Exploring Plant Cell Components and Their Vital Roles
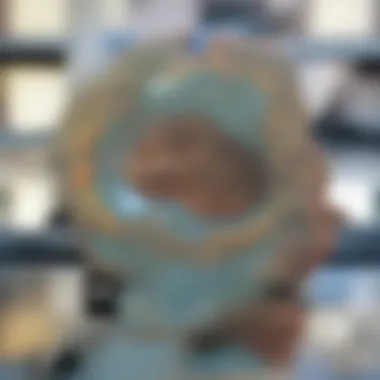
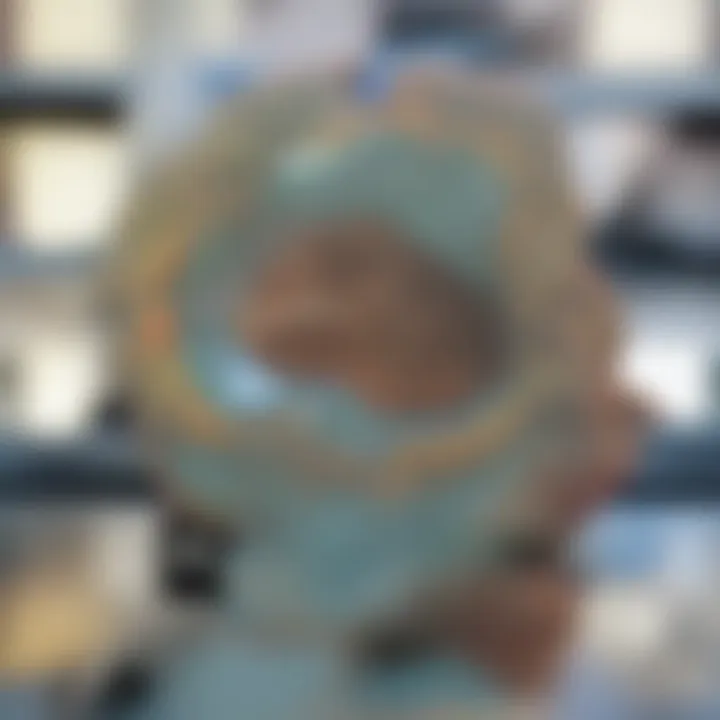
Intro
Understanding the structure and function of plant cells is crucial for many fields such as biology, agriculture, and environmental science. Plant cells are complex entities, each organelle playing a significant role. This article aims to dissect the intricate components of plant cells, revealing how they contribute to the overall health of the plant. From photosynthesis in chloroplasts to support from the cell wall, every part has specific duties that sustain plant life. By analyzing these organelles, we can appreciate the sophisticated biological processes that govern plant growth and development.
Recent Advances
Recent research has expanded our knowledge of plant cellular biology. Scientists continuously uncover new insights about plant cell parts, paving the way for innovative applications in agriculture and environmental conservation.
Latest Discoveries
In recent years, researchers identified new functions of certain organelles. For example, studies have shown that vacuoles, once thought primarily for storage, also play critical roles in cellular signaling. This finding underlines the dynamic nature of plant cells and challenges previous assumptions about their functionality.
Additionally, some studies focus on the role of ribosomes in plants. Ribosomes are not just protein factories; they also influence plant responses to environmental stress. Investigating these details reshapes our understanding of how plants adapt to their surroundings.
Technological Innovations
The advancement of imaging technologies has transformed how plant cells are studied. Techniques like super-resolution microscopy allow researchers to observe organelles at ultra-high resolutions. This innovation reveals the intricate structures and interactions within the cells that were previously undetectable. Moreover, genetic editing tools like CRISPR-Cas9 are enabling targeted modifications to study specific cellular functions in plants more effectively.
Methodology
A structured approach to studying plant cell components enhances the reliability of findings. Methodological rigor is essential when tackling complex biological questions.
Research Design
Research in plant cellular biology often employs both observational and experimental designs. Observational studies may focus on the behavior of plants under various conditions, while experimental approaches might manipulate specific variables to assess their impacts on cell functions.
Data Collection Techniques
Data collection usually involves diverse techniques such as:
- Microscopy for visualizing cell structures.
- Molecular biology methods to analyze genetic material.
- Biochemical assays to study metabolic processes.
These methods collectively provide a comprehensive view of plant cell functions, contributing to a deeper understanding of both normal and aberrant cellular processes. Incorporating data from various studies allows for synthesis of knowledge on how different cell parts collaborate to maintain plant health.
"Understanding the collaboration of cellular components is key to revealing the mysteries of plant biology."
Foreword to Plant Cells
Understanding plant cells is pivotal for grasping the complexities of plant biology. Plant cells form the basic unit of life within plants, enabling a variety of functions that are essential not only to plant health but also to broader ecological systems. This section provides an overview of what defines plant cells and their significance in biological studies.
Defining Plant Cells
Plant cells are eukaryotic cells, which means they contain a well-defined nucleus and organelles surrounded by membranes. Unique features distinguish plant cells from other cell types. One prominent characteristic is the presence of a rigid cell wall made primarily of cellulose. This structure provides physical support and protection, and it is also involved in regulating cell growth. In addition, plant cells are equipped with chloroplasts, which are vital for photosynthesis. These organelles enable plants to convert light energy into chemical energy, essential for growth and energy storage.
Furthermore, plant cells often contain large central vacuoles that serve as storage for nutrients, waste products, and help maintain turgor pressure, crucial for cell structure. The unique features of plant cells are essential for their role in supporting plant life and functioning within ecosystems.
Importance of Plant Cells in Biology
The importance of plant cells extends beyond their basic functions within a single organism. They play an indispensable role in generating oxygen and sustaining life on Earth. Through photosynthesis, plant cells produce oxygen that is crucial for the survival of aerobic organisms. This process also forms the foundation of the food chain, as plants serve as primary producers.
Moreover, studies of plant cell biology can lead to advances in various fields including agriculture, medicine, and environmental science. An understanding of how plant cells operate and interact can drive innovations in crop improvement and sustainable practices. Here are a few key points:
- Photosynthesis: Fundamental for energy creation in ecosystems.
- Oxygen production: Life-supporting process for aerobic organisms.
- Agricultural advancements: Enhancing crop yield and resistance through genetic research.
"The study of plant cells is not simply an academic exercise; it is an exploration of biological necessities that sustain life on our planet."
In summary, defining plant cells and recognizing their biological significance lays the foundation for deeper exploration into each cellular component. This knowledge serves as a springboard for further discussions on the various parts of plant cells and their functions.
Overview of Plant Cell Structure
The structure of plant cells is a vital topic within the study of plant biology. Understanding how these cells are organized and what components they consist of is crucial for recognizing their functions and contributions to the overall health and growth of plants. The architecture of plant cells is uniquely designed to enable them to perform essential tasks that support life. Notably, these tasks include photosynthesis, nutrient storage, and structural support, all of which are pivotal for plant survival and adaptability in various environments.
A careful examination of plant cell structure reveals distinct differences compared to animal cells. These differences not only underline the unique adaptations plants have evolved over time but also enable researchers and students to appreciate the complexity of plant biology. In this section, we will explore how plant cells differ from animal cells and identify the key components that define plant cells.
Comparison with Animal Cells
Plant cells and animal cells share basic cellular structures, but several critical differences set them apart. Key distinctions include:
- Cell Wall: One of the most prominent features of plant cells is the presence of a rigid cell wall. This structure maintains cell shape and provides additional protection against environmental stressors. In contrast, animal cells lack a cell wall, relying on a flexible plasma membrane for their structure.
- Chloroplasts: Only plant cells possess chloroplasts, which contain chlorophyll and are responsible for photosynthesis. This process converts sunlight into chemical energy, a function not available in animal cells.
- Vacuoles: Plant cells usually contain a large central vacuole, which stores water, nutrients, and waste products. This vacuole also helps maintain turgor pressure, ensuring the plant remains upright. Animal cells may have smaller vacuoles, but they do not serve the same pivotal role in maintaining cell structure.
- Shape: Lastly, plant cells often have a more regular, rectangular shape due to the rigid cell wall. Animal cells tend to have a more varied and flexible morphology, reflecting their different functions.
These differences highlight the adaptations that allow plants to thrive in their ecosystems. Recognizing these features can deepen the understanding of the roles plant cells play in larger biological systems.
Key Components of Plant Cells
The study of plant cell components is essential for grasping their physiological functions. Key components include:
- Cell Wall: Made primarily of cellulose, this structure not only provides protection but also contributes to growth by allowing flexibility.
- Chloroplasts: Within these organelles, light energy is converted into glucose, a crucial nutrient.
- Vacuoles: As previously mentioned, vacuoles are storage units, but they also play a role in creating internal pressure that supports cell structure.
- Mitochondria: Responsible for energy production, mitochondria are found in both plant and animal cells, showcasing the similarity in energy needs.
- Ribosomes: Essential for protein synthesis, ribosomes translate genetic information into functional proteins, crucial for cellular function.
- Endoplasmic Reticulum and Golgi Apparatus: These structures work together to synthesize and package proteins and lipids, ensuring they are modified and transported to where they are needed.
"Plant cells possess unique structures and functions that allow them to perform essential tasks critical for life on Earth."
Understanding these key components and their roles will provide insights into how plant cells operate and adapt to their environments. This knowledge serves as a building block for further explorations into plant biology, from genetics to ecology.
Cell Wall
The cell wall is a fundamental part of plant cells, providing structure and support. Within the context of this examination, understanding the cell wall helps highlight its significance in plant biology. It not only gives rigidity but also plays a pivotal role in various physiological functions. Moreover, the cell wall enables plants to adapt to their environment, making it an essential topic in cellular biology.
Composition and Structure
The cell wall is primarily composed of cellulose, a polysaccharide that forms strong microfibrils. These microfibrils create a network that provides structural integrity. Apart from cellulose, the cell wall also contains hemicellulose and pectin, which contribute to its flexibility and porosity.
Additionally, proteins and lignin may be present, especially in mature and woody plants, offering further strength. The structure can be categorized into three layers:
- Primary Cell Wall: Thin and flexible, serves as the first layer formed during cell growth.
- Secondary Cell Wall: Typically found in mature cells, it is thicker and more rigid, often containing lignin.
- Middle Lamella: A layer rich in pectin that acts as a glue, binding adjacent cells together.
Understanding these components helps in appreciating how the cell wall contributes to the overall functionality of plant cells.
Functions of the Cell Wall
The cell wall has multiple critical functions that extend beyond mere structural support.
- Protection: It acts as a first line of defense against pathogens and mechanical damage.
- Shape Maintenance: The rigidity provided by the cell wall helps maintain the shape of the plant cell, preventing it from collapsing under osmotic pressure.
- Transport Regulation: The cell wall helps in regulating the transport of materials into and out of the cell, contributing to homeostasis.
- Communication: Plasmodesmata, channels that traverse the cell wall, allow for the transport of signaling molecules and nutrients between cells, facilitating communication.
"The cell wall not only supports plant cells but also enhances their ability to interact with the environment".
Cell Membrane
The cell membrane is a crucial part of plant cells. It acts as a barrier that separates the interior of the cell from the external environment. This membrane is not just a protective layer; it is directly involved in regulating what enters and leaves the cell. Understanding the cell membrane helps to illustrate how plant cells maintain their integrity and functionality. This section will cover both the structure of the cell membrane and its role in homeostasis, highlighting its significance in cellular processes.
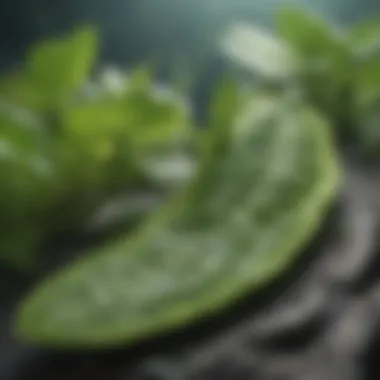
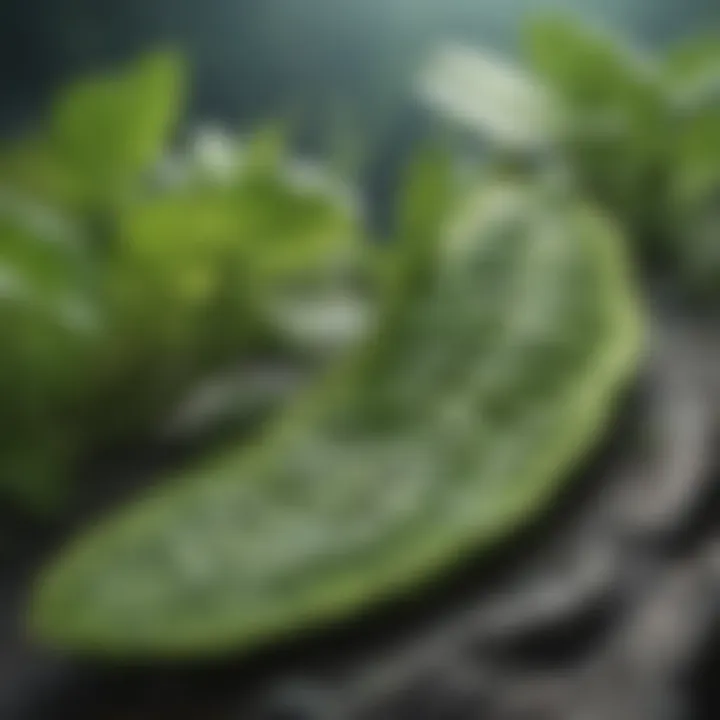
Structure of the Cell Membrane
The cell membrane is composed of a phospholipid bilayer with embedded proteins, cholesterol, and carbohydrates. Phospholipids consist of hydrophilic (water-attracting) heads and hydrophobic (water-repelling) tails. This unique arrangement allows the membrane to be semi-permeable, meaning it controls which substances can pass through.
- Phospholipid Bilayer: The bilayer structure is fundamental to the membrane's function. The hydrophilic heads face outward toward the water inside and outside the cell, while the hydrophobic tails point inward, creating a barrier to certain molecules.
- Proteins: Integral proteins span the membrane, serving various functions such as transport and signaling. Peripheral proteins are attached to the membrane's surface and often play roles in communication and support.
- Cholesterol: This component contributes to membrane fluidity, ensuring it remains flexible at varying temperatures.
- Carbohydrates: Often attached to proteins or lipids on the extracellular side, these molecules play a key role in cell recognition and signaling.
Understanding the structure of the cell membrane provides insight into its complex functionalities.
Role in Homeostasis
The cell membrane plays a vital role in maintaining homeostasis within plant cells. Homeostasis refers to the stable internal conditions that cells must maintain, even when external environments change. The membrane regulates this balance by selectively allowing substances to enter or exit.
Key functions include:
- Passive Transport: Small molecules and ions can pass through the membrane without energy use, moving from areas of higher concentration to lower concentration. This process is essential for nutrient absorption and waste removal.
- Active Transport: Some substances require energy to move against their concentration gradient. This process is crucial for maintaining necessary concentrations of ions and other molecules inside the cell.
- Signal Transduction: The cell membrane is involved in detecting signals from the environment. When these signals bind to membrane receptors, they trigger responses that help the cell adapt to changing conditions.
"The cell membrane is a complex, living entity that interacts with every aspect of the cell's environment."
Cytoplasm
Cytoplasm plays a crucial role in plant cells, existing as a gel-like substance that fills the interior. It is not just an inactive filler; rather, it is a dynamic environment where vital cellular processes occur. The cytoplasm houses organelles, enzymes, and various cytoplasmic components, all of which contribute to the overall function of a plant cell. This section outlines the description and composition of cytoplasm, followed by its significant functions in cellular activities.
Description and Composition
Cytoplasm consists of various elements, primarily water, salts, and organic molecules. Water makes up about 70-90% of the cytoplasm, providing a suitable medium for biochemical reactions. In addition to water, the cytoplasm contains
- Cytosol: the fluid portion of the cytoplasm, where metabolic reactions occur.
- Organelles: structures such as mitochondria, chloroplasts, and the endoplasmic reticulum, each with specialized functions.
- Cytoskeleton: a network of fibers aiding in structure, organization, and movement.
The composition is vital as it influences the cell's metabolic processes and the efficiency of enzyme action. Furthermore, varying concentrations of ions and molecules in the cytoplasm are critical for maintaining an appropriate environment for those processes.
Function in Cellular Processes
The cytoplasm is essential for multiple cellular functions. First, it provides a medium for the movement of cytoplasmic components, ensuring that organelles and molecules can efficiently interact. Its structure facilitates intracellular transport, which is necessary for proper organelle function and distribution.
Second, the cytoplasm facilitates metabolic processes, including glycolysis, where glucose is broken down for energy. It is also involved in protein synthesis, where ribosomes translate genetic information into functional proteins.
In addition to these functions, the cytoplasm plays a role in cellular signaling. Signals received from outside the cell are often transmitted through cytoplasmic pathways, coordinating the cell's response to environmental changes.
Thus, the cytoplasm is not merely a space within the cell but a complex environment crucial for sustaining life at the cellular level.
In summary, the cytoplasm acts as a vital component in the life of plant cells, influencing both their structure and function. Without the cytoplasm, essential cellular processes would be obstructed, leading to impaired plant health and functionality.
Nucleus
The nucleus holds paramount significance in the study of plant cell biology. It serves as the command center for cellular activities and plays a crucial role in genetic information management. Understanding the structure and functions of the nucleus is essential for comprehending how plants grow, develop, and respond to their environment.
Structure of the Nucleus
The plant cell nucleus is typically spherical and is surrounded by a double membrane known as the nuclear envelope. This envelope is perforated by nuclear pores, which regulate the passage of molecules in and out of the nucleus. The interior of the nucleus contains chromatin, a mix of DNA and proteins, which condenses to form chromosomes during cell division. The nucleolus, often visible within the nucleus, is the site of ribosomal RNA synthesis and ribosome assembly. The nucleus is larger in plant cells compared to animal cells, reflecting the complexity of the genetic material and functions it encompasses.
Genetic Information Management
The primary function of the nucleus is to store and manage genetic information. Each plant cell contains a complete set of DNA, which contains instructions for the cell's functions and traits. The process begins when a gene is expressed, leading to the synthesis of corresponding proteins that influence various cellular activities.
The nucleus orchestrates the transcription of DNA to messenger RNA (mRNA), which is essential for protein production. This mRNA then exits through the nuclear pores to reach ribosomes in the cytoplasm, where translation occurs.
Many factors influence genetic regulation within the nucleus. Environmental signals often dictate gene expression, allowing the plant to adapt to changing conditions. Additionally, the organization of DNA within chromatin can affect how readily genes are accessed for transcription, highlighting the nucleus's role in both specific and broad cellular responses to stimuli.
The nucleus is not merely a storage site for DNA; it is a dynamic hub of activity that ensures plants can respond effectively to their environment.
Chloroplasts
Chloroplasts are essential organelles found in plant cells and certain algae. They play a critical role in photosynthesis, which is the process by which light energy is converted into chemical energy stored in glucose. This function is not just vital for the plantβs energy but also for life on Earth as a whole since it contributes oxygen to the atmosphere and forms the basis of the food chain.
Photosynthesis Process
The photosynthesis process occurs in two main stages: the light-dependent reactions and the light-independent reactions, also known as the Calvin cycle.
- Light-Dependent Reactions:
- Calvin Cycle:
- These reactions require light energy, which is absorbed by chlorophyll, a pigment inside chloroplasts.
- Water molecules are split, releasing oxygen as a byproduct.
- Energy-carrying molecules, ATP and NADPH, are produced during this stage.
- This cycle does not directly require light. It utilizes ATP and NADPH generated in the first stage.
- Carbon dioxide is fixed into organic molecules, ultimately producing glucose, which serves as an energy source for the plant.
"Photosynthesis not only supports plant life but also sustains almost all ecosystems on Earth."
This intricate process illustrates the interconnectedness of life, highlighting the vital function of chloroplasts in sustaining ecosystems.
Chloroplast Structure
Chloroplasts have a unique structure that facilitates their function in photosynthesis. They are enclosed by a double membrane, with an intermembrane space between the two layers. Inside, chloroplasts contain several important structures, such as:
- Thylakoids:
- Stroma:
- Granum:
- These are flattened sacs stacked in structures known as granum. They house chlorophyll and other pigments necessary for capturing light energy.
- The fluid matrix surrounding the thylakoids, where the Calvin cycle occurs, contains enzymes, ribosomes, and the chloroplastβs own DNA, allowing for some independent protein synthesis.
- Composed of multiple thylakoids, it increases the surface area for light absorption, enhancing the efficiency of the photosynthesis process.
Understanding the structure of chloroplasts provides insights into how plants effectively harness light energy, contributing to their growth and development. Their specialized components are fine-tuned for the conversion of solar energy into chemical energy, which is fundamental not only to plant life but also to virtually every organism on the planet.
Mitochondria
Mitochondria are often referred to as the powerhouses of the cell. This designation comes from their crucial role in energy production. In plant cells, mitochondria function similarly to what they do in animal cells, converting nutrients into adenosine triphosphate (ATP) through a process called cellular respiration. Understanding mitochondria is essential, as they not only provide the energy needed for various cellular activities but also participate in metabolic processes that are fundamental to plant growth and development.
Role in Energy Production
Mitochondria are integral in the energy production process. They take in nutrients, mainly glucose, and convert them to ATP through the Krebs cycle and oxidative phosphorylation. This process involves a series of reactions that occur in the inner mitochondrial membrane and matrix. The ATP generated serves as a primary energy currency for the cell. It drives many cellular processes, including biosynthesis, cell division, and response to environmental stimuli.
Additionally, mitochondria help regulate metabolic pathways and maintain metabolic stability under varying conditions. They can adapt their function based on the energy demands of the cell, thus playing a central role in plant responses to stress, growth phases, and development. Their ability to produce reactive oxygen species (ROS) also places them at the center of signaling pathways that can influence the health of the plant.
Comparison with Chloroplasts
While both mitochondria and chloroplasts are involved in energy processes, they serve different functions. Mitochondria are responsible for cellular respiration, breaking down organic molecules to generate ATP. In contrast, chloroplasts are involved in photosynthesis, capturing light energy to convert carbon dioxide and water into glucose and oxygen. This distinction is crucial for understanding plant energy dynamics.
- Origin: Mitochondria are derived from ancient proteobacteria, while chloroplasts originated from cyanobacteria. This evolutionary background highlights their distinct roles in energy metabolism.
- Functionality: Chloroplasts capture solar energy, while mitochondria extract energy from organic compounds. Thus, chloroplasts are primarily active during daylight, while mitochondria function continuously.
- Energy Flow: Both organelles are linked in energy transfer; the glucose produced in chloroplasts feeds into mitochondria for respiration.
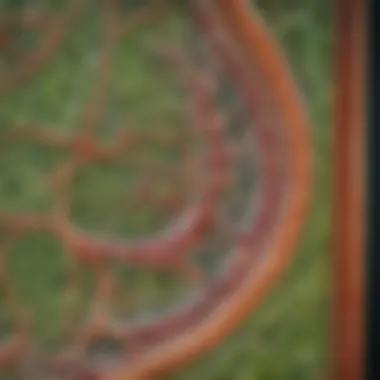
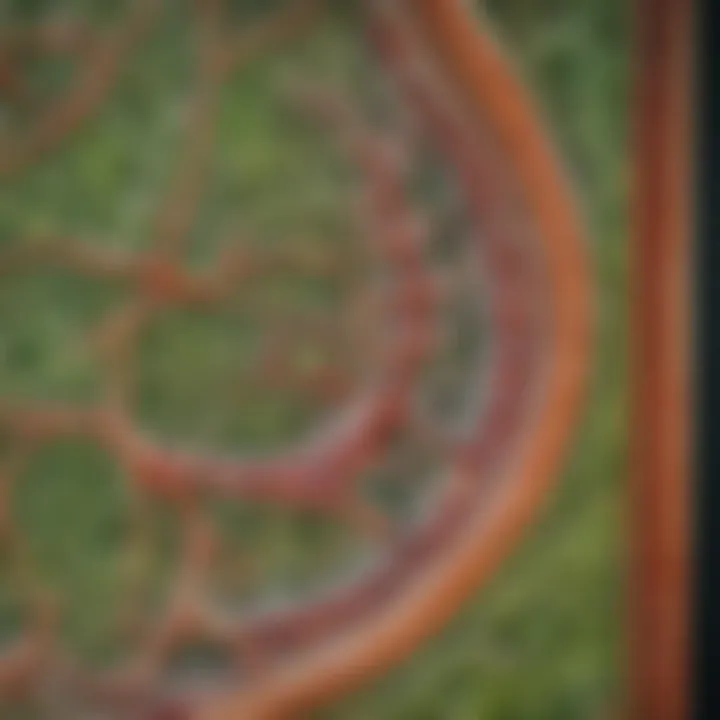
Endoplasmic Reticulum
The Endoplasmic Reticulum (ER) is a crucial organelle in plant cells, playing a vital role in the synthesis, folding, modification, and transport of proteins and lipids. This extensive network of membranes is not just a structural component; it is essential for cellular function and overall plant health. Understanding the specifics of the ER enhances our grasp of plant biology, as many cellular processes rely on the effective operation of this organelle.
Rough vs. Smooth ER
The Endoplasmic Reticulum can be divided into two distinct types: Rough ER and Smooth ER. The Rough ER is characterized by its ribosome-studded surface, which gives it a rough appearance. This design is significant because it is directly involved in protein synthesis and processing. Proteins synthesized here often enter the ER lumen for further modification before being transported to various destinations within or outside the cell.
In contrast, the Smooth ER lacks these ribosomes, giving it a smooth texture. It is associated primarily with lipid synthesis and metabolism. Additionally, the Smooth ER plays a role in detoxifying certain chemicals and storing calcium ions, which are critical in various signaling pathways. By clearly distinguishing between these two types, we can appreciate how specialized the functions of the Endoplasmic Reticulum are in maintaining cellular balance and facilitating essential biochemical processes.
Functions of the Endoplasmic Reticulum
The functions of the Endoplasmic Reticulum extend beyond mere protein and lipid synthesis. Key responsibilities include:
- Protein Folding and Modification: After synthesis in the Rough ER, proteins undergo folding and post-translational modifications. Chaperones present in the lumen assist in correct folding, ensuring functionality.
- Lipid Synthesis: The Smooth ER is crucial for synthesizing phospholipids and sterols that form cellular membranes, impacting the overall cell structure.
- Detoxification: This organelle can modify and neutralize harmful substances, which is particularly important for maintaining cellular health under stress conditions.
- Calcium Storage: The ER acts as a reservoir for calcium ions, which are essential for various cellular activities, including muscle contraction and cell signaling.
The Endoplasmic Reticulum is central to plant cells, ensuring that proteins and lipids are produced correctly, contributing fundamentally to maintaining cell health and responding to environmental changes.
In summary, the Endoplasmic Reticulum in plant cells plays a multifaceted role that is essential for the growth and sustainability of the plant. Its distinct sections, Rough and Smooth ER, showcase how specialization in cellular functions is crucial for overall plant vitality.
Golgi Apparatus
The Golgi apparatus plays a critical role in plant cells. It is often referred to as the cell's post office due to its involvement in modifying, sorting, and packaging proteins and lipids for secretion or delivery to other organelles. Understanding the structure and function of the Golgi apparatus is vital because it ensures cells produce and distribute the necessary molecules for growth and maintenance. This section explains how its structure supports its function and digs into the significance of protein modification and transport within the cellular context.
Structure and Function
The structure of the Golgi apparatus consists of stacked, flattened membrane-bound sacs called cisternae. These cisternae are arranged in a series, resembling a stack of pancakes. The Golgi apparatus typically features a distinct polarity, with a receiving side known as the cis face and an opposite side called the trans face. Proteins synthesized in the endoplasmic reticulum (ER) arrive at the Golgiβs cis face, where they undergo post-translational modifications.
Here's a simple explanation of the processes inside:
- Protein Arrival: Newly formed proteins and lipids from the rough endoplasmic reticulum enter the Golgi.
- Modification: Enzymes present in the Golgi apparatus modify these proteins, often through glycosylation.
- Sorting: Modified proteins are sorted for transport to their specific destinations.
- Secretion: Finally, proteins are packed into vesicles that bud off from the trans face.
These features of the Golgi apparatus enable it to function efficiently in processing and distributing materials necessary for plant cell function.
Protein Modification and Transport
Protein modification within the Golgi apparatus is crucial for the functionality of these molecules. The alterations can include the addition of carbohydrate moieties, which are essential for the proper folding and stability of proteins. Additionally, modifications can determine the proteins' eventual location within the plant cell or their secretion outside the cell.
Key aspects of protein transport include:
- Glycosylation: Addition of sugars helps in the formation of glycoproteins, essential for cell recognition and signaling.
- Vesicle Budding: Proteins are encapsulated into vesicles that target the appropriate destinations.
- Targeting Signals: Each protein has specific signals that direct it to its next location, whether that's the plasma membrane, other organelles, or secretion outside the cell.
"The Golgi apparatus is the hub of protein sorting and modification, essential for cellular function and integrity, particularly in plants where cell communication is vital for survival."
The Golgi apparatus exemplifies how plant cells manage the complexity of protein trafficking and the importance of precise molecular interactions. Its structural and functional characteristics contribute significantly to plant growth, adaptability, and homeostasis.
Vacuoles
Vacuoles are essential organelles in plant cells, playing a key role in maintaining cellular function and overall plant health. They are particularly important for storage and structural integrity, serving various functions that are crucial for the plant's growth and survival. Understanding vacuoles helps shed light on how plants manage nutrients and waste products, as well as how they respond to environmental changes.
Types of Vacuoles
Vacuoles can be categorized primarily into two types: central vacuoles and contractile vacuoles.
- Central Vacuoles: These are large and typically occupy a significant portion of the plant cell's interior. Central vacuoles are filled with a solution of water, ions, and other molecules. They are crucial for maintaining turgor pressure, which helps keep the plant upright and resistant to wilting.
- Contractile Vacuoles: These are primarily found in some unicellular organisms and in certain plant cells. Their main function is to expel excess water from the cell. This helps maintain osmotic balance, which is vital for cellular health in aquatic environments.
Both types of vacuoles have distinct roles but share the common function of helping in cellular homeostasis by regulating the internal environment of the cell.
Function in Storage and Support
Vacuoles serve multiple functions in storage and providing structural support within plant cells. They can store various substances, including nutrients, waste products, and secondary metabolites. These metabolites can include pigments, alkaloids, and tannins, which play a role in defense mechanisms against herbivores and pathogens.
"Vacuoles are the storage centers of the plant cell, significantly influencing plant metabolism and health."
In terms of support, the pressure exerted by the liquid within the central vacuole creates turgor pressure. This pressure is vital for maintaining the rigidity of plant cells and, consequently, the entire plant. A well-hydrated vacuole ensures that the plant remains upright, which is important for maximizing light absorption and promoting photosynthesis.
In summary, vacuoles are more than just storage units. They are integral to a plant's ability to adapt to its environment and are crucial for its growth and reproduction. Understanding their structure, types, and functions can provide insight into broader biological processes involving plant cells.
Ribosomes
Ribosomes are essential organelles within plant cells, playing a pivotal role in the complex processes of protein synthesis. These microscopic structures are sometimes referred to as the "protein factories" of the cell, highlighting their critical function in translating genetic information into functional proteins. Without ribosomes, plant cells would lack the means to produce the proteins necessary for their growth and development, which includes enzymes, hormones, and structural components vital for cellular integrity.
Protein Synthesis
Ribosomes are responsible for assembling amino acids into polypeptides, which fold into proteins. This process begins with the messenger RNA (mRNA), transcribed from DNA, carrying the genetic instructions from the nucleus to the ribosome. As the ribosome reads the mRNA sequence, transfer RNA (tRNA) molecules bring the appropriate amino acids from the cytoplasm. Each tRNA corresponds to a specific codon on the mRNA, ensuring that the correct amino acid is incorporated into the growing polypeptide chain.
The ribosome moves along the mRNA, linking the amino acids in the proper order. This activity allows for the formation of primary, secondary, and tertiary protein structures. Once synthesized, the new proteins can be used for a variety of functions such as catalyzing metabolic reactions, providing structural support, and regulating cellular processes.
Protein synthesis is fundamental for the maintenance of cellular functions and overall plant health.
Location within the Cell
Ribosomes can be found in several locations throughout the plant cell, most notably in two primary forms: free ribosomes and membrane-bound ribosomes. Free ribosomes float freely within the cytoplasm and synthesize proteins destined for use within the cytoplasm itself. In contrast, membrane-bound ribosomes are attached to the outer membrane of the endoplasmic reticulum, forming what is known as the rough endoplasmic reticulum. These ribosomes typically synthesize proteins that are either secreted from the cell or incorporated into cellular membranes.
Additionally, ribosomes are also present within chloroplasts and mitochondria. This unique presence underscores the endosymbiotic theory, which proposes that these organelles descended from free-living prokaryotic cells. The ribosomes found in these organelles resemble those of bacteria and are involved in synthesizing proteins essential for the organelles' functions in energy production and photosynthesis.
In summary, ribosomes are indispensable for the overall functionality of plant cells, facilitating the vital process of protein synthesis in various cellular compartments. Their ability to produce the necessary proteins ensures that not only are fundamental cellular processes carried out efficiently, but also that the plant can respond to environmental changes and stimuli.
Peroxisomes
Peroxisomes are small, membrane-bound organelles present in the cells of plants. These structures are crucial for various cellular processes, making them significant in the overall context of plant cell function. Their importance lies mainly in the pathways they facilitate in metabolism, specifically in lipid metabolism and the breakdown of harmful reactive oxygen species.
Function in Metabolism
Peroxisomes play a vital role in cellular metabolism. They contain enzymes that are essential for the Γ-oxidation of fatty acids. This process is critical because it helps break down long-chain fatty acids into shorter units, which can be further utilized for energy production or converted into other essential compounds. This function is especially important for seeds, which rely on stored fats as a primary energy source during germination and early growth.
Additionally, peroxisomes are involved in the breakdown of various metabolic byproducts. They help in converting hydrogen peroxide, a byproduct of metabolic reactions that can be harmful in excess, into water and oxygen. This detoxifying role is essential for maintaining cellular health, preventing damage from oxidative stress.
Oxidative Reactions
Oxidative reactions are a key aspect of peroxisomal function. In plants, peroxisomes contain enzymes such as catalase and various oxidases that facilitate these reactions. The enzyme catalase converts hydrogen peroxide into water and oxygen, effectively neutralizing potential cellular toxins. This reaction is vital in protecting cells from oxidative damage, which can arise from both normal metabolic activities and environmental stressors like UV radiation or pollutants.
Moreover, peroxisomes are responsible for the oxidation of other substrates, enabling the production of important metabolic intermediates. For example, they participate in the metabolism of purines and the synthesis of hormones like jasmonates, which are involved in stress responses and plant defense mechanisms. Thus, the correct functioning of peroxisomes is directly linked to the plant's resilience and adaptability to its environment.
"Peroxisomes are not just waste management units; they are active participants in complex metabolic networks within the plant cell."
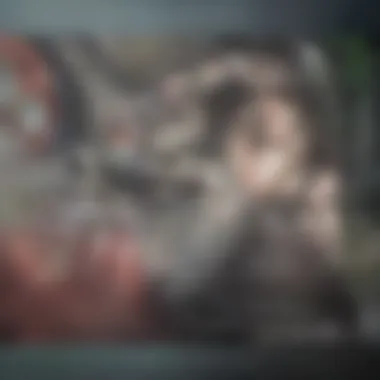
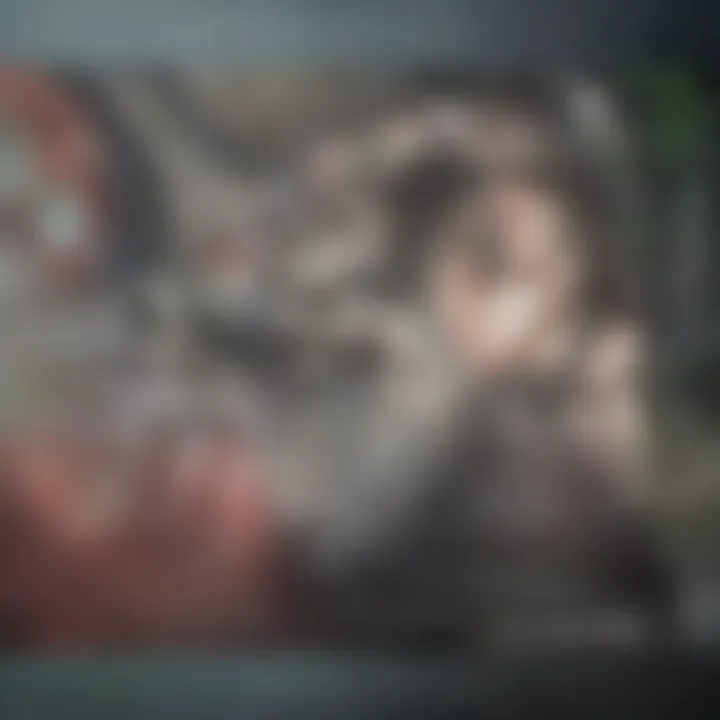
In summary, peroxisomes are essential organelles with multifunctional roles in plant cell metabolism. From breaking down fatty acids to detoxifying harmful substances, these structures are vital for maintaining the balance and functionality of plant cells.
Plasma Membrane
The plasma membrane is a crucial element of plant cells, playing a pivotal role in maintaining cellular integrity and facilitating communication between the cell and its external environment. It acts as a selective barrier, regulating the movement of substances in and out of the cell. This function is essential for the overall health and functionality of the plant cell. Notably, the plasma membrane not only protects the cell's internal structures but also participates in various metabolic processes.
Semi-Permeability
The semi-permeable nature of the plasma membrane allows certain molecules to pass through while blocking others. This selective permeability is fundamental to preserving the unique cytoplasmic composition of the cell. Molecules such as water, ions, and small uncharged molecules can freely diffuse across the membrane, whereas larger or charged molecules often require specific transport mechanisms.
- Types of Transport:
- Passive Transport: such as diffusion and osmosis, does not require energy and occurs along the concentration gradient.
- Active Transport: requires energy, often in the form of ATP, to move substances against their concentration gradient.
This balance of movement ensures that essential nutrients enter the cell and waste products are expelled effectively.
Cell Signaling
Cell signaling is another significant function of the plasma membrane. It contains various receptors that detect external signals. These receptors initiate cellular responses that are vital for plant growth and adaptation. For instance, when a plant encounters stress, such as drought or pathogen attack, signaling pathways are activated through the plasma membrane, leading to changes in gene expression and other cellular activities.
"The plasma membrane acts not just as a barrier, but also as a communicator, allowing the plant to respond to environmental changes rapidly."
- Key Signaling Molecules:
- Hormones like auxins, which regulate growth and response to light.
- Reactive oxygen species that can signal stress responses.
Understanding how the plasma membrane functions aids in comprehending plant biology and the various mechanisms that ensure the survival and adaptation of plant cells.
Cytoskeleton
The cytoskeleton is an integral part of plant cell architecture. It provides structural support and plays a critical role in the cell's functionality. Understanding the cytoskeleton is vital, as it not only aids in maintaining the cell's shape but also facilitates movement within the cell and during cell division. Without a well-functioning cytoskeleton, a plant cell would struggle to maintain its integrity and perform essential functions. Thus, the significance of the cytoskeleton cannot be understated in the context of plant cellular biology.
Components of the Cytoskeleton
The cytoskeleton is composed of three main types of filaments: microtubules, microfilaments, and intermediate filaments. Each component has distinct properties and functions:
- Microtubules: These are hollow tubes made from tubulin proteins. They are crucial in maintaining cell shape and are involved in intracellular transport. Additionally, microtubules play a vital role in cell division by forming the mitotic spindle.
- Microfilaments: Composed of actin, these fibers are involved in muscle contraction and cell motility. In plant cells, microfilaments help in the movement of organelles and other cellular components, as well as providing structural support.
- Intermediate Filaments: These provide tensile strength to the cell and help maintain its shape. They are more stable and less dynamic compared to microtubules and microfilaments.
The combination of these components creates a dynamic structure that is adaptable to the needs of the cell.
Role in Cell Shape and Movement
The cytoskeleton is essential for determining the shape of a plant cell. It establishes a rigid framework that protects the cell's integrity while allowing necessary flexibility. This flexibility is vital for growth, as it allows the cell to adapt to changing environmental conditions.
In terms of movement, the cytoskeleton facilitates the transport of organelles and vesicles within the cell. This transport is critical for nutrient distribution and waste removal, directly impacting cellular health. Additionally, during cell division, the cytoskeleton directs the separation of chromosomes, ensuring that each daughter cell receives the correct genetic material.
"The cytoskeleton acts as both a scaffold and a transport system, crucial for maintaining cellular organization and function."
Organelles and Their Interactions
In the realm of plant cell biology, organelles play a pivotal role not just within their structures but in how they function together. This section emphasizes the importance of understanding the dynamic interactions that occur between organelles. By elucidating these connections, we gain deeper insights into how plant cells maintain their integrity and health. Effective collaboration among these organelles leads to optimized functions, ensuring that metabolic processes and cellular responses are conducted efficiently.
Collaboration between Organelles
Collaboration between organelles is fundamental to the productivity of plant cells. Each organelle has a specific responsibility, yet their interdependence is what allows cells to perform complex tasks. The nucleus, for example, serves as the control center, housing genetic material and coordinating cellular activities by signaling other organelles. Ribosomes depend on the nucleus for instructions on protein synthesis. The endoplasmic reticulum, both smooth and rough, acts as a site for translating these instructions into functioning proteins.
The close relationship between chloroplasts and mitochondria highlights another aspect of collaboration. Chloroplasts perform photosynthesis, converting sunlight into glucose, which is a primary energy source. Mitochondria then execute cellular respiration, breaking down glucose to produce ATP, the energy currency of cells. This seamless transfer of energy through organelle interaction underscores the efficiency of plant metabolism.
"The coordination among organelles exemplifies nature's ingenuity in optimizing cellular processes and fulfilling the requirements of life."
Furthermore, peroxisomes help in detoxification processes that may arise from the metabolic activities of other organelles. They collaborate by processing hydrogen peroxide, a byproduct of metabolism, and converting it into water and oxygen. This ensures that cells maintain homeostasis and protect themselves from oxidative damage.
Importance of Organelle Communication
Communication between organelles is as crucial as their collaboration. Organelles must exchange signals and materials to function coherently. For instance, the Golgi apparatus receives proteins from the endoplasmic reticulum, modifies them, and then dispatches them to various parts of the cell or to the exterior. This process exemplifies how organelles coordinate their actions through signaling pathways.
Moreover, the plasma membrane plays an essential role in organelle communication. It regulates what enters and exits the cell, ensuring that essential molecules are available when needed. This selective permeability facilitates interactions, allowing for responses to environmental changes.
The integration of signals within the cell is vital in creating an adaptable and responsive organism. This characteristic is especially important for plants as they face variable environmental conditions. By maintaining effective organelle communication, cells can rapidly adjust their internal processes, ultimately ensuring the plant can adapt and thrive.
Understanding organelle interactions and their communication lays the groundwork for appreciating plant cell functionality. This knowledge opens avenues for further study in areas such as genetics, agriculture, and environmental science.
Plant Cell Division
Plant cell division is a critical process in the lifecycle of plants that allows for growth, repair, and reproduction. A deeper understanding of this topic reveals various elements, benefits, and considerations that can impact the health of entire plant populations. Cell division in plants encompasses not only the replication of genetic material but also the intricate mechanisms that ensure each new cell fulfills its specific role while contributing to the overall functioning of the organism.
Mitosis in Plant Cells
Mitosis is the primary method of cell division in plant cells. It is responsible for producing two daughter cells that are genetically identical to the parent cell. Mitosis is subdivided into phases: prophase, metaphase, anaphase, and telophase, which work systematically to ensure proper distribution of genetic material. The process starts with chromatin condensing into visible chromosomes, followed by the alignment of these chromosomes at the cell's equator.
During anaphase, the sister chromatids are separated and pulled toward opposite poles of the cell. Finally, in telophase, the chromosomes de-condense back into chromatin, and the nuclear envelope reforms around each set of chromosomes. Cytokinesis follows, leading to the formation of two distinct daughter cells. Unlike animal cells, where a cleavage furrow forms, plant cells undergo cytokinesis through the formation of a new structure called the cell plate, which later develops into the cell wall separating the two daughter cells.
The significance of mitosis in plant cells cannot be overstated. This process is fundamental for growth and the rejuvenation of tissues damaged by factors like disease or environmental stresses. For instance, when a plant is pruned, the resultant wounds must heal, which will involve new cells generated through mitosis. Understanding this process also provides insight into how plants adapt and flourish in a wide variety of environments.
Role of Organelles in Cell Division
Organelles play a vital role in the successful completion of mitosis. Key players include the nucleus, which houses the genetic material, and the endoplasmic reticulum, which is involved in the synthesis of proteins necessary for cell division. Moreover, the Golgi apparatus is essential for the packaging and distribution of enzymes and proteins that aid the process.
Additionally, microtubules, part of the cytoskeleton, are responsible for forming the spindle fibers that facilitate the movement of chromatids during mitosis. The proper functioning of these organelles is crucial for accurate cell division and maintaining cellular integrity. The failure of any one of these components can lead to errors in mitosis, potentially resulting in cell malfunction or improper tissue development.
In summary, the coordination between these organelles guarantees that plant cells divide correctly, allowing for sustained growth and overall plant health. The study of plant cell division integrates key knowledge from cellular biology while highlighting the complex interplay among various cellular components.
Culmination
In the exploration of plant cell parts and their functions, it becomes clear that understanding these elements is crucial. Plant cells are the fundamental unit of life in plants, and each organelle plays a distinct role that contributes to the overall health and functionality of the plant organism. The knowledge gained from studying these organelles enriches our comprehension of basic biological processes and emphasizes their interconnectedness.
Summary of Key Functions
Plant cells possess several key functions carried out by their organelles:
- Cell Wall: Provides structural support and protection against mechanical stress and pathogens.
- Chloroplasts: Facilitate photosynthesis, converting light energy into chemical energy.
- Mitochondria: Produce ATP through cellular respiration, essential for energy transfer.
- Vacuoles: Store nutrients, waste products, and help maintain turgor pressure for structural integrity.
- Endoplasmic Reticulum and Golgi Apparatus: Involved in the synthesis and transportation of proteins and lipids.
- Nucleus: Houses genetic material and coordinates cellular activities, including growth and reproduction.
Each of these components is vital for maintaining homeostasis within the plant cell and ultimately the plant as a whole.
Implications for Plant Biology Studies
The implications of understanding plant cell functions extend beyond mere academic interest. Here are some considerations:
- Agricultural Advancements: Insights into plant cell functions can lead to innovations in crop management and improvement of yield.
- Environmental Research: Studying how plants respond at the cellular level to environmental changes helps in developing strategies for climate adaptation.
- Biotechnology Applications: Knowledge of cellular processes can inform genetic engineering approaches to enhance desirable traits in plants.
Research into plant cellular structures not only contributes to our fundamental biological knowledge but also has practical applications that affect food security, ecosystem management, and sustainable practices in agriculture.
Understanding the functions of plant cell parts is not just an academic endeavor; it is a step toward solving real-world problems in agriculture and environmental sustainability.