient outcomes. It has also opened pathways for novel therapies and vaccines, especially in response to emerging infectious diseases and genetic disorders.
"DNA isolation is the foundation upon which advancements in genetic research, forensic science, and medical diagnosis are built. Without it, many modern techniques would not be possible."
Safety and Ethical Considerations
Safety and ethical aspects are pivotal in the context of DNA isolation. As research in molecular biology expands, it becomes essential to ensure laboratory environments are safe and that research practices maintain ethical standards. This safeguard protects not only the integrity of scientific work but also the well-being of researchers and the public.
Laboratory Safety Protocols
Adhering to laboratory safety protocols is crucial in any scientific investigation involving DNA isolation. Various chemicals used in the process, such as phenol and chloroform, can be toxic if handled improperly.
It is essential to:
- Utilize personal protective equipment (PPE), including gloves, lab coats, and eye protection.
- Implement proper waste disposal protocols to manage hazardous materials responsibly.
- Ensure lab spaces are well-ventilated to mitigate the risk of inhalation.
- Regularly train personnel on safety procedures and emergency protocols. This ongoing education fosters a culture of safety within the laboratory environment.
By maintaining rigorous safety standards, laboratories not only protect their staff but also enhance the reliability and reproducibility of their research findings.
Ethics in Genetic Research
With advancements in DNA isolation and manipulation come significant ethical considerations. Research involving human genetic material raises questions about consent, privacy, and the potential for misuse. Ethical guidelines must comply with institutional, national, and international standards to ensure responsible usage of genetic data.
Key ethical concerns include:
- Informed Consent: Researchers must obtain explicit consent from participants who provide genetic samples. This consent should detail how samples will be used, stored, and potentially shared in the future.
- Privacy Protections: Safeguarding personal genetic data is paramount as breaches can lead to discrimination or stigmatization. Implementing stringent data protection protocols mitigates these risks.
- Responsible Use: The potential for genetic engineering and modification necessitates a thorough examination of the implications and consequences of such research. Researchers must consider the long-term effects of their work on individuals and society.
Ethical considerations in genetic research are more than regulatory requirements; they are fundamental to the advancement of knowledge in a respectful and responsible manner.
Overall, prioritizing safety and ethical considerations in DNA isolation practices fosters a secure and respectful research environment, ensuring that advancements in genetic science benefit society as a whole.
Future Directions in DNA Isolation Technologies
The field of DNA isolation is continuously evolving. As molecular biology advances, innovative approaches are being developed to enhance DNA extraction processes. Understanding future directions in DNA isolation technologies is crucial for researchers and practitioners. These advancements can potentially lead to improved efficiency, accuracy, and applicability across various fields such as medicine and genetics. The next few sections will outline emerging techniques and the role of automation in these processes.
Innovative Techniques Under Development
New techniques are emerging in DNA isolation that promise to increase both the yield and quality of extracted DNA. One such method is the use of magnetic beads, which offer distinct advantages over traditional extraction techniques. Magnetic beads bind to DNA, allowing for easy separation from other cellular components.
Another promising area of development is microfluidic technology. This method utilizes tiny channels to manipulate fluids at a very small scale. This can lead to highly efficient DNA isolation, requiring less sample and reagents than conventional methods. Efforts are also being made to enhance the specificity of DNA extraction.
Some notable techniques include:
- CRISPR-based extraction methods that use gene-editing tools for more precise isolation.
- Nanopore sequencing technologies that may enable real-time monitoring of DNA isolation processes.
These techniques not only improve the overall extraction process but also expand the potential applications in clinical diagnostics and personalized medicine.
Impact of Automation and Robotics
Automation in DNA isolation is transforming laboratory practices. It minimizes the risk of human error and increases throughput, allowing more samples to be processed efficiently.
Robotic systems can perform repetitive tasks with high precision, making them ideal for large-scale studies where consistency is key. The integration of software to control robotic equipment can also facilitate complex workflows, allowing researchers to focus on data analysis rather than the manual labor of DNA extraction.
Key benefits of automation in DNA isolation include:
- Increased reproducibility by eliminating variability in sample processing.
- Faster turnaround times for results, which is crucial in areas such as forensic analysis and clinical diagnostics.
- Reduced labor costs, freeing up personnel for other important tasks in the laboratory.
"The shift towards robotics in extracting DNA is not just about speed; it represents a paradigm shift towards efficiency and accuracy in research workflows."
Emphasizing these future technologies not only gives researchers the tools they need but also aligns with broader trends in biotechnology focusing on precision and efficiency.
The End
The conclusion of this article encapsulates the essence of DNA isolation, revealing its multifaceted importance in the field of molecular biology. This final section is vital as it not only recaps the main insights presented but also provides a comprehensive overview of the relevance and potential future of DNA isolation techniques.
Recapitulation of Key Points
Throughout the article, we examined various crucial aspects, including:
- Techniques and Procedures: Several methods such as phenol-chloroform extraction, silica gel membrane methods, and column purification were highlighted. Each technique demonstrates the intricacies involved in isolating DNA, reflecting both historical context and modern advancements.
- Sources of DNA: We discussed a variety of DNA sources, emphasizing plant, animal, and microbial origins. Understanding these sources aids in selecting appropriate isolation methods.
- Applications: The applications of isolated DNA in genetic engineering, forensic analysis, and medical diagnostics were explored, showcasing the profound implications of isolation on science and society.
These points illustrate that DNA isolation is not merely a laboratory procedure; it serves as a foundational element that enables a myriad of scientific discoveries and applications.
Significance of Continued Research
Continuing research in DNA isolation is paramount. As technology evolves, the efficiency and effectiveness of isolation methods can significantly improve. Benefits of ongoing research include:
- Enhanced Techniques: Innovative approaches, such as automation and robotics, promise to streamline protocols and reduce contamination risks.
- Increased Accessibility: As methods become more efficient, DNA isolation will be more accessible to smaller labs and educational institutions, fostering broader scientific inquiry.
- Ethical Considerations: Continuous research allows for a better understanding of ethical implications in genetic research, guiding policies and practice.