Karyotype Analysis: Exploring Genetic Structures
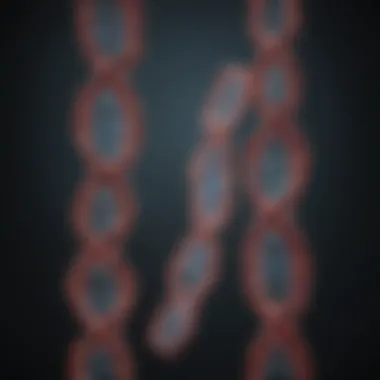
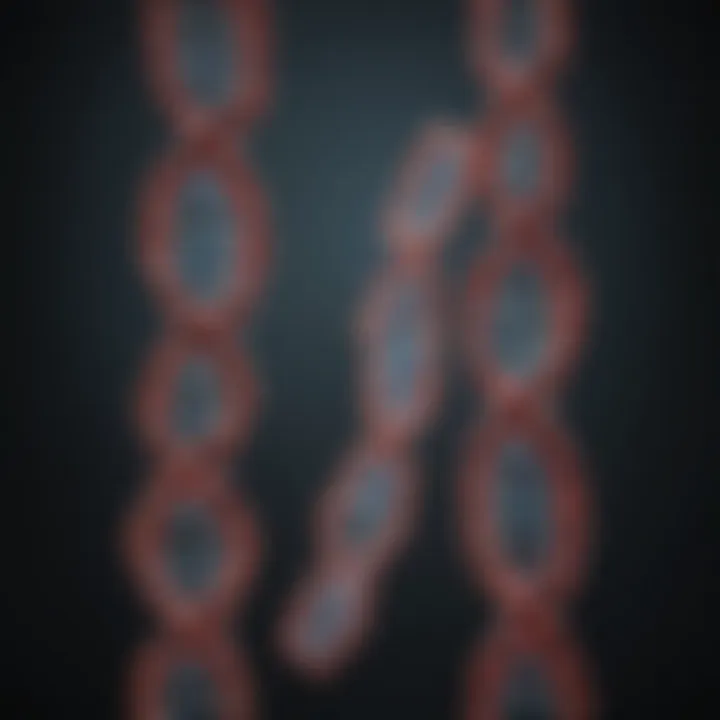
Intro
Karyotype analysis is a vital process in genetics that allows for the visual representation of chromosomal structures. This method is essential for understanding genetic makeup and the implications of chromosomal abnormalities. Through karyotyping, researchers can diagnose genetic disorders, study evolutionary relationships, and unravel the underlying causes of various health conditions. The significance of karyotype analysis extends beyond mere visualization; it plays a crucial role in informing clinical decisions and advancing genetic research.
In the following sections, we will delve into recent advances in karyotype analysis, explore the methodologies employed in this field, and address the ethical considerations that accompany genetic research. This comprehensive guide aims to equip students, educators, and professionals with a nuanced understanding of karyotyping and its impact on genetics and healthcare.
Recent Advances
Latest Discoveries
Recent research in karyotype analysis has unveiled critical insights into chromosomal structure and function. Studies have revealed that specific chromosomal abnormalities correlate with increased risks for various genetic disorders. For instance, advancements in high-resolution karyotyping techniques have allowed scientists to detect microdeletions and duplications that were previously undetectable. These findings enhance the ability to diagnose conditions like Turner Syndrome and Down Syndrome at an earlier stage.
Another significant discovery involves the relationship between chromosomal arrangements and cancer. Researchers have identified that certain chromosomal translocations are consistently associated with specific types of cancers. Understanding these connections could lead to better-targeted therapies.
Technological Innovations
Technological advancements have significantly enhanced karyotype analysis. Developments in software and imaging tools allow for more precise chromosomal identification. Software programs can now automate the detection and analysis of chromosomal abnormalities, improving both the efficiency and accuracy of karyotyping.
The application of next-generation sequencing techniques has further revolutionized the field. Whole genome sequencing can now complement traditional karyotyping, providing a more extensive view of the genetic landscape. This integration offers a broader understanding of genetic variations, enabling researchers to draw more comprehensive conclusions regarding chromosomal health.
"With the integration of advanced technologies, karyotype analysis is reaching new heights in accuracy and scope. These tools empower researchers to uncover the complexities of genetic structures and their implications for health and disease."
Methodology
Research Design
Karyotype analysis typically follows a structured research design that includes preparatory stages, such as sample collection, cell culture, and chromosome visualization. Cell samples may be obtained from blood, bone marrow, or amniotic fluid, depending on the study objectives. After cultivating the cells, they are treated with a substance that halts cell division, allowing for the analysis of chromosomes during the metaphase stage.
Data Collection Techniques
The data collection in karyotype analysis involves several key techniques. These include:
- Chromosome staining: Techniques like G-banding and FISH (Fluorescence In Situ Hybridization) are utilized to visualize chromosomes. These methods enhance contrast, making it easier to identify abnormalities.
- Image analysis: Software tools analyze captured images of karyotypes, facilitating the identification of chromosomal patterns and anomalies.
- Statistical analysis: Researchers employ statistical methods to evaluate the significance of the findings, correlating specific chromosomal changes with genetic disorders.
Prelims to Karyotype Analysis
Karyotype analysis is critical to understanding the genetic framework of both humans and other organisms. It involves the examination of chromosomal structures and can provide valuable insights into genetic abnormalities, evolutionary processes, and various biological principles. In a world where genetic disorders and chromosomal aberrations can have profound impacts on health and development, karyotype analysis serves as a vital tool for researchers, healthcare professionals, and educators.
The topic of karyotype analysis encompasses several components, including the definition of what a karyotype is, its historical significance in genetics, and its contemporary applications. Understanding these elements not only helps in recognizing the role of karyotyping in diagnosing chromosomal disorders but also in exploring its potential in evolutionary studies and personalized medicine. Such knowledge is essential for students and professionals engaged in genetics, biology, and medicine.
It is noteworthy that the benefits of karyotype analysis extend beyond mere diagnostics. This analytical approach allows for a comprehensive view of an organism's genetic material, revealing not only the number and structure of chromosomes but also their functional implications. Consequently, most studies leverage karyotypes to elucidate complex genetic architectures and derive insights into both health and disease.
Definition of Karyotype
A karyotype refers to the complete set of chromosomes within a cell, arranged and classified by size, shape, and number. Typically, a normal human karyotype consists of 46 chromosomes, which are organized into 23 pairs, including one pair of sex chromosomes. Karyotype analysis enables the characterization of these chromosome arrangements and assists in identifying deviations from standard chromosome counts, a situation often linked to various genetic disorders.
The implications of karyotype variations are broad. They can play a role in developmental biology, oncology, and reproductive health. Understanding the normal karyotype provides a baseline against which any anomalies can be measured, aiding in clinical diagnoses and guiding therapeutic decisions when necessary.
Historical Context
The study of karyotypes began in the early 20th century, coinciding with advancements in microscopy and cellular biology. The first successful identification of human chromosomes occurred in 1956, when researchers identified the number of chromosomes in human cells. At this time, the association between certain chromosomal abnormalities and genetic disorders began to emerge, paving the way for the vital practice of karyotype analysis.
As the field of genetics progressed, so too did the methods and technologies used in karyotyping. Techniques such as G-banding made it possible to visualize chromosomes in greater detail, allowing scientists to identify specific chromosomal abnormalities much more accurately. This clarity helped make karyotyping a standard practice in diagnosing conditions like Down syndrome and Turner syndrome.
Throughout the years, karyotype analysis has evolved, responding to advances in both technology and our understanding of genetics. It remains a cornerstone of genetic research and medical genetics, affecting how we understand chromosomal behavior across species and its implications for health and disease.
Significance of Karyotyping in Genetics
Karyotyping plays a crucial role in the field of genetics. It is a systematic way of studying the chromosome structure and number, which sheds light on various genetic conditions and species diversity. Understanding the significance of karyotyping helps in diagnosing diseases, guiding treatment options, and advancing research. The methods used in karyotyping also allow scientists to explore phylogenetic relationships among organisms, providing insights into the evolutionary trajectories of species.
Chromosomal Disorders
Chromosomal disorders arise from abnormalities in the number or structure of chromosomes. These abnormalities can lead to various medical conditions, often severe. For example, Down syndrome occurs due to an extra copy of chromosome 21. Understanding these disorders is critical for both diagnosis and treatment planning.
Karyotyping helps identify these disorders by visually inspecting chromosomes from a sample. Changes in chromosomes such as deletions, duplications, and translocations can be detected easily. Karyotype analysis can confirm a suspicion based on physical features or family history, thus guiding further medical evaluations. This diagnostic capability is invaluable for genetic counseling, helping expecting parents make informed decisions.
Prenatal Diagnosis
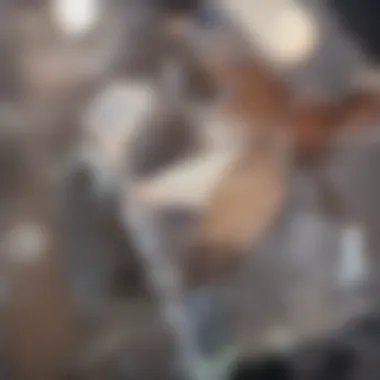
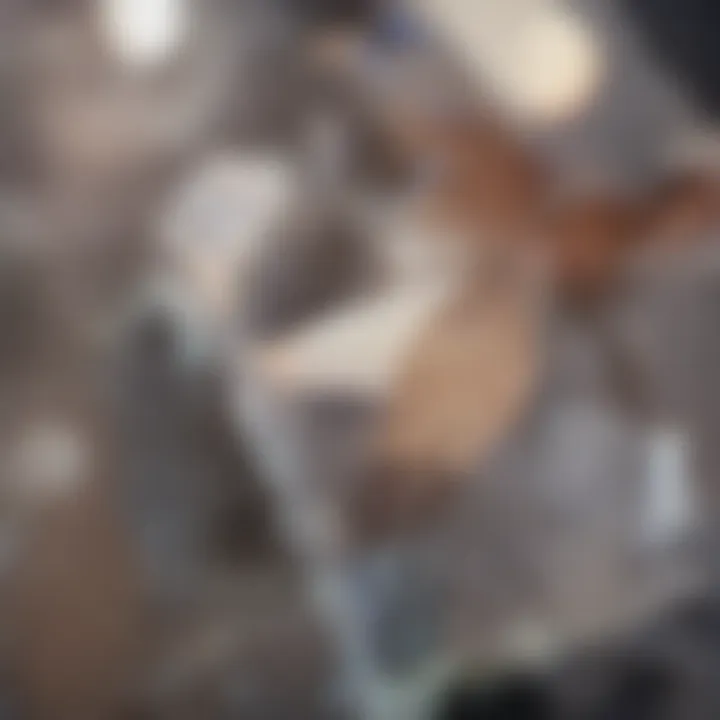
Karyotyping is especially significant in the realm of prenatal diagnosis. The ability to analyze fetal chromosomes helps detect potential genetic issues before birth. Various methods such as amniocentesis and chorionic villus sampling can provide samples for karyotyping.
Through these tests, parents can learn about genetic conditions such as Turner syndrome or Klinefelter syndrome, among others. The implications are profound, as knowing about these conditions early can lead to more prepared parenting, changes in prenatal care, or considerations regarding termination.
The significance of karyotyping in genetics extends beyond clinical applications. It also informs societal decisions, ethical considerations, and personal choices regarding family planning.
Karyotype Analysis Process
The karyotype analysis process is a critical stepping stone in understanding the chromosomal architecture of an organism. One might consider it as the bridge that connects the intricacies of genetic material with observable characteristics. This process encompasses several stages, each of which plays a vital role in ensuring accurate results. Various techniques involved contribute significantly to the reliability of findings in genetic research and diagnosis of disorders.
Sample Collection Techniques
Collecting samples is the very first step in karyotype analysis. The quality of the sample can greatly influence the success of the subsequent analysis. Common sources for sample collection include blood, bone marrow, or amniotic fluid. In humans, peripheral blood is often the preferred source because it is easier to obtain and yields a higher number of cells compared to other sources.
Key considerations during sample collection include:
- Ensuring sterility to prevent contamination.
- Using appropriate anticoagulants to preserve the sample.
- Collecting enough volume to perform subsequent tests.
In cases of prenatal diagnosis, amniocentesis or chorionic villus sampling may be used. These methods require careful handling and are often performed under ultrasound guidance to minimize risk to the fetus. The efficiency of these techniques directly impacts the accuracy of the karyotyping process.
Cell Culture Methods
Once samples are collected, the next crucial step is cell culture. This process involves growing the collected cells under controlled laboratory conditions, which allows for the division and proliferation of cells. The primary goal is to obtain a sufficient number of cells for analysis.
There are a few different methods of cell culture employed in karyotype analysis:
- Standard cell culture: Involves placing cells in a nutrient-rich medium that supports growth.
- Suspension culture: Useful for liquid samples, where cells are cultured in suspension rather than adhering to a surface.
- Microbial culture: Sometimes relevant for species other than humans, where organisms are cultured to analyze their genetic structure.
Maintaining optimal conditions, such as temperature and gas concentrations, is crucial for successful culturing. Failure to do so may lead to compromised cell integrity, thus affecting the outcome of the karyotype analysis.
Staining Techniques
Finally, staining techniques are pivotal in visualizing chromosomes. Without proper staining, observing and differentiating the chromosomal structures would be nearly impossible. The most commonly used stains in karyotype analysis are Giemsa stain, which provides a distinct banding pattern on chromosomes.
Common staining methods include:
- G-banding: Produces light and dark striations that help identify individual chromosomes based on their characteristic banding patterns.
- Q-banding: Utilizes a different fluorescent dye, enhancing resolution in specific areas of the chromosomes.
- C-banding: This method stains only the centromeric regions, useful for identifying certain chromosomal abnormalities.
These techniques enable researchers to visually compare chromosomes, aiding in identifying structural abnormalities. Accurate staining is critical for the success of karyotype analysis, as it directly affects the ability to interpret results.
"Karyotype analysis process is a meticulous journey, from sample collection through cell culture, to chromosome identification through staining techniques. Each step requires attention to detail and precise execution for accurate outcomes."
Interpreting Karyotype Results
Interpreting karyotype results is crucial in the field of genetics. This section elucidates how scientists derive meaning from karyotypic data. A proper interpretation can lead to significant insights about an organism's health, genetic conditions, and even its evolutionary history.
Identifying Chromosomes
When analyzing karyotypes, the first step is identifying individual chromosomes. Each chromosome has a distinct size, shape, and banding pattern. These characteristics are important for classification. The process typically involves:
- Visualizing Chromosomes: Scientists use microscopy to examine stained cells. Chromosomes are photographed and arranged in a standard format known as a karyogram.
- Classification: Chromosomes are classified according to their position of the centromere, which divides them into short and long arms. Understanding these features is essential for identifying abnormalities.
- Pairing Homologous Chromosomes: Once visualized, chromosomes are paired with their homologs. This helps clarify whether the organism has the correct number of chromosomes, which is particularly important in diagnosing conditions like Down syndrome.
Identifying chromosomes sets the foundation for deeper genetic understanding. It enables researchers to compare the karyotypes of different individuals or species, contributing to the field of comparative genomics.
Detecting Abnormalities
The second key aspect in interpreting karyotype results is detecting abnormalities. Chromosomal abnormalities can manifest in several ways. They may include extra copies of chromosomes, missing chromosomes, or structural changes in existing chromosomes.
Critical points in this process include:
- Numerical Abnormalities: These involve changes in the number of chromosomes. For example, Trisomy 21, which is Down syndrome, results from an extra copy of chromosome 21.
- Structural Abnormalities: These occur when chromosomes break and rearrange. Examples include deletions (missing chromosome segments), duplications (extra segments), inversions (reversed segments), and translocations (segments swapped between nonhomologous chromosomes).
- Clinical Implications: Abnormalities can have significant health implications. Accurate detection helps medical professionals diagnose genetic disorders, offering paths for treatments or interventions.
Accurate identification of chromosomal abnormalities is not just academic. It can influence patient management and inform family planning decisions.
Interpreting karyotype results requires skill and attention to detail. The ability to identify chromosomes accurately and detect their abnormalities plays a key role in understanding genetic disorders. This process ultimately allows for early diagnosis and better patient outcomes in clinical settings.
Applications in Various Organisms
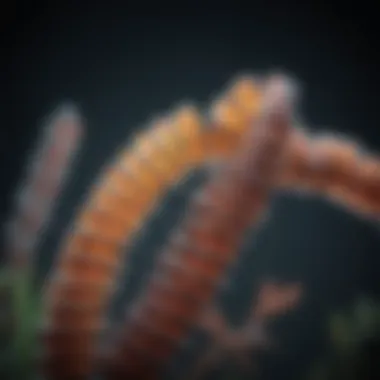
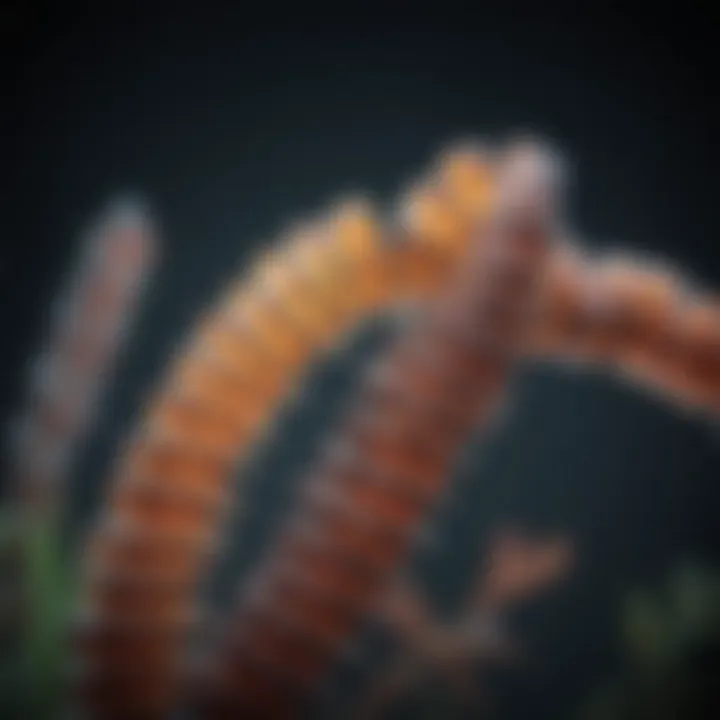
Karyotype analysis is not limited to human genetics; it has profound implications in a wide array of organisms. Understanding chromosomal structures across diverse species aids in various fields, including agriculture, conservation, and medicine. This section will outline the significant applications of karyotype analysis in humans, plants, and animals, stressing its multifaceted importance.
Human Karyotyping
Human karyotyping involves examining the chromosomal makeup of individuals. It is crucial in identifying genetic disorders, which may arise from chromosomal abnormalities such as Down syndrome, Turner syndrome, or Klinefelter syndrome. By analyzing karyotypes, scientists can detect aneuploidies, deletions, and duplications that might not be apparent through other diagnostic methods.
Karyotyping can be especially valuable during prenatal diagnostics. For instance, non-invasive prenatal testing uses fetal DNA to identify any potential genetic issues. Identifying these abnormalities early in pregnancy can guide expectant parents and healthcare providers in decision-making and potential interventions.
Additionally, human karyotyping aids in cancer research. Tumor cells often exhibit distinct karyotypic changes that differ from normal cells. Understanding these variations helps researchers develop targeted therapies and better understand the mechanisms of cancer development.
Plant Karyotyping
Karyotype analysis in plants provides insights into their genetic diversity, evolution, and adaptability. The chromosomal structure of plants can greatly affect their reproductive strategies, growth, and responses to environmental pressures. Through karyotype studies, scientists can classify plant species, understand their phylogenetic relationships, and track evolutionary changes over time.
In agriculture, plant karyotyping is particularly useful for crop improvement. By identifying genetic traits associated with yield, disease resistance, or stress tolerance, breeders can select parents for hybridization. This method enhances the efficiency of plant breeding programs, directly impacting food security and agricultural sustainability.
Moreover, examining karyotypic variations among related species can illuminate pathways for speciation. Researchers can observe how chromosomal rearrangements influence reproductive isolation and adaptation to specific habitats.
Animal Karyotyping
Animal karyotyping plays a vital role in genetic research and biodiversity conservation. Analyzing the chromosomes of different animal species allows researchers to study genetic variations and understand evolutionary relationships. Karyotypes help in constructing phylogenetic trees, thereby illustrating how species have diverged over time.
In wildlife conservation, karyotyping can assist in identifying populations at risk of inbreeding. By analyzing genetic diversity within a species, conservationists can establish management strategies that support the survival of endangered species. Additionally, karyotyping contributes to understanding how certain traits are inherited and expressed within animal populations.
Overall, karyotype analysis in animals contributes significantly to veterinary medicine. Understanding genetic disorders specific to certain breeds enables veterinarians to diagnose and treat conditions effectively.
Karyotype analysis serves as a bridge connecting genetics, evolution, and practical applications across organisms.
Each of these areas highlights the extensive relevance of karyotyping analysis beyond humans, offering vital tools for research and applications in diverse fields of study.
Karyotype Analysis in Evolutionary Biology
Karyotype analysis plays a critical role in evolutionary biology by providing insights into the genetic structures of various organisms. The data derived from karyotype studies can offer valuable information regarding evolutionary relationships among species. Understanding these relationships is important for elucidating the mechanisms of evolution, speciation, and genetic diversity. This section will discuss the significance of karyotyping in deciphering phylogenetic relationships and the comparative studies that help to interpret evolutionary patterns.
Phylogenetic Relationships
Phylogenetic relationships illustrate how different species are related through common ancestry. Karyotype analysis helps in constructing phylogenetic trees by examining chromosomal similarities and differences. Chromosomes are the carriers of genetic information. By analyzing the structure and number of chromosomes in various species, researchers can identify evolutionary links.
For example, the karyotypic comparison between closely related species can reveal chromosomal fusions or fissions, which are indicators of evolutionary changes. Such changes might suggest adaptations to specific environments or ways organisms cope with genetic mutations. The resulting karyotype data assists in establishing a framework for understanding the genetic lineage and ancestry of species, enabling scientists to predict genetic characteristics in descendants as well.
Comparative Karyotype Studies
Comparative karyotype studies extend beyond just analyzing one species. By examining the karyotypes of several species, researchers can identify evolutionary trends and changes over time. This method allows for a broader understanding of genetic evolution within groups.
In these studies, researchers look for specific chromosomal variations. These variations can include:
- Chromosome number: Some organisms may have more or fewer chromosomes, which often reflects their adaptability and evolutionary history.
- Chromosomal structure: Changes in the physical structure of chromosomes can signify evolutionary adaptations.
- Banding patterns: Different banding patterns can be indicative of certain evolutionary pressures or environmental adaptations.
These findings provide a picture of how species have evolved, adapted, or diverged from a common ancestor. By understanding these comparatives, scientists can make hypotheses regarding the genomic adjustments that occurred through evolutionary time.
Understanding karyotype variations provides crucial insights into the evolutionary processes that shape life on Earth.
Technological Advancements in Karyotype Analysis
Technological advancements have undeniably transformed the field of karyotype analysis. These innovations not only enhance the efficiency and accuracy of analyzing chromosomal structures but also open new avenues for research and clinical applications. As geneticists aim for high precision in diagnosing chromosomal abnormalities and understanding genetic diseases, these advancements become central to both the methods and outcomes of karyotyping.
Modern techniques are essential in accommodating the increasing need for rapid and precise karyotyping. The adoption of advanced technologies allows laboratories to process large numbers of samples simultaneously, which is crucial in clinical settings where timely results can affect diagnosis and treatment plans. With the advancements, researchers can now utilize sophisticated imaging and data analysis algorithms that minimize errors previously common in manual assessments.
High-Throughput Karyotyping
High-throughput karyotyping is one of the most revolutionary items in this domain. This method allows for simultaneous analysis of multiple samples, vastly increasing efficiency. The use of automated systems and robotic technologies reduces the manual labor involved in preparing samples and analyzing chromosomal images. High-throughput techniques have become especially significant in research settings where large datasets are required.
Some benefits include:
- Speed: Rapid processing of samples reduces the time from sample to result.
- Scalability: Laboratories can scale operations to meet demand without a corresponding increase in personnel.
- Data Integration: Facilitates the integration of genomic data, enhancing the depth of analysis.
However, there are considerations. Quality control becomes crucial as the volume of samples increases. The robustness of algorithms used to analyze images is vital to ensure that accuracy is maintained despite the high throughput.
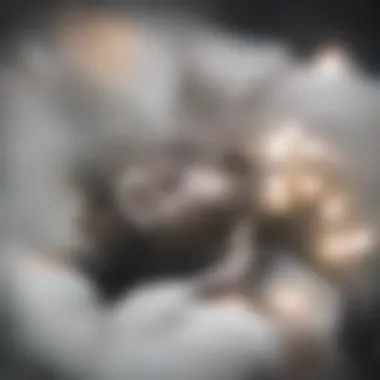
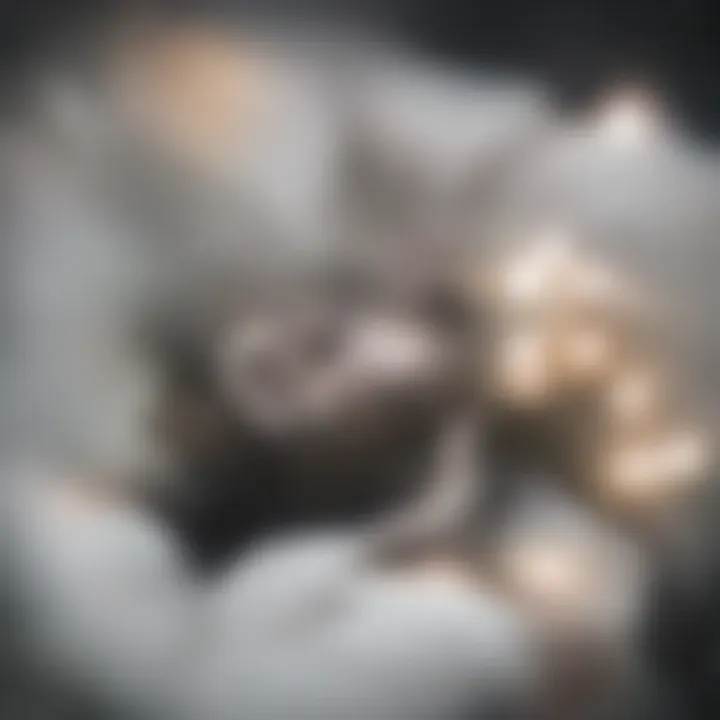
Digital Karyotyping
Digital karyotyping is another advancement that employs imaging technology to provide a detailed analysis of chromosomal structures. This process involves capturing high-resolution images of chromosomes and using software to digitally sort and analyze them. Digital karyotyping allows for a more nuanced examination than traditional methods.
Key elements of digital karyotyping include:
- Enhanced Resolution: Higher image quality reveals finer structural details of chromosomes.
- Automated Analysis: Algorithms facilitate quicker and more accurate identification of chromosomal abnormalities.
- User-Friendly Interfaces: Software is designed to allow easier use by laboratory personnel, reducing training time.
"Technological advancements in karyotype analysis not only improve efficiency but also redefine the scope of genetic research, advancing our understanding of chromosomal abnormalities and their implications in various fields."
The progress in these technologies demonstrates the evolving landscape of karyotyping, making it an essential area for those interested in genetics, whether for educational, clinical, or research purposes.
Ethical Considerations in Genetic Research
Ethical considerations in genetic research are paramount, as they address the moral implications associated with studying and manipulating genetic materials. These concerns are particularly germane in karyotype analysis, a technique that not only reveals the chromosomal structure of organisms but also impacts individual lives through potential diagnoses of genetic disorders. As we progress in our understanding of genetics, it becomes crucial to reflect on the ethical boundaries that govern this field of study.
Privacy Concerns
The issue of privacy looms large in the context of genetic research. Genetic data can reveal sensitive information about an individual, including predispositions to certain diseases and other hereditary traits. This becomes even more consequential in karyotype analysis, where data is often collected from human samples. The potential for misuse of genetic information raises significant questions about consent and data protection.
Individuals should have control over their genetic information, and researchers must ensure that any data collected during karyotype analysis is stored and handled with utmost confidentiality. Regulatory frameworks, such as the Health Insurance Portability and Accountability Act (HIPAA) in the U.S., aim to protect this sensitive data, but enforcement measures vary widely.
The right to privacy must be emphasized, as genetic information is unique and irreplaceable. Failing to protect this could lead to discrimination based on genetic makeup.
Implications of Genetic Testing
Genetic testing, a practice closely tied to karyotype analysis, carries several implications that merit careful consideration. While the ability to identify genetic disorders early can be life-saving and allow for better management of health, it also opens doors to ethical dilemmas. For instance, knowing one's genetic risks may lead to psychological stress or anxiety for individuals and families.
Furthermore, societal implications come into play. There is the risk of stigmatization or discrimination in the workplace based on genetic information, which can affect employment opportunities and insurance coverage. Therefore, it is essential for both researchers and healthcare providers to address these concerns when offering genetic testing services.
Future Perspectives of Karyotype Analysis
Karyotype analysis holds considerable promise for the future of genetic research and application. Its capacity to provide insight into chromosomal structures is evolving alongside technological advancements. Understanding these future perspectives is crucial for educators, researchers, and professionals in genetics, as it can inform the direction of upcoming studies and practical implementations.
Technological innovation plays a key role in enhancing karyotype analysis. As methods improve, the accuracy and speed of karyotyping are likely to increase significantly. Advanced imaging techniques, such as high-resolution microscopy, offer clearer views of chromosomal arrangements. Moreover, automated image analysis can facilitate the efficient processing of samples, which is vital in large-scale studies or clinical settings. These innovations not only improve the reliability of results but also contribute to the overall efficiency of genetic assessments.
- Increased access to genomic information: As karyotype methods become more advanced and widespread, they will likely be integrated into mainstream medical diagnostics. This shift can lead to the rapid identification of chromosomal abnormalities in diverse populations, enhancing prenatal screening and patient care.
- Interdisciplinary approaches: Future analyses will increasingly involve collaboration between genetics, bioinformatics, and computational biology. Integration of data from various fields can offer richer insights into complex genetic structures, helping uncover subtle chromosomal variations that affect health and disease.
"The future of karyotype analysis lies in its blend with technology and interdisciplinary research, potentially transforming our understanding of genetics."
Innovations in Methodologies
Innovations in methodologies are central to the future of karyotype analysis. Advances in genomics, robotics, and software development facilitate new techniques for chromosomal analysis. These methodologies are designed to streamline processes and improve accuracy and depth of analysis.
- Next-Generation Sequencing (NGS): NGS allows for the sequencing of entire genomes which provides a comprehensive view of chromosomal structures. Combining NGS with traditional karyotyping can enhance the ability to detect structural variations.
- Fluorescence In Situ Hybridization (FISH): This method is being refined continuously, allowing for more precise detection of chromosomal abnormalities with minimal sample preparation.
- Whole Genome Amplification (WGA): WGA techniques enable analysis from small or degraded samples, such as those found in prenatal diagnostics or preserved specimens.
Role in Personalized Medicine
Karyotype analysis is poised to play a significant role in personalized medicine. The ability to understand an individual's unique chromosomal makeup can inform tailored treatment strategies. This personalized approach is beneficial in several ways:
- Disease Prevention: By identifying genetic susceptibilities through karyotyping, healthcare providers can recommend preventive measures tailored to individuals.
- Targeted Therapies: Knowledge of chromosomal abnormalities can guide the selection of therapeutic options. For cancers, specific treatment protocols can be implemented based on the karyotype profile of a tumor.
- Informed Decision Making: Patients can make educated decisions about their healthcare based on their chromosomal information. The implications for family planning and understanding heritable conditions are profound.
In summary, the future of karyotype analysis is linked closely to technological advancements and its incorporation into personalized medicine. These developments will likely refine how genetic disorders are diagnosed and treated, propelling the field forward.
Finale
The conclusion of this article is vital in encapsulating the importance and breadth of karyotype analysis within genetics. It synthesizes the primary aspects discussed throughout the sections, illustrating how karyotyping serves as an essential tool. Karyotype analysis not only enables the identification of chromosomal disorders but also plays a crucial role in various fields, such as prenatal diagnosis and comparative studies across species.
Summary of Key Insights
Karyotype analysis highlights several significant insights in genetics:
- The identification of structural and numerical chromosomal abnormalities helps in diagnosing various genetic disorders.
- Techniques like high-throughput and digital karyotyping have transformed the scope of genetic research, enhancing accuracy and efficiency.
- It has vast applications in both human and non-human organisms, presenting a clearer view of genetic variations.
- Karyotyping contributes to our understanding of evolutionary biology, emphasizing phylogenetic relationships among species.
By systematically interpreting the findings, the article provides clarity on these complex concepts, making them accessible to students, researchers, and educators alike.
Implications for Future Research
Future research in karyotype analysis holds many promising avenues:
- Innovations in methodologies will continue to enhance the precision of karyotyping, potentially leading to earlier and more accurate diagnoses of genetic disorders.
- The integration of karyotype analysis in personalized medicine can pave the way for targeted therapy, improving patient outcomes.
- Further comparative karyotype studies across diverse species can unravel evolutionary histories and inform conservation strategies.
In summary, the impact of karyotyping on genetics is profound, shaping both current understanding and future inquiries. This analysis not only proposes immediate applications but also establishes a framework for ongoing exploration in this essential scientific domain.
Continuous advancements in karyotype analysis will greatly influence the landscape of genetic research and clinical practice, promoting a deeper comprehension of human health and biodiversity.