Labeled Antibody with Fluorophore: Insights and Applications
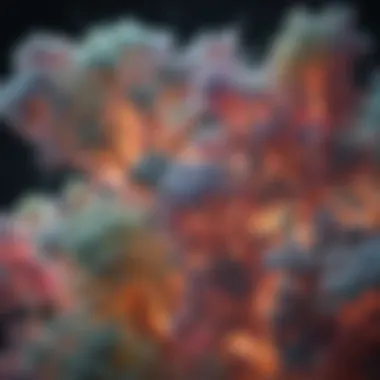
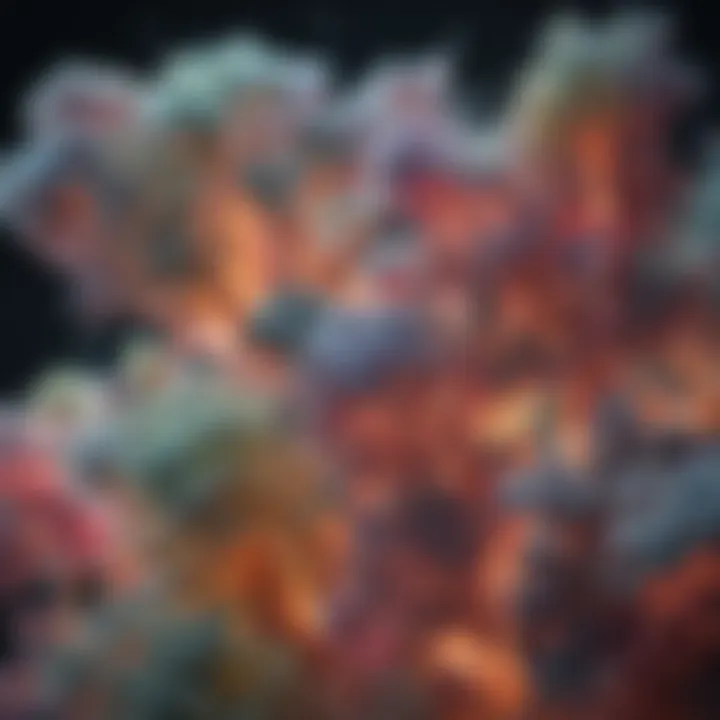
Intro
The intersection of immunology and fluorescence has led to significant improvements in biological research and diagnostics. Labeled antibodies with fluorophores are essential tools that enhance detection methods, offering insights into cellular functions, disease mechanisms, and therapeutic interventions. These advancements not only improve specificity and sensitivity in assays, but also open avenues for novel applications in various scientific disciplines. This article aims to provide a comprehensive exploration of the principles, methodologies, and implications of labeled antibodies with fluorophores.
Recent Advances
Recent innovations in this field focus on the development of more effective fluorophores and advanced antibody labeling techniques.
Latest Discoveries
Research indicates that new fluorophores exhibit improved brightness and stability. For instance, innovative dyes such as Atto532 and Alexa Fluor 647 have emerged, showcasing remarkable properties that enhance detection in complex biological samples. These recent discoveries make it possible to visualize cellular processes more accurately, thus providing a clearer picture of biological phenomena.
Technological Innovations
Furthermore, advancements in imaging technologies, such as super-resolution microscopy, allow scientists to observe structures at unprecedented resolutions. These tools integrate labeled antibodies with fluorophores to reveal details that traditional techniques cannot capture. The synergy between these innovations greatly elevates the potential of fluorescence microscopy in research.
Methodology
In studying labeled antibodies with fluorophores, a clear methodology is crucial. This section outlines key aspects associated with research design and data collection techniques utilized in this sphere.
Research Design
The research typically involves both in vitro and in vivo studies, testing the efficacy of labeled antibodies in diverse applications. Design considerations include the selection of appropriate fluorophores and antibodies tailored to specific targets. This step ensures that the desired signals are detectable against the background of complex biological samples.
Data Collection Techniques
Data collection employs various techniques, such as flow cytometry and confocal microscopy. These tools help quantify and visualize fluorescent signals emitted from labeled antibodies. By using specific controls and calibrations, researchers can validate results and minimize non-specific binding, which is a common challenge in these experiments.
Preface to Labeled Antibodies
Labeled antibodies have become essential tools in biological research, diagnostics, and various medical applications. Understanding their function and significance is crucial for anyone involved in the life sciences. The intricate relationship between antibodies and fluorophores enhances visualization techniques, allowing researchers to detect and analyze specific proteins in complex biological samples. The development of labeled antibodies has fundamentally changed how scientists approach tasks in immunology and cellular biology.
Definition and Importance
Labeled antibodies refer to antibodies that are conjugated to a fluorescent dye or fluorophore. The primary importance of these labeled antibodies lies in their ability to enable the detection of specific antigens using various techniques like immunofluorescence microscopy or flow cytometry. By allowing scientists to visualize the distribution and abundance of proteins within cells or tissues, labeled antibodies facilitate a wealth of discoveries in cellular functions, disease mechanisms, and therapeutic targets.
For instance, these antibodies can help in identifying disease markers, thereby aiding early diagnosis and treatment strategies. The specificity of labeled antibodies also minimizes background noise, increasing the accuracy of results in assays. In summary, the utilization of labeled antibodies integrated with fluorophores is significant because it provides precise insights into biological processes, improving our understanding of health and disease.
Historical Context
The journey of labeled antibodies began in the mid-20th century with the advent of fluorescence microscopy. Early proponents of this technology sought to visualize cell components without the hindrance of traditional staining methods that were often non-specific. The first labeled antibodies were created by conjugating enzymes to antibodies; however, these lacked the sensitivity and clarity required for detailed analysis.
In the 1970s, with the development of fluorophores such as fluorescein isothiocyanate, researchers found a more effective way to attach labels to antibodies. This leap in technology permitted the more reliable detection of proteins at a cellular level. Over the years, the quality and variety of fluorophores have expanded significantly, leading to the emergence of multiple labeling strategies. Such advancements have resulted in complex applications across various fields, from basic research to clinical diagnostics, marking a drastic change in how molecular biology is studied and understood.
Understanding Fluorophores
Fluorophores are crucial components in the realm of labeled antibodies. Their significance cannot be understated as they contribute to various research and diagnostic applications. Within the framework of this article, understanding fluorophores allows for deeper insights into how they enhance the visualization of biological processes. Their unique properties enable researchers to track and study specific targets in cells or tissues more efficiently. This understanding not only aids in experimental design but also supports the interpretations drawn from fluorescence-based assays.
Chemical Properties of Fluorophores
The chemical properties of fluorophores dictate their performance in various applications. Key characteristics include the ability to absorb light at specific wavelengths and subsequently emit light at different wavelengths. The stability of these compounds under various conditions, such as pH changes or the presence of other chemicals, is vital.
Furthermore, the quantum yield, which is a measure of how efficiently a substance converts absorbed light into emitted light, is also critical. Higher quantum yields generally translate to better brightness in imaging applications. Understanding these properties will equip researchers with the knowledge necessary to select appropriate fluorophores for their studies.
Types of Fluorophores
In identifying suitable fluorophores, different types can be considered based on their unique attributes and applications. The main types of fluorophores include organic fluorophores, quantum dots, and proteins that function as fluorophores. Each type contributes distinct advantages and may be selected based on specific research needs.
Organic Fluorophores
Organic fluorophores are widely used due to their ease of synthetic accessibility and versatility. They usually exhibit bright fluorescence and are favorable for various biomedical applications. A key characteristic of organic fluorophores is their strong interaction with different solvents, which facilitates diverse labeling techniques.
However, they can also have significant drawbacks. For example, some organic fluorophores suffer from photobleaching, where their fluorescence diminishes over time when exposed to light. Therefore, while they are a popular choice in many studies, their limitations must be carefully managed in experimental designs.
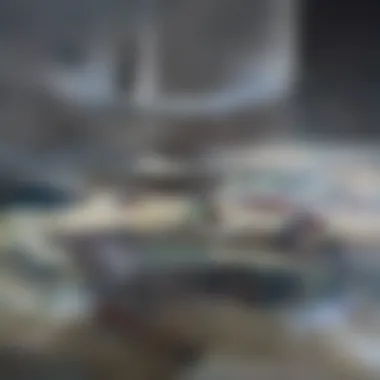
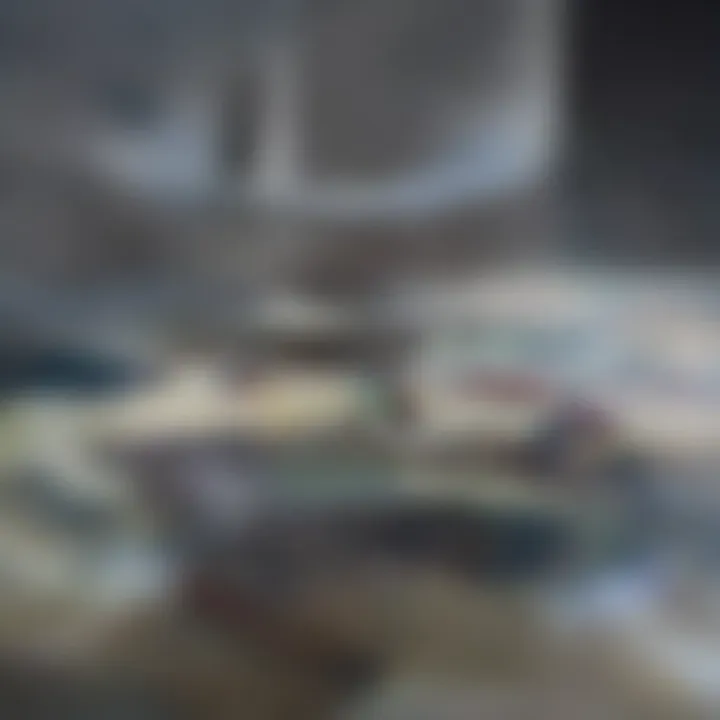
Quantum Dots
Quantum dots represent a newer class of fluorophores characterized by their semiconductor properties. These offer unique advantages such as size-tunable emission wavelengths and superior photostability compared to organic compounds. Quantum dots maintain their fluorescence longer, making them attractive for long-term imaging studies.
Nevertheless, the synthesis of quantum dots is often more complex and expensive. Additionally, concerns regarding their biocompatibility can limit their use in biological systems. Their properties require careful consideration when planning experimental protocols.
Proteins as Fluorophores
Proteins that are intrinsically fluorescent have gained prominence as viable fluorophores. They provide specific advantages such as being naturally occurring within biological systems, minimizing issues related to biocompatibility. A key characteristic is their ability to be genetically engineered to enhance or modify their fluorescent properties.
On the downside, the brightness of protein-based fluorophores might not match that of synthetic alternatives. They also may vary in expression levels, introducing variability in experimental outcomes. Nonetheless, their unique features make them an essential consideration in the context of labeled antibodies.
Understanding the diverse types of fluorophores enhances the selection process for labeling antibodies, ultimately leading to improved experimental outcomes in research.
Mechanisms of Antibody Labeling
Understanding the mechanisms of antibody labeling is essential in the field of immunology and fluorescence microscopy. Labeled antibodies facilitate the visualization of specific proteins and cellular structures. This section emphasizes the different methods employed to attach fluorophores to antibodies, delving into the implications each technique presents.
Direct Labeling Techniques
Direct labeling involves the covalent attachment of fluorophores to antibodies. This method ensures that the fluorescent tag is part of the antibody's structure. The benefits of direct labeling include simplicity and the ability to produce consistent results. Employing this technique, researchers can create labeled antibodies efficiently, which are ready for use in experiments.
However, there are limitations. Direct labeling can alter the antibody's binding affinity, potentially affecting its functionality. It is critical to select an appropriate fluorophore to minimize interference. Designing experiments requires in-depth knowledge of the labeling chemistry.
When using direct labeling, important considerations include:
- Fluorophore choice: Select a fluorophore with suitable spectral properties.
- Antibody concentration: Adequate concentration is necessary to ensure effective labeling.
- Reaction conditions: Optimize pH and temperature to enhance the labeling process.
Indirect Labeling Techniques
Indirect labeling involves the use of secondary antibodies that have been labeled with fluorophores. This strategy first requires binding a primary antibody to the target antigen, followed by the application of a secondary antibody that recognizes the primary. This process amplifies the fluorescent signal, which can be beneficial in experiments with low abundance targets. The enhanced sensitivity of indirect labeling makes it a popular choice.
Advantages of indirect labeling include:
- Signal amplification: Secondary antibodies can bind multiple primary antibodies, increasing signal strength.
- Flexibility in fluorophore selection: Various secondary antibodies can be labeled with different fluorophores, allowing for multiplexing.
- Reduced risk of altering primary antibody function: The primary antibody is less likely to be impacted by the labeling process.
Nevertheless, indirect labeling has challenges, such as the potential for additional background noise due to non-specific binding of the secondary antibody. Rigorous validation is essential to ensure specific detection in complex samples.
Comparison of Techniques
While both direct and indirect techniques serve the purpose of fluorescent labeling, a detailed comparison reveals distinct advantages and drawbacks.
- Direct Labeling:
- Indirect Labeling:
- Simplicity and speed.
- Potential alteration of antibody functionality.
- Higher sensitivity due to signal amplification.
- Greater flexibility with fluorophore selection.
When to choose one over the other:
- Direct labeling is preferred for quick applications where time is crucial.
- Indirect labeling is beneficial when high sensitivity is needed, for example, in detecting low-abundance proteins.
Fluorescence Mechanisms
Fluorescence mechanisms are pivotal in the understanding and application of labeled antibodies with fluorophores. These principles outline how fluorescent molecules behave under specific conditions, enabling researchers to visualize biological processes in real-time. Understanding these mechanisms allows for optimized experimental design, enhancing clarity and accuracy in various scientific disciplines. Each aspect, from excitation to emission and the overall retention of fluorescence, plays a crucial role in the effectiveness of fluorescence-based assays.
Excitation and Emission
Excitation and emission are the cornerstone of fluorescence principles. Fluorophores absorb photons at specific wavelengths. When excitation occurs, fluorophores become excited to a higher energy state. As they return to their ground state, they emit photons at a longer wavelength than the absorbed one.
The efficiency of this process is determined by several factors, including the type of fluorophore and the wavelength of the incident light. Selecting the right excitation wavelength is critical for optimizing the signal-to-noise ratio in fluorescence imaging.
Additionally, understanding the emission properties helps in distinguishing between different labeled antibodies in multiplex assays. This allows researchers to identify multiple targets within a single sample by utilizing fluorophores with distinct emission spectra.
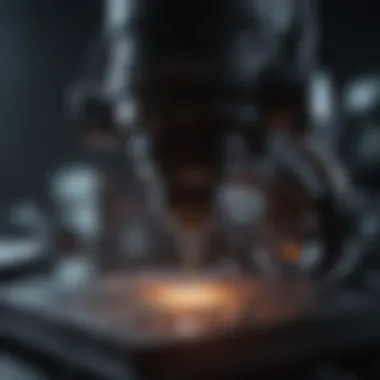
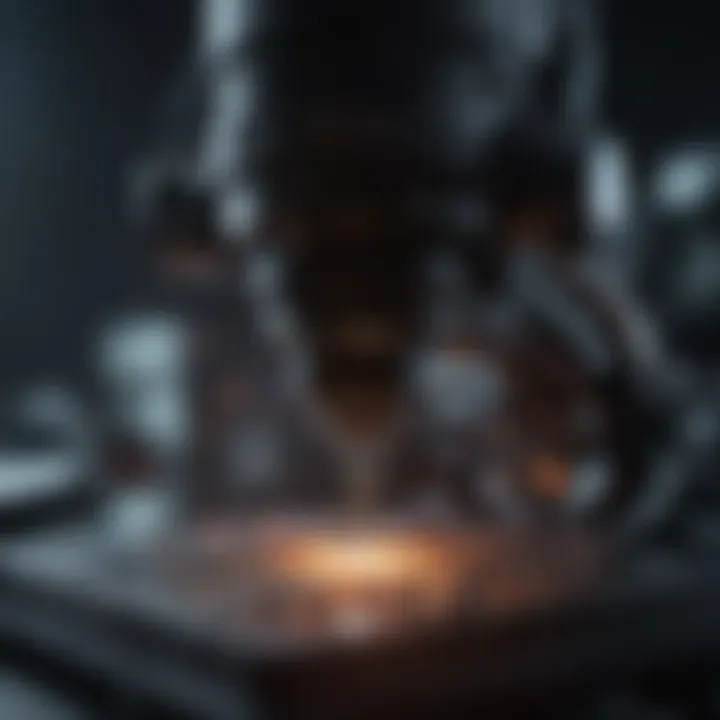
For practical applications, it is necessary to consider:
- Concentration of fluorophores: Higher concentrations may lead to changes in excitation and emission properties.
- Environment: Factors like pH and temperature can influence the behavior of fluorophores.
"Fluorescent signals can easily be misinterpreted without adequate understanding of their excitation and emission characteristics."
Fluorescence Lifetime
Fluorescence lifetime refers to the average time a fluorophore remains in its excited state before emitting a photon. This characteristic is crucial for distinguishing between different fluorescent species. The fluorescence lifetime can vary significantly based on factors such as solvent viscosity and the local environment of the fluorophore.
Understanding fluorescence lifetime can enhance the specificity of imaging techniques. For example, advanced methods like time-gated imaging rely on measuring the fluorescence lifetime to differentiate between overlapping signals in complex biological systems.
Key elements to consider in fluorescence lifetime include:
- Variability with Environment: The lifetime may change with changes in local pH or ionic strength.
- Applications in FRET: Fluorescence resonance energy transfer (FRET) techniques depend heavily on analyzing fluorescence lifetime to study interactions at the molecular level.
In summary, incorporating knowledge of fluorescence mechanisms into research protocols enables more precise and informative results when working with labeled antibodies. This insight not only supports studies in fundamental research but also offers significant implications for diagnostic developments.
Applications in Research
The application of labeled antibodies with fluorophores represents a significant advancement in biological research and diagnostics. These tools enhance the ability to visualize specific proteins or cells in complex biological systems. They provide researchers clarity in understanding cellular processes and interactions, leading to insights that can influence various scientific fields.
Immunofluorescence Microscopy
Immunofluorescence microscopy is a primary application of labeled antibodies with fluorophores. This technique enables the visualization of specific antigens in cells or tissue sections. By applying fluorophore-labeled antibodies that bind to target proteins, researchers can detect and study cellular localization in real time. The resulting fluorescence can be observed under a fluorescence microscope, aiding in the identification of cellular architecture and function.
This method is crucial in various research applications like pathology, cellular biology, and immunology. The specificity offered by these antibodies enhances the accuracy of diagnoses in diseases such as cancer. However, careful selection of appropriate fluorophores is essential to avoid issues such as overlapping fluorescence emissions.
Flow Cytometry
In flow cytometry, labeled antibodies with fluorophores allow for the analysis of individual cells suspended in a fluid stream. This technique can measure multiple parameters simultaneously, providing a comprehensive profile of the cell population. Each cell is passed through a laser beam, which excites the fluorophores. This excitation leads to emission of light that a detector measures, allowing identification and quantification of specific cell types based on their marker expression.
Flow cytometry is widely utilized in clinical laboratories for immunophenotyping, monitoring immune responses, and evaluating stem cell characteristics. The ability to analyze thousands of cells per second provides a high-throughput solution for researchers, enabling the analysis of complex mixtures of cells in various diseases including HIV, leukemia, and other hematologic disorders.
In Vivo Imaging
The use of labeled antibodies with fluorophores in vivo opens up new possibilities in medical research. In vivo imaging allows for the observation of biological processes in live animals, offering insights into disease progression and the effectiveness of treatments. For example, fluorescently labeled antibodies can target specific tumor sites, enabling non-invasive imaging to monitor tumor size and response to therapy.
This application highlights the role of fluorescence in understanding dynamics within live systems, such as tracking immune responses or evaluating biomarker expression over time. The challenges in in vivo imaging include the need for careful fluorophore selection to ensure adequate penetration and minimal background signal. Improvements in imaging technologies continually expand the possibilities of in vivo studies, further enhancing our understanding of complex biological processes.
The ability of labeled antibodies to provide real-time information in living organisms stands as a frontier in biomedical research.
In summary, the applications of labeled antibodies with fluorophores in research not only enhance visualization and analysis but also deepen our understanding of various biological phenomena. They serve as an indispensable toolset for researchers, educators, and clinicians alike.
Optimization of Labeling Procedures
Optimizing labeling procedures is critical for achieving accurate and reproducible results in the use of labeled antibodies with fluorophores. This optimization enhances sensitivity and specificity while reducing background noise in various applications. By fine-tuning these procedures, researchers can significantly improve the reliability of their findings. Factors influencing the outcome of labeling directly affect the entire experimental process. These factors can range from the nature of the antibodies and fluorophores used to environmental conditions such as temperature and pH.
In many scenarios, poor labeling efficiency can compromise the quality of results. This can lead to misinterpretation of data, which is particularly detrimental in sensitive experiments like immunoassays. Hence, understanding and optimizing the labeling process is necessary to ensure high-quality assays.
Factors Affecting Labeling Efficiency
There are several influential factors in labeling efficiency. Some of these include:
- Antibody Quality: The source of the antibody influences its binding capacity. Monoclonal antibodies often exhibit more consistency in performance compared to polyclonal antibodies.
- Concentration of Antibodies: The concentration chosen for the labeling reaction can affect the yield. If too low, insufficient labeling occurs; if too high, excess unbound antibodies may interfere with results.
- Fluorophore Chemistry: Different fluorophores have varying chemical properties that can impact stability and brightness. Choosing the appropriate fluorophore is crucial.
- Reaction Conditions: Factors such as temperature, time, and buffer composition can dramatically impact labeling efficiency. A controlled environment is necessary for optimal results.
- Product Quality of Fluorophores: The specific manufacturerβs protocols should be considered as some fluorophores are engineered for higher efficiency than others.
Optimizing these elements can lead to a more effective labeling process, ultimately resulting in enhanced experimental outcomes.
Strategies for Improvement
Improving labeling efficiency can be addressed through several strategic approaches:
- Thorough Optimization Trials: Conduct multiple trials adjusting variables such as antibody concentration and incubation times. This helps identify optimal conditions for specific combinations of antibodies and fluorophores.
- Use of Secondary Antibodies: Employing secondary antibodies conjugated with fluorophores can amplify signals, improving sensitivity without directly compromising primary antibodies.
- Testing Various Fluorophores: Experiment with different fluorophores that may be more compatible. Assess their photostability and emission characteristics to identify better options.
- Application of Blocking Agents: To minimize non-specific binding, use blocking agents effectively. These agents occupy available binding sites, reducing background noise.
- Implementing Advanced Labeling Techniques: Explore novel technologies such as click chemistry or site-specific labeling methods, which may offer improvements in efficiency and specificity.
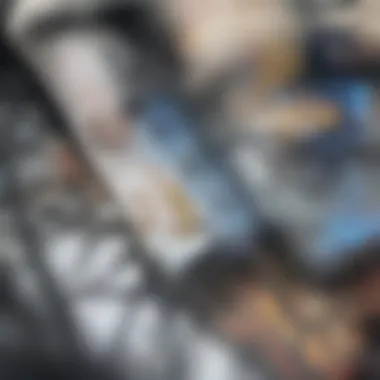
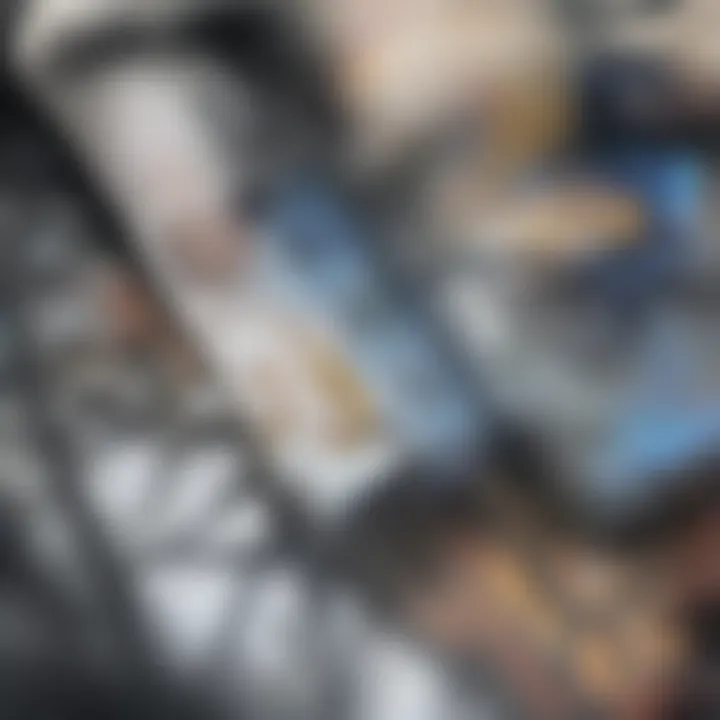
It is important to document all testing procedures and outcomes meticulously. This data can aid in the replication of successful experiments and foster further innovation in label optimization.
Conclusion: Optimizing labeling procedures is not just a necessity; it is a foundation for any robust experimental design involving labeled antibodies with fluorophores. Being methodical and strategic enhances the overall quality of research outcomes.
Challenges in Using Labeled Antibodies
Labeled antibodies have become integral tools in various research and diagnostic applications. However, several challenges can complicate their use. Understanding these challenges is vital for researchers, educators, and professionals working in immunology and related fields. Each issue emphasizes the need for careful consideration during experimental design and execution. The major challenges include photobleaching, non-specific binding, and cross-reactivity. Addressing these issues ensures more reliable and reproducible results.
Photobleaching
Photobleaching refers to the irreversible loss of fluorescence from a labeled molecule. It occurs when the fluorophore undergoes photochemical changes after absorbing light. This can significantly impact the quality and interpretability of results in various applications, especially in fluorescence microscopy. Photobleaching can vary based on the spectral properties of the fluorophore and the intensity of the excitation light.
When conducting experiments, one must often balance excitation energy and observation time to minimize photobleaching. Special techniques, such as employing more stable fluorophores, can help. Moreover, using time-lapse imaging or light-reduced settings may contribute to maintaining fluorescence. The implications of photobleaching are profound, as it can lead to erroneous interpretations of the spatial and temporal dynamics of labeled antibodies in live cells.
Non-specific Binding
Non-specific binding occurs when antibodies attach to unintended targets. This leads to background noise and complicates the analysis. Non-specific interactions can arise from various factors, including protein concentration, incubation time, and buffer composition. Addressing non-specific binding is crucial for enhancing signal-to-noise ratios in assays.
Researchers can minimize non-specific binding by optimizing blocking protocols with proteins or detergents. Further, it is essential to use well-characterized antibodies, preferably with validated protocols specific to the target. Failing to address this challenge can result in ambiguous data, rendering the use of labeled antibodies less reliable.
Cross-reactivity Issues
Cross-reactivity issues arise when an antibody binds to multiple targets. This can compromise the specificity of assays and lead to misleading conclusions. Such problems often stem from similarities in antigen structures or conformations, which may not be immediately obvious. Identifying these interactions requires rigorous validation steps, increasing the complexity of experimental designs.
Strategies to combat cross-reactivity include thorough characterization of antibody specificity through methods such as ELISA or western blotting. Employing monoclonal antibodies may also reduce cross-reactivity by targeting unique epitopes. Ultimately, recognizing and addressing cross-reactivity is essential to avoid pitfalls in experimental work, ensuring that results reflect true biological phenomena rather than artifacts.
"Understanding the challenges associated with labeled antibodies is critical for driving advancements in biological research."
The complexities of using labeled antibodies necessitate a comprehensive understanding of potential pitfalls. By carefully considering challenges like photobleaching, non-specific binding, and cross-reactivity, researchers can improve their methodologies, leading to more robust and insightful outcomes.
Future Directions
The exploration of labeled antibodies with fluorophores is not stagnant; it evolves continuously. This section discusses the upcoming trends and techniques in this field. As researchers strive for better accuracy and efficiency in biological assays, understanding future directions becomes essential. The focus will be on emerging fluorophore technologies and how they integrate with novel techniques. This understanding is vital for expanding the applications of labeled antibodies in cutting-edge research.
Emerging Fluorophore Technologies
Advancements in fluorophore technologies are critical in enhancing the effectiveness of labeled antibodies. New classes of fluorophores are being developed that improve brightness and stability. For instance, the emergence of new organic fluorophores provides greater spectral diversity and higher quantum yields.
- Enhanced Stability: Newer fluorophores demonstrate resistance to photobleaching, making them more reliable in long-duration experiments.
- Smaller Size: Recent developments in nanocrystals, such as quantum dots, allow for multiplexing without significant overlap in emission spectra.
- Environmental Sensitivity: Researchers are also focusing on finding fluorophores whose fluorescence properties change in response to environmental factors, paving the way for real-time monitoring in live cells.
These innovations are crucial for expanding the utility of labeled antibodies in various applications, including diagnostics and therapeutics. The integration of such advanced fluorophores can lead to improved outcomes in both basic research and clinical settings.
Integration with Novel Techniques
Integrating labeled antibodies with novel techniques adds another layer of depth to their applications. Technologies like CRISPR and advanced imaging modalities can benefit immensely from the use of labeled antibodies.
- CRISPR Technology: By tagging CRISPR components with fluorophores, scientists can visualize and track gene-editing processes in real-time within living cells. This integration helps confirm the efficiency of targeted delivery systems.
- Super-resolution Microscopy: Recent advancements in super-resolution microscopy techniques can significantly amplify the applications of labeled antibodies. These methods provide detailed spatial resolution, allowing the observation of cellular structures at unprecedented levels.
- High-throughput Screening: The combination of labeled antibodies with high-throughput technologies facilitates large-scale analysis of interactions. This method is beneficial for drug discovery and development phases.
These integrations not only enhance research capabilities but also enable new applications across various disciplines, including genetics, cancer research, and developmental biology.
The future in using labeled antibodies with fluorophores lies substantially in the developments of technology. As these technologies expand, so too will the potential for new scientific discoveries.
End
The importance of the conclusion in this article cannot be overstated. It serves as the final synthesis of explored themes, reinforcing the critical role that labeled antibodies with fluorophores play in modern biological research and diagnostics. Summarizing the key points not only strengthens the reader's understanding but also provides a clear takeaway for their future applications.
This comprehensive guide highlights several specific elements. First, the mechanisms behind labeling antibodies significantly enhance detection methods. By utilizing fluorophores, researchers can achieve greater specificity and sensitivity in their assays. The optimization techniques discussed help researchers to refine their experiments, ensuring that the labeling processes yield reliable and reproducible results.
Moreover, considerations regarding future research directions emerge in the conclusion. Identifying challenges like photobleaching and non-specific binding highlights areas that require further innovative solutions. Overall, the conclusion encapsulates the benefits derived from incorporating labeled antibodies with fluorophores into scientific studies, pushing the boundaries of what researchers can achieve in understanding biological systems.
Summary of Key Points
- Labeled antibodies with fluorophores enhance specificity and sensitivity in assays.
- Fluorophore technologies continue to evolve, providing researchers with innovative tools.
- Challenges such as photobleaching and non-specific binding impact the effectiveness of fluorescence methods.
- Optimizing labeling procedures increases the reliability of experimental results.
Implications for Future Research
The implications for future research in the domain of labeled antibodies with fluorophores are significant. As researchers continue to bridge the gaps in the current methodologies, several areas warrant attention:
- Emerging Technologies: New fluorophore design strategies could lead to more effective labeling techniques that reduce non-specific binding and maximize fluorescence.
- Hybrid Approaches: Combining labeled antibodies with other imaging modalities could improve the overall resolution and context of biological samples.
- Cross-disciplinary Methods: Integrating labeled antibodies with computational tools will elevate data analysis and interpretation, enhancing the understanding of complex biological processes.
- Therapeutic Applications: Exploring the role of labeled antibodies in drug delivery systems might revolutionize personalized medicine, making treatments more precise.
In summary, the article underscores the pivotal role of labeled antibodies with fluorophores, reiterating their importance in both current research and future innovations. As the field progresses, continuous exploration is essential for developing novel strategies that push the boundaries of detection and analysis.