Innovations and Applications of Molecular Devices
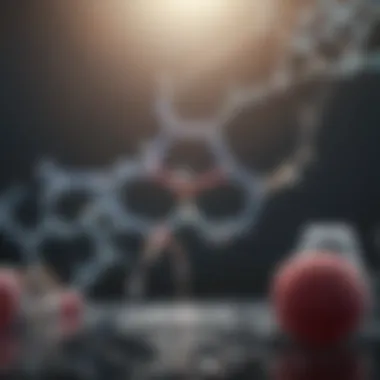
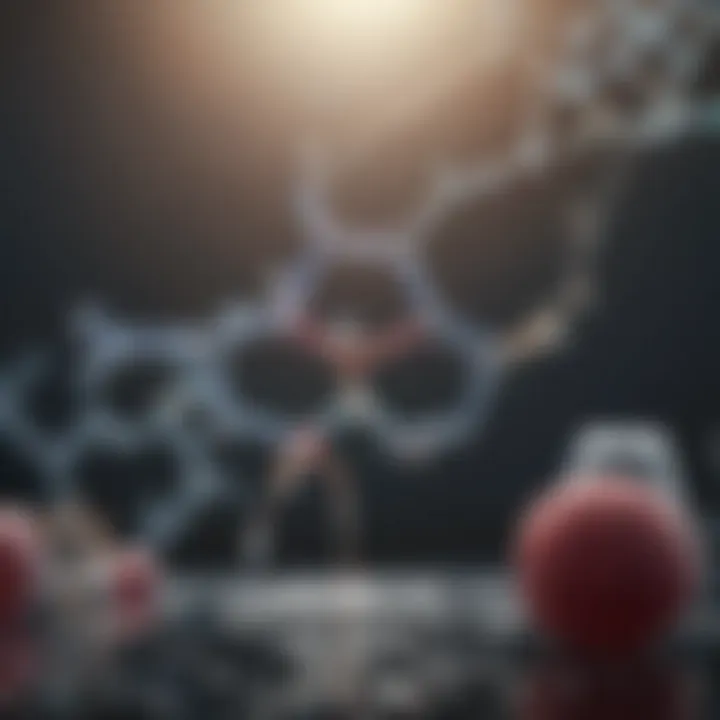
Intro
Molecular devices are pivotal in modern scientific advancements, bridging the gap between chemistry, physics, and engineering. They consist of nanoscale components that enable unique functionalities, which makes them essential for diverse applications such as sensing, energy harvesting, and computing. The exploration of these devices sheds light on their innovative design and mechanisms, allowing researchers to harness their potential across various scientific disciplines.
This article aims to unpack the intricate nature of molecular devices, focusing on recent breakthroughs and their implications for future research and technology.
Recent Advances
Molecular devices have seen remarkable progress in recent years, fueled by interdisciplinary collaboration and technological advancements. This section focuses on the latest discoveries and innovations that shape the current landscape of molecular devices.
Latest Discoveries
Scientists have made significant strides in the synthesis of novel molecular components, leading to enhanced functionality. Recent research highlights include:
- Molecular Switches: These devices can manipulate electrical signals in response to specific stimuli, offering potential applications in data storage and logic circuits.
- Bio-inspired Systems: Researchers are developing devices that mimic natural processes, such as energy conversion in photosynthesis, which could revolutionize renewable energy sources.
- Nanomachines: The creation of nanoscale machines that can perform tasks at the molecular level is a groundbreaking development, enabling applications in targeted drug delivery and material synthesis.
Technological Innovations
Modern technology continues to push the limits of molecular devices. Key innovations involve:
- Nanostructured Materials: The use of graphene, carbon nanotubes, and other advanced materials enhances the efficiency and capability of molecular devices.
- Flexible Electronics: The integration of molecular components with flexible substrates allows for the development of wearable technologies that can monitor health and environmental conditions.
- Quantum Dots: These semiconductor particles have unique optical properties, paving the way for advancements in displays and solar cells.
"Molecular devices have the potential to revolutionize fields ranging from electronics to medicine, providing innovative solutions to complex problems."
Methodology
Understanding the innovations and applications in molecular devices requires a rigorous approach to research design and data analysis.
Research Design
The study of molecular devices often involves experimental and theoretical frameworks. Researchers utilize a combination of laboratory experiments and computational modeling to draw meaningful conclusions about molecular assembly and functionality.
Data Collection Techniques
Various techniques are employed to collect data, including:
- Spectroscopy: This method helps analyze molecular interactions and reactions, allowing scientists to observe real-time processes.
- Electron Microscopy: High-resolution imaging techniques provide insights into the structure and behavior of molecular devices at the nanoscale.
- Simulation Software: Advanced modeling tools are used to predict molecular behaviors and interactions prior to experimental validation, streamlining the research process.
Intro to Molecular Devices
Molecular devices represent a significant intersection of molecular biology, chemistry, and engineering. Their study facilitates a deeper understanding of how molecular structures can be harnessed for technological applications. This article aims to shed light on this intricate field, emphasizing the vital role molecular devices play in revolutionizing industries such as medicine, environmental science, and information technology.
Defining Molecular Devices
Molecular devices can be fundamentally described as systems that utilize individual molecules or assemblies of molecules to perform specific operations or functions. These devices often exhibit unique properties that stem from their molecular makeup. The ability to control their operation at the molecular level grants them a range of functionalities, making them pivotal in areas such as data storage, sensing, and catalysis.
Key characteristics of molecular devices include:
- Scalability: Their small size means they can be integrated into larger systems or assembled in vast quantities.
- Versatility: They can be engineered for various purposes, from molecular switches to sensors.
- Efficiency: Molecular devices can operate at lower energy levels compared to traditional devices, often leading to reduced operational costs.
Historical Context
The concept of molecular devices is not entirely modern. Early explorations date back to the 1980s when scientists began examining how molecules could be manipulated for practical applications. The work of researchers like Jean-Pierre Sauvage, who pioneered the field of supramolecular chemistry, laid the groundwork for understanding how complex molecular assemblies can function as devices.
As technology advanced, so did the understanding and capabilities related to molecular devices. Breakthroughs in synthetic chemistry allowed for the design of molecules with tailored functions. With the advancement of nanotechnology in the late 20th century, molecular devices began to see broader applications, particularly in biomedical fields. The capability to create functional devices at the nanoscale paved the way for innovation, leading to contemporary developments that integrate molecular devices in diagnostics, drug delivery, and environmental monitoring.
Through this historical lens, one can appreciate not just the scientific achievements but also the increasing relevance of molecular devices in solving real-world problems. The ongoing evolution of this field continues to promise advancements that could be transformative across various sectors.
Fundamental Principles of Molecular Devices
Understanding the fundamental principles of molecular devices is essential in order to grasp their underlying function and broad applications. These principles dictate how molecules interact, respond to external stimuli, and perform specific tasks. They also provide insight into how innovations in this field can lead to significant advancements in technology and science. The interrelationship between molecular mechanics, optoelectronic properties, and thermodynamics creates a foundational framework that governs the behavior and efficacy of molecular devices.
Molecular Mechanics
Molecular mechanics focuses on the physical principles that govern the interactions between molecules. It uses classical physics to model molecular behavior without delving into quantum mechanics, which simplifies the understanding of molecular systems.
The main components include:
- Atoms and Bonds: The arrangement and type of atoms in a molecule heavily influence its properties and reactivity.
- Force Fields: These are mathematical functions that describe how molecules interact. They account for forces such as bond stretching and angle bending.
- Energy Minima: Systems tend to move towards states of lower energy. This principle is key in predicting molecular conformation and stability.
The comprehension of molecular mechanics is crucial when designing devices, optimizing their performance and ensuring reliability under various conditions. Designing molecular devices requires a careful balance of these mechanical principles to enhance functionality while preserving structural integrity.
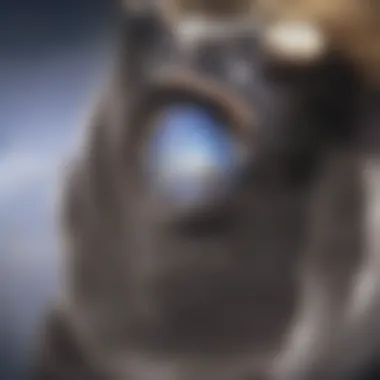
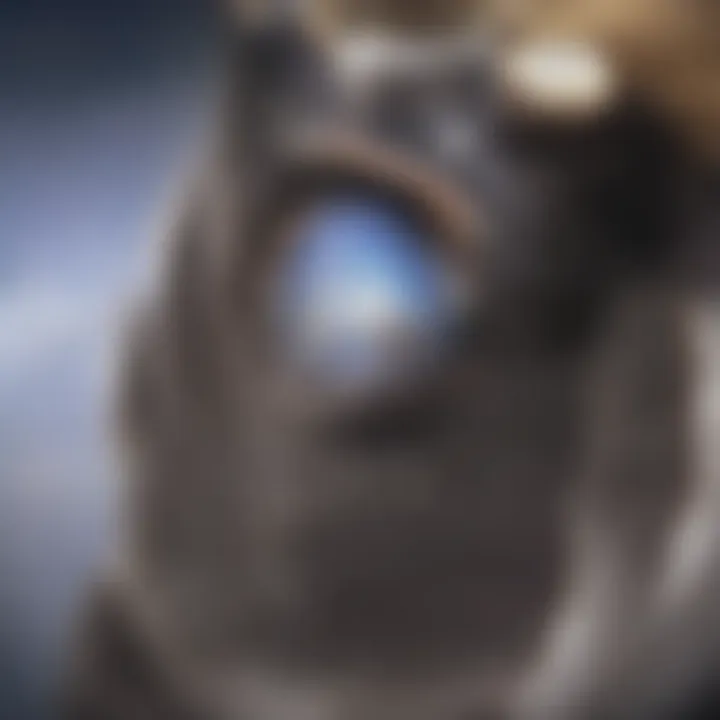
Optoelectronic Properties
Optoelectronic properties refer to the interaction between light and electrically charged particles in a material. Understanding these properties is vital for the development of devices such as sensors, transistors, and photovoltaic cells.
Key factors include:
- Absorption of Light: Molecular devices must efficiently absorb light to convert it into electrical signals or energy. This property determines the effectiveness of solar cells and LED technology.
- Charge Transport: The ability of a molecule to transport charge carriers impacts the overall performance of electronic applications. Poor charge mobility can limit device efficiency.
- Photoconductivity: This phenomenon, where a material's conductivity increases due to incident light, is foundational for numerous optoelectronic applications.
The synthesis and application of materials with tailored optoelectronic properties play a transformative role in the design of advanced molecular devices in the ever-evolving field of electronics and photonics.
Thermodynamics in Molecular Systems
Thermodynamics is the branch of physics that deals with heat and temperature and their relation to energy and work. In the context of molecular devices, it provides a framework for understanding energy changes and stability during molecular interactions and reactions.
Important concepts include:
- Gibbs Free Energy: Changes in Gibbs energy help predict whether a reaction will occur spontaneously. This concept is crucial for understanding reaction mechanisms in molecular devices.
- Entropy and Enthalpy: The interrelationship of these two thermodynamic properties aids in the assessment of stability and reactivity of molecular systems. Increased disorder (entropy) can drive processes even if they require energy input (enthalpy).
- Temperature Effects: Molecular behavior is highly temperature dependent. Knowing how devices respond to temperature variations is key to ensuring performance and longevity.
In summary, thermodynamics plays an essential role in the design and analysis of molecular devices, influencing their operational efficiency and stability.
Understanding these fundamental principles allows researchers and developers to innovate more effectively in the field of molecular devices.
By comprehensively exploring these principles, we can connect the dots between theory and practical applications, strengthening the knowledge base required to propel future advancements in the domain of molecular devices.
Types of Molecular Devices
Molecular devices represent a groundbreaking integration of chemistry and engineering. This section discusses the various types that exist in this domain. Each type is important for advancing technology and discovering new applications. The versatility of molecular devices makes them crucial in both research and practical applications. By understanding different types, we can appreciate their potential to revolutionize fields such as medicine, environmental science, and information technology.
Molecular Switches
Molecular switches are fundamental components in the world of molecular devices. These devices can change their state in response to external stimuli. Common stimuli include electrochemical signals, light, and changes in pH. The ability to toggle states makes them ideal for applications in data storage and molecular computing.
One major benefit of molecular switches is their potential for ultra-miniaturization. Traditional electronic components are often bulk and hinder the advancement of nanoelectronics. By employing molecular switches, we can achieve much smaller and more efficient devices.
Molecular switches can provide unique functionalities, enabling systems that mimic biological processes.
Applications in memory devices and sensors are particularly promising. The chemical elegance of these switches allows for diverse applications. They can play pivotal roles in batteries, where they help regulate energy flow according to demand.
Molecular Sensors
Molecular sensors are another vital category within molecular devices. These sensors are designed to detect specific chemical or biological entities. They operate by producing measurable signals in response to the presence of targeted analytes. Their sensitivity is unmatched in some cases, achieving detection limits previously thought impossible.
These devices find extensive use in medicine and environmental monitoring. For example, they can detect pathogens in bloodstream samples or analyze pollutants in water. This capability is essential for public health and environmental preservation. Molecular sensors operate on principles ranging from fluorescence to electrochemical responses.
The integration of molecular sensors into wearable technology showcases their potential in personal health monitoring. Continuous glucose monitoring systems for diabetes patients exemplify the practical benefits brought by molecular sensor technology.
Molecular Motors
Molecular motors highlight the potential of molecular devices to perform mechanical work. These devices can convert chemical energy into motion. They are inspired by biological motors seen in living organisms. Understanding these systems opens new avenues in robotics and nanotechnology.
One exciting aspect of molecular motors is their ability to operate at the nanoscale. This means they can manipulate molecules with incredible precision. Applications may include targeted drug delivery, where motors can transport therapeutic agents directly to cells.
The fascination with molecular motors extends to the development of synthetic versions. Ongoing research is dedicated to creating motors that can function autonomously in different environments.
In summary, the types of molecular devicesβswitches, sensors, and motorsβserve distinct but interrelated functions. They collectively push the boundaries of current technology and hold immense promise for future innovations.
Synthetic Approaches in Molecular Device Fabrication
Synthetic approaches in molecular device fabrication are crucial for advancing the capabilities of molecular devices. The effective synthesis of these devices not only impacts their performance but also their scalability and integration into existing technologies. As molecular devices become more prevalent in various applications, the methodologies for their fabrication must ensure precision and reliability.
Synthetic approaches can be broadly categorized into two main techniques: bottom-up assembly and top-down fabrication. Each of these methods offers distinct advantages and has unique considerations that researchers and developers must account for. A thorough understanding of these approaches allows scientists and engineers to choose the best fabrication method based on specific requirements, whether it be for molecular switches, sensors, or other applications.
Bottom-Up Assembly Techniques
Bottom-up assembly techniques focus on constructing molecular devices from smaller, simpler building blocks. This approach relies on chemical processes that assemble molecules into larger structures. Often, it begins at the atomistic level, where individual molecules are combined through various interactions, such as covalent bonding, van der Waals forces, and hydrogen bonding.
The benefits of bottom-up techniques include:
- High precision: These techniques allow for precise control over molecular arrangement and orientation.
- Diversity of structures: They enable the synthesis of a wide range of molecular architectures, resulting in the creation of unique device functionalities.
- Cost-effective: Generally, this method reduces the consumption of materials, leading to lower production costs.
However, there are considerations to be taken into account, such as the complexity of the self-assembly process, potential limitations in scalability, and reproducibility of device performance in different batches.
Top-Down Fabrication Methods
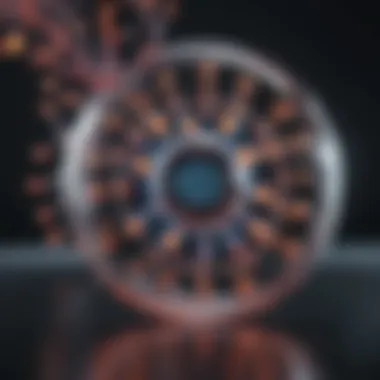
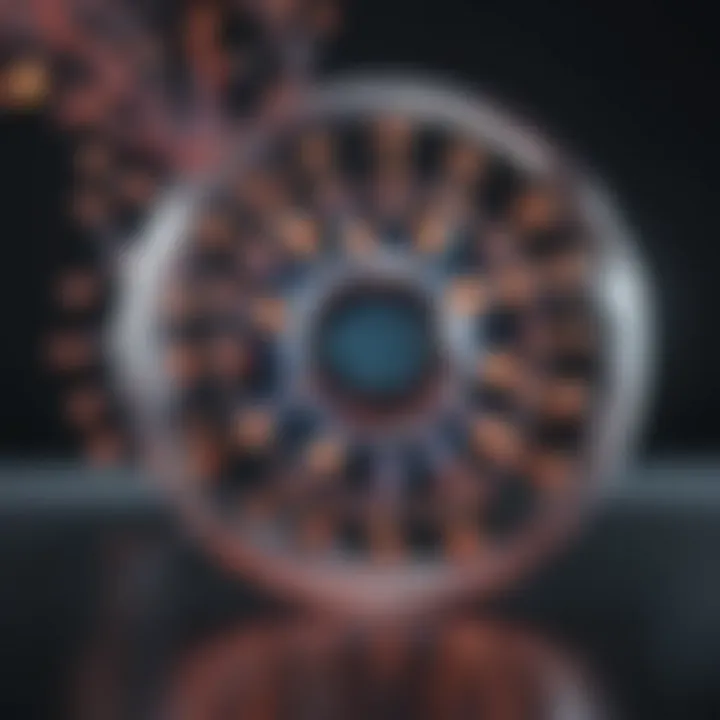
In contrast to bottom-up techniques, top-down fabrication begins with a larger structure that is systematically broken down into smaller components. This approach is akin to traditional semiconductor fabrication methods and often involves physical and chemical processes to shape materials into desired forms.
Advantages of top-down fabrication include:
- Scalability: This method can easily be adapted for mass production, making it suitable for commercial applications.
- Integration with existing technologies: It allows for the incorporation of molecular devices with current manufacturing processes in electronics and material science.
- Uniformity: Top-down techniques can produce devices with consistent properties across larger scales.
Despite these advantages, challenges such as precision limitations, waste generation, and potential defect formation remain prevalent. Careful optimization of the processes is necessary to overcome these hurdles and achieve the desired outcomes in molecular device fabrication.
Overall, the choice between bottom-up and top-down methods largely depends on the specific application, desired scale, and particular characteristics of the molecular devices being developed.
As the field progresses, innovative hybrid approaches may emerge, combining elements from both methodologies to enhance device performance and functionality.
Characterization Techniques
Characterization techniques are pivotal in the field of molecular devices. They enable researchers to gain insights into the properties and functionalities of these devices. Understanding these techniques is key for optimizing performance in practical applications. The right characterization method can yield critical data about molecular interactions, structural integrity, and response to various stimuli. This information fosters innovation and helps drive advancements in molecular technology.
Spectroscopic Methods
Spectroscopic methods are vital tools for probing molecular features. Techniques like UV-Vis spectroscopy, infrared spectroscopy, and nuclear magnetic resonance (NMR) provide a window into molecular behavior. Each method has unique advantages. For example, UV-Vis spectroscopy is excellent for analyzing electronic transitions, while infrared spectroscopy is adept at identifying molecular vibrations.
- UV-Vis Spectroscopy: This method allows the detection of electronic transitions within molecules. It helps in determining the absorbance and transmittance of light, facilitating the study of electronic structures.
- Infrared Spectroscopy: Useful for identifying functional groups, this technique looks at molecular vibrations. It can characterize various compounds, offering detailed information about chemical bonding.
- NMR: This technique offers insights into molecular structure and dynamics by examining magnetic properties of atomic nuclei. It is essential for understanding complex molecular architectures.
These methods often work in conjunction, providing a holistic view of molecular devices.
Electrochemical Analysis
Electrochemical analysis is crucial to understanding the electrochemical behavior of molecular devices. It focuses on the relationship between electrical currents and chemical reactions. Techniques such as cyclic voltammetry and impedance spectroscopy are prevalent in this field.
- Cyclic Voltammetry: This method investigates the redox properties of molecules. By varying the potential applied to an electrode, researchers can observe current responses, which reveals information about electron transfer kinetics.
- Impedance Spectroscopy: This technique measures the impedance of a system as a function of frequency. It provides data about charge transport and reaction kinetics at the electrode interface.
Real-time analysis is possible with these methods, facilitating the development of more efficient molecular devices.
Microscopic Techniques
Microscopic techniques offer unparalleled insights into the physical structures of molecular devices. High-resolution imaging methods like atomic force microscopy (AFM) and transmission electron microscopy (TEM) allow researchers to observe nanoscale features.
- Atomic Force Microscopy (AFM): AFM provides three-dimensional surface profiles of materials at the nanoscale. It helps measure surface roughness, thickness, and mechanical properties.
- Transmission Electron Microscopy (TEM): TEM enables imaging at atomic resolutions, making it invaluable for studying crystal structures and defects in molecular devices.
These microscopic techniques illuminate the relationship between structure and function, guiding development efforts.
"Characterization techniques are essential for unlocking the full potential of molecular devices, informing design and optimization strategies."
In summary, these characterization techniques provide a comprehensive understanding of molecular devices. They ensure the development process is grounded in empirical data, ultimately driving innovation and application across various sectors.
Applications of Molecular Devices
The applications of molecular devices are paramount in advancing technology and science. Engaging with this topic offers profound insights into how these devices contribute to various fields. Their versatility and precision enhance functionalities across medicine, environmental monitoring, and information technology. Each application addresses specific challenges, providing innovative solutions that underscore the significance of molecular devices in the modern world. Understanding these applications helps to appreciate the impact and potential of molecular devices.
In Medicine
Molecular devices in medicine have revolutionized diagnostics and therapeutic techniques. These devices allow for targeted therapies, improving treatment efficacy and reducing side effects. For example, molecular sensors can detect biomarkers for diseases at very low concentrations, enabling early diagnosis. Moreover, molecular motors can facilitate drug delivery systems, ensuring precise targeting of afflicted areas within the body.
In addition, advancements in DNA-based devices have led to novel approaches in gene editing and personalized medicine. Technologies like CRISPR rely on molecular devices to edit genomes with high accuracy. This capability is instrumental in treating genetic disorders, highlighting the potential of molecular devices in transforming healthcare.
"The synergy between molecular devices and medical applications promises a new era of precision medicine, tailored to individual patient needs."
In Environmental Monitoring
Environmental monitoring is another crucial application of molecular devices. These devices enable real-time tracking of pollutants and hazardous substances in ecosystems. For instance, molecular sensors are capable of detecting specific contaminants at minute levels, providing valuable data for environmental protection efforts. This information supports timely interventions, which can mitigate harmful effects on biodiversity and human health.
Moreover, the development of biodegradable molecular devices offers potential solutions to pollution. These devices can break down over time, reducing their ecological footprint. They can be utilized in soil and water testing, allowing for sustainable monitoring practices. This capability is vital in preserving ecosystems and ensuring a healthy environment for future generations.
In Information Technology
The role of molecular devices in information technology is particularly fascinating. Molecular computing and quantum-dot based devices represent a significant leap in processing power and data storage. These devices leverage the unique properties of molecules to perform calculations at speeds far beyond traditional semiconductors.
For example, molecular logic gates can perform complex computations, paving the way for miniaturization and energy-efficient computing. Furthermore, the integration of molecular devices into nanoscale electronics holds the promise of next-generation technologies. These devices can facilitate increased data density and faster processing speeds, making them integral to future advancements in this field.
In summary, the applications of molecular devices illustrate their expansive potential. From enhancing medical treatments and safeguarding the environment to revolutionizing information technology, these devices play a crucial role in shaping a better future. Their continued development will likely lead to even more innovative uses across different sectors.
Case Studies of Notable Molecular Devices
Exploring case studies of notable molecular devices is crucial for understanding the practical implications of these innovations. They provide concrete examples of theoretical concepts, showcasing the real-world impact of molecular devices in various fields. This section highlights some significant advancements, offering insights into the challenges and benefits associated with each type of device.
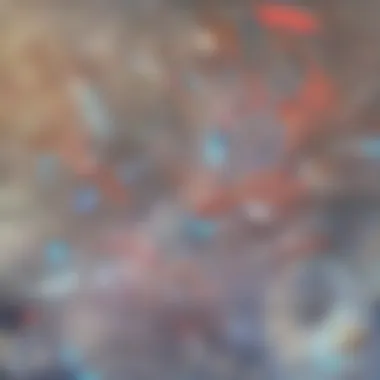
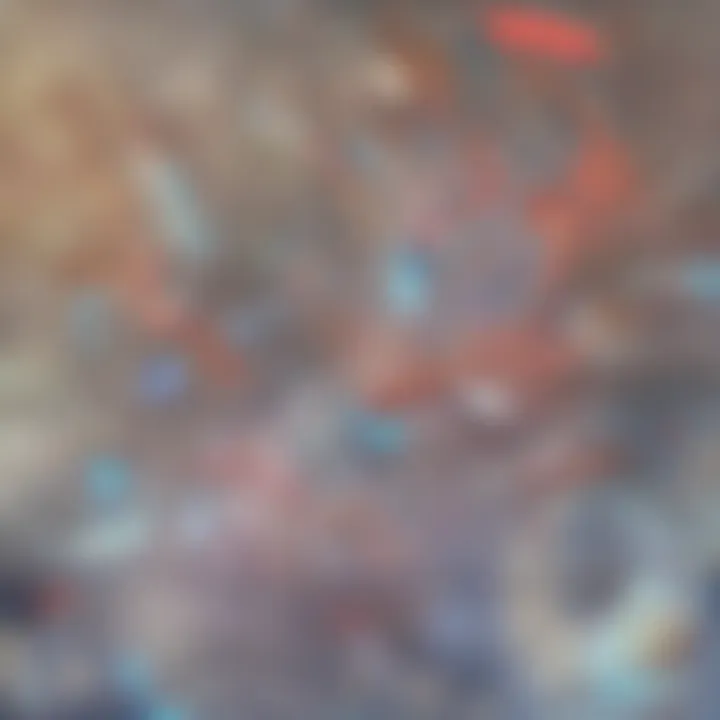
Molecular Logic Gates
Molecular logic gates represent a unique intersection of chemistry and information technology. These devices mimic the fundamental operations of electronic logic gates but utilize molecular interactions. By achieving logic functions at the molecular level, they enable the development of sophisticated computation systems.
One prominent example is the AND gate constructed from DNA strands. When two specific DNA sequences are present, the gate produces a signal. This mechanism opens possibilities for complex computations in very small spaces. Molecular logic gates are important not only for processing information but also for creating diagnostic tools in medicine, where they can respond to specific biomolecules.
"Molecular logic gates may revolutionize computing, providing levels of miniaturization previously thought impossible."
DNA-Based Devices
DNA-based devices leverage the inherent properties of DNA molecules for innovative applications. They demonstrate versatility in sensing, diagnostics, and targeted drug delivery. A notable case is DNA origami, a method for folding DNA strands into specific shapes, which can be used to create nanoscale devices.
These devices can operate as biosensors, responding to specific biological substances. For instance, researchers have developed DNA-based sensors that detect cancer biomarkers at very low concentrations, enabling early diagnosis. Additionally, they can deliver drugs selectively to cancer cells, reducing side effects associated with conventional treatments.
The scalability and programmability of DNA make it a potent tool in molecular device development. As the field advances, more applications in synthetic biology and personalized medicine are expected to emerge.
Nanoparticle Sensors
Nanoparticle sensors harness the properties of nanoparticles to detect specific chemical and biological agents. These sensors often use metals like gold and silver due to their unique optical properties. An example includes using gold nanoparticles for detecting glucose, which is crucial for diabetes management.
The efficiency of nanoparticle sensors lies in their ability to provide rapid results with high sensitivity. They can be designed to change color or fluorescence in response to the presence of specific molecules. This characteristic makes them valuable in environmental monitoring, ensuring safety and compliance in various industries.
As research progresses, nanoparticle sensors are being integrated into wearable technology, offering real-time health monitoring and improving health outcomes.
Challenges in Molecular Device Development
Molecular devices represent a frontier in scientific innovation, yet their development comes with significant challenges. Recognizing these challenges is crucial for fostering advancements in this domain. As molecular devices are integrated into various fields like medicine, electronics, and environmental monitoring, the hurdles faced during their development can influence their effectiveness and viability in real-world applications.
Stability and Reliability
Stability and reliability are fundamental aspects in the development of molecular devices. The performance of these devices can be severely impacted by environmental factors such as temperature, humidity, and chemical stability. For example, molecular sensors used for detecting pollutants must maintain high functionality in changing conditions. Any degradation in performance could lead to incorrect readings, which could have serious consequences.
Key considerations include:
- Material selection: Choosing materials that can withstand environmental stresses contributes to device longevity.
- Protective coatings: Applying coatings can help shield devices from harsh conditions, increasing stability.
- Rigorous testing: Extensive testing protocols must be established to ensure reliability over time under various conditions.
"The future of molecular devices hinges on overcoming stability issues, ensuring they can perform reliably in diverse applications."
Scalability Issues
Scalability is another pressing challenge in molecular device development. The transition from laboratory-scale prototypes to commercially viable products can encounter obstacles. Factors such as cost, manufacturing processes, and material availability can significantly affect scalability.
Critical elements to consider include:
- Economic feasibility: It's essential to assess if the production cost aligns with market expectations.
- Manufacturing techniques: Innovations in fabrication techniques, such as roll-to-roll printing or microfabrication, can enhance scalability.
- Supply chains: Establishing reliable sources for required materials is vital for consistent production.
Achieving scalable molecular devices involves not just technological advancements but also a thorough understanding of market dynamics and production logistics.
Future Perspectives
The future of molecular devices presents a landscape rich with potential advancements and innovations. As the scientific community continues to unravel the complexities of molecular systems, it becomes increasingly clear that these devices hold significant promise in various fields. The importance of discussing future perspectives lies in their ability to shape research agendas and inspire new applications that can positively impact society.
Emerging Trends
Several key trends are emerging in the study and application of molecular devices. One such trend is the integration of artificial intelligence in the design of molecular systems. AI can facilitate the prediction of molecular behavior, leading to more efficient designs that enhance device functionality. Furthermore, there is a growing interest in biomimetic materials that mimic biological processes for use in sensors and actuators. This approach not only offers sustainability but also opens avenues for innovation in personalized medicine.
Additionally, advancements in nanotechnology are set to revolutionize molecular devices. As researchers develop smaller, more sophisticated components, applications in biomedical diagnostics and treatment are likely to expand. The convergence of disciplines, including materials science, biology, and electrical engineering, is helping give rise to hybrid systems that combine the strengths of different technologies.
- Utilization of AI in molecular design
- Growth of biomimetic materials
- Progress in nanotechnology for diagnostics
Societal Impact
The implications of advancements in molecular devices extend beyond the lab. These technologies can drive significant societal change. For instance, in medicine, molecular devices have the potential to transform personalized healthcare, allowing for treatments tailored to individual genetic profiles. Moreover, molecular sensors can enhance environmental monitoring, enabling quicker and more accurate responses to pollutants.
As these devices become more prevalent, ethical considerations will arise. Issues such as data privacy in medical applications and the environmental impact of nanoscale materials must be addressed. Public awareness and dialogue about these challenges are essential as we navigate this new terrain. The convergence of scientific research and societal needs will ultimately shape the direction of molecular device development.
"Emerging molecular devices are not just tools; they are solutions that can address some of the most pressing challenges facing humanity."
The future of molecular devices is promising, with innovations poised to affect many aspects of daily life while addressing global challenges. The interplay between emerging trends and societal impact will define the trajectory of molecular devices, ensuring they remain at the forefront of scientific and technological advancements.
Closure
The conclusion of this article serves as a pivotal element in reinforcing the knowledge obtained about molecular devices. It wraps up the myriad innovations and applications discussed throughout the text, synthesizing the information into a coherent overview.
Understanding molecular devices is crucial because they are at the forefront of several scientific advancements. Their relevance spans various fields including medicine, environmental monitoring, and information technology. The potential to revolutionize these areas makes it imperative to grasp not just the theoretical underpinnings but also real-world applications.
One significant element discussed is the future perspectives on molecular devices. Emerging trends indicate a growing trend towards integration with nanotechnology and biotechnology. This integration could lead to enhanced functionalities in devices, improving their efficiency and applicability in real-world scenarios.
Moreover, the challenges in development, such as stability and scalability, provide important considerations that warrant attention. Addressing these obstacles is key for future advancements. Innovative solutions must be found to enhance the reliability and durability of molecular devices, paving the way for commercial usage and broader acceptance.