Understanding Qubit Computing: Foundations and Future
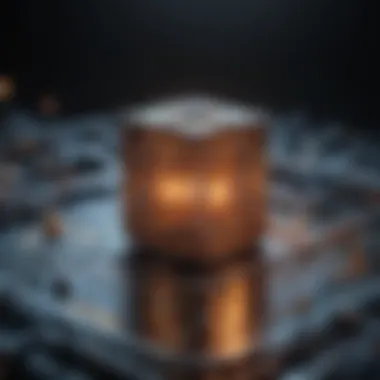
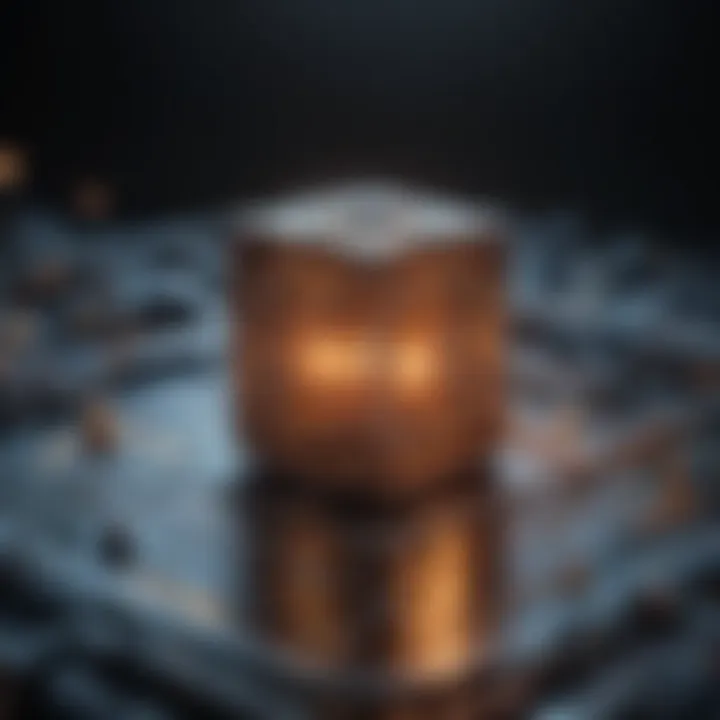
Intro
As we march into an era defined by advanced computational techniques, understanding the fundamental shift brought about by qubit computing is essential. At the heart of quantum technology, qubits offer new avenues of problem-solving and information processing beyond the capabilities of traditional bits. In this exploration, we will dissect the core concepts driving qubit computing, its implications across sectors, and the recent advancements that have shaped this exciting field.
Recent Advances
Developments in qubit technology have accelerated at a breakneck pace, mirroring the rapid advancements in materials science and quantum physics. This section will illuminate the latest discoveries and technological innovations that bolster our understanding and application of qubits.
Latest Discoveries
Recent breakthroughs in the manipulation and control of qubits have deepened our grasp of quantum behavior. For instance, scientists at Google recently reported the successful demonstration of quantum supremacy, where a quantum computer performed a specific task far quicker than any classical computer could. Such achievements highlight the potential for qubit systems to handle complex computations in a fraction of the time, providing a glimpse of their transformative power.
Additionally, researchers have pinpointed improved techniques for qubit error correction, addressing a significant hurdle faced in real-world applications. Studies have shown that utilizing a combination of topological qubits could lead to more robust systems less susceptible to environmental disturbances.
Technological Innovations
Beyond theoretical advancements, tangible technological innovations are emerging, making qubit computing more accessible. One notable example is the development of superconducting qubits, which leverage low temperatures to maintain quantum states effectively. Companies like IBM have pioneered architectures that integrate these units within modular systems, catering to diverse computational tasks.
Moreover, platforms such as Rigetti Computing's quantum cloud services allow users to engage with qubit systems remotely. This opens doors for researchers, educators, and professionals to experiment with quantum algorithms without needing specialized hardware.
Methodology
To delve deeper into the intricacies of qubit computing, it is important to understand the methodologies underpinning research in this area. The following sections outline a framework that researchers employ to navigate this complex field.
Research Design
The design of research projects within qubit computing typically revolves around a blend of experimental and theoretical approaches. Scientists combine simulation techniques with hardware experimentation to verify theoretical models and develop new applications. This dual approach is vital to gaining insights that are both practically applicable and firmly rooted in established physics.
Data Collection Techniques
Data collection methods in quantum computing research vary widely. Researchers employ:
- Experimental Data: This involves direct measurement of qubit operations, including fidelity and coherence times, using advanced microscopy techniques.
- Simulation Data: Sophisticated algorithms simulate quantum behaviors, enabling scientists to foresee potential outcomes before actual hardware tests.
- Collaborative Data: Sharing findings through platforms like arXiv.org fosters community engagement and accelerates collective understanding in this rapidly evolving field.
In summary, the rise of qubit computing represents a monumental leap in our computing capabilities. By combining the latest discoveries with innovative technologies, researchers are crafting a future where quantum computing can solve problems previously deemed insurmountable.
As we journey through this exploration, keeping an eye on both the foundational principles and the applied methods in qubit technology will reveal not just possibilities, but a pathway to redefine the landscape of computation.
Intro to Qubit Computing
The world of computing is undergoing a seismic shift, a transformation that promises not just speed, but a fundamentally new way of processing information. Qubit computing is the linchpin of this revolution, standing distinct from the traditional binary systems that have defined computing for decades. Understanding qubits is not merely for those in a lab coat or behind a computer screen; it impacts diverse fields ranging from cryptography to material science. By embracing the intricacies of this technology, we can unlock potentials that were once relegated to the realm of science fiction.
Definition of a Qubit
A qubit, or quantum bit, represents the smallest unit of quantum information. Unlike a classical bit, which is confined to a state of either 0 or 1, a qubit can exist in a state called superposition. This means it can hold multiple values simultaneously, broadening its functional repertoire exponentially. To put it simply, if you’ve ever flipped a coin, you’re familiar with this concept; when a coin spins in the air, it's not decisively heads or tails but rather has the potential to be both until it lands.
In technical jargon, if we denote the states of a qubit as |0⟩ and |1⟩, then a qubit can represent:
where α and β are complex numbers that describe probability amplitudes. The squares of the magnitudes of these coefficients indicate the likelihood of finding the qubit in the respective states during measurement.
Historical Context
The journey towards qubit computing doesn’t emerge from a vacuum but is rooted in centuries of scientific inquiry and technological innovation. The seeds for this quantum revolution were planted in the early 20th century through the pioneering work of physicists like Max Planck and Albert Einstein, who began unraveling the mysteries of quantum mechanics. The advent of quantum theory laid down the roadmap for further explorations into the microcosm of atomic and subatomic particles.
Fast forward to the 1980s, when figures like David Deutsch began to explore the concept of quantum computation. His seminal work paved the way for what we now know as the quantum computing framework, incorporating principles of superposition and entanglement. In the years that followed, various implementations of qubits emerged, making it clear that this wasn’t merely theoretical—quantum machines were on the horizon.
Throughout the 1990s and early 2000s, notable advances were made, including Peter Shor's algorithm, which showcased the potential of qubit computing to outperform classical systems in specific tasks, particularly in factoring large numbers, a fundamental principle in cybersecurity. As the stakes grew, so too did interest from governments and industry players, marking a pivotal point in the evolution of this technology.
In essence, the narrative of qubit computing is one of collaboration, innovation, and the relentless pursuit of knowledge. Today, as researchers and engineers stand at the threshold of the next generation of computing, they leverage not only theoretical insights but also practical experiences gleaned from over a century's worth of scientific endeavor.
Understanding qubit computing is crucial in contextualizing its transformative impact across various applications, setting the stage for the discussions that will unfold in the subsequent sections.
Fundamental Principles of Quantum Mechanics
Understanding the fundamental principles of quantum mechanics is crucial for grasping the underpinnings of qubit computing. These principles paint a picture of how quantum systems operate, distinguishing them sharply from classical systems. Within this realm, concepts like superposition and entanglement emerge as foundational elements that unlock new levels of computational potential, allowing for processing capabilities that were once thought to be purely theoretical. By delving into these principles, we uncover the intricate behaviors that qubits exhibit, providing a solid framework upon which quantum computing technologies are built.
Superposition
Superposition serves as a pillar of quantum mechanics. It allows a qubit to exist in multiple states at once, which can drastically enhance processing capabilities.
Mathematical Representation
The mathematical representation of superposition often employs complex numbers and linear algebra. For instance, a qubit's state can be expressed as a linear combination of the basis states |0⟩ and |1⟩:
$$ \mid \psi \rangle = \alpha \mid 0 \rangle + \beta \mid 1 \rangle $$
where ( \alpha ) and ( \beta ) are complex coefficients that define the probability amplitude for each state. This representation is not just academically interesting; it provides concrete tools for quantum algorithms, showcasing the power of superposition in facilitating multiple computations simultaneously. This unique feature significantly boosts the efficiency of processing tasks.
However, the use of complex numbers can introduce confusion for those new to the field, making it both an advantage and a disadvantage depending on the context of its application.
Implications of Superposition
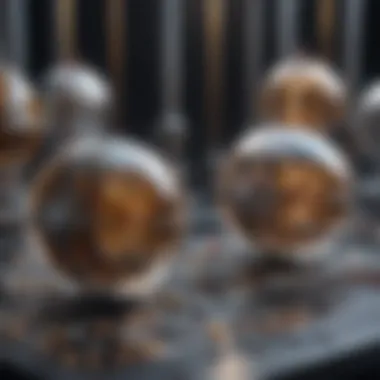
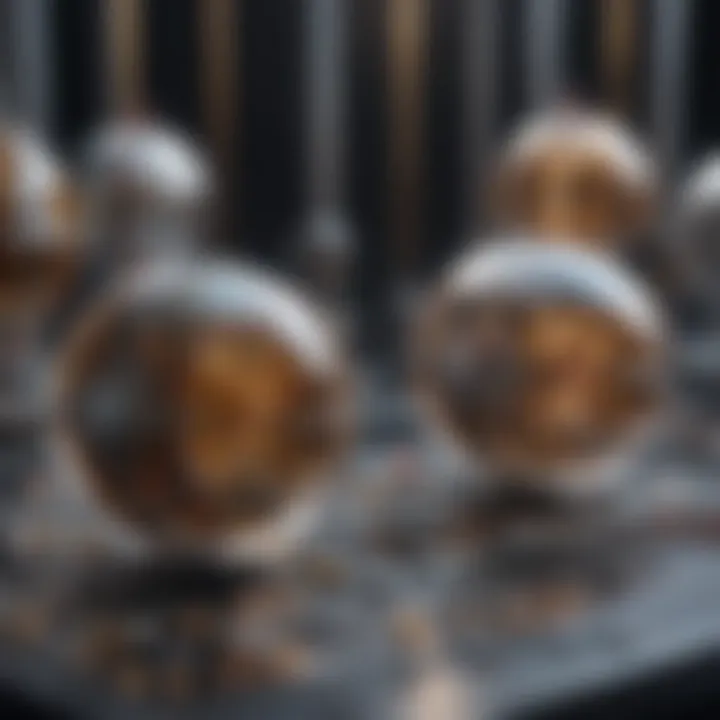
The implications of superposition in computing are profound. One of the most notable aspects is the ability to perform computations on multiple inputs simultaneously. Because qubits can represent more than just 0 or 1, they open possibilities for algorithms that could outpace classical counterparts in specific areas like optimization and search problems.
This characteristic not only showcases the strength of quantum systems but also highlights its potential to unravel solutions to complex issues unseen with traditional computing methods. However, this comes with a caveat; the intricate behavior of superposed states can make actual computation more challenging. Often, the art lies in collapsing these states into a definitive outcome without losing information about the computation performed.
Entanglement
Entanglement is one of the most fascinating phenomena in quantum mechanics. It describes a condition in which two or more qubits become interconnected to the degree that the state of one qubit instantaneously influences the state of the other, regardless of the distance separating them.
Definition and Characteristics
The definition of entanglement can seem head-scratching at first. When qubits become entangled, the entire system's joint state cannot be factored into individual states. This means their behaviors are interlinked, which can be illustrated mathematically as:
$$ \mid \Psi \rangle = 1/\sqrt2(\mid 00 \rangle + \mid 11 \rangle) $$
This equation implies that measuring one qubit instantly gives information about the other, demonstrating a strong correlation between them. This characteristic is paramount to the efficiency attributed to quantum information transmission and processing, making it a vital feature in quantum algorithms, error correction, and cryptography.
While the advantages of entanglement are compelling, the actual entanglement process can be complex and sensitive to environmental interference. Researchers are investigating methods to bolster entangled states’ stability, paving the way for practical implementations.
Role in Quantum Computing
The role of entanglement in quantum computing cannot be understated. It enhances computational power significantly by enabling qubits to share information and work collaboratively far more effectively than classical bits. The entangled states can be exploited in various quantum computing protocols, such as quantum teleportation and superdense coding, amplifying the system’s capability.
This collaboration among qubits leads to increased information density and speed in processing. In scenarios such as quantum algorithms for factorizing large numbers, entanglement contributes mightily to achieving results that were previously thought unattainable. However, entanglement's delicate nature necessitates rigorous control measures to maintain effectiveness.
"Quantum computing is not simply about speed; it’s about a new way of processing information without limitations imposed by classical constraints."
As quantum technology evolves, mastering these fundamental principles will be essential for harnessing the full power of qubit computing and its transformative potential across various sectors.
Traditional Computing vs. Qubit Computing
When engaging with the world of computing, it's crucial to draw a line between traditional computing systems and the innovative qubit computing paradigm. Understanding these differences not only elucidates their respective strengths and limitations but also sets the stage for discerning their potential applications across various sectors.
Classical Bits Explained
At the heart of traditional computing lies the classical bit. A classical bit is the fundamental unit of information, represented by either a 0 or a 1. This binary system underpins all processing in classical computers, from simple calculations to complex operations. Each bit acts independently; when a computation is performed, classical bits undergo straightforward transformations according to binary logic.
In layman's terms, think of classical bits as light switches that can either be off (0) or on (1). This on-off simplicity allows traditional computers to perform reliable calculations, yet, it also limits their computational capacity.
Key Characteristics of Classical Bits:
- Deterministic Behavior: Each bit is easily predictable based on the input and operations.
- Defined States: A classical bit's value can only be either 0 or 1.
- Binary Logic Operations: Classical algorithms rely on Boolean operations to process bits.
Comparative Analysis of Computational Power
Transitioning from classical to qubit computing reflects a fundamental shift in computational power. One cannot simply state that qubit computing is superior, but the differences in processing power and capabilities are paramount to the discussion.
Complex Problem Solving
Complex problem solving stands out as a significant area of computational power. Classical computers can tackle complicated problems, however, their approach is linear. In contrast, qubit computing harnesses quantum capabilities like superposition and entanglement to explore many solutions simultaneously.
This makes quantum computers particularly effective for problems like factoring large integers – a crucial task for cryptography. Their ability to consider multiple possibilities at once notably decreases the time needed to find solutions. The unique feature here lies in their parallelism, presenting a edge in scenarios where the number of potential outcomes is vast.
- Advantages:
- Disadvantages:
- Speed in solving problems that classical computers struggle with.
- Enhanced efficiency by executing multiple calculations at the same time.
- Complex to implement and require specialized knowledge to program effectively.
Data Processing Speed
Data processing speed is another vital aspect that merits examination. In traditional computing, speed is linked to the ability to execute sequential tasks swiftly, where website loading times and application responsiveness are typical examples.
In stark contrast, qubit computers may execute processes at an exponential scale faster for specific tasks. The processing speed of qubit computing leverages the principles of superposition; they have the ability to manage massive data sets simultaneously, fundamentally reshaping how we analyze information.
- Key Characteristics:
- Advantages:
- Disadvantages:
- Ability to operate on multiple data points concurrently.
- Quantum algorithms such as Grover’s can significantly reduce search times in unstructured databases.
- Faster processing for large datasets, beneficial for fields like big data analytics.
- Capability to restructure processes that would take classical computers an impractically long time.
- Throws up technical challenges, especially concerning qubit coherence and error rates.
Types of Qubits
In the realm of quantum computing, understanding the different types of qubits is vital to grasping how this technology works and evolves. Each type carries its own set of advantages, disadvantages, and operational nuances, shaping the direction of research and application in this cutting-edge field. The distinction between superconducting qubits and trapped ion qubits highlights not only the diversity of approaches but also the potential paths available for future development.
Superconducting Qubits
Operational Mechanism
Superconducting qubits operate on the principle of superconductivity. This phenomenon allows certain materials to exhibit zero resistance to electric current when cooled below a critical temperature. The key characteristic of superconducting qubits lies in the Josephson junction, a thin barrier that separates two superconductors. When an external microwave pulse is applied, it changes the energy states of the qubit, enabling the encoding of information.
The importance of this operational mechanism cannot be overstated. It provides a relatively robust way to create quantum bits that can operate at higher temperatures compared to other qubit types. While the precision of control is exceptional, this mechanism does have its challenges, notably susceptibility to decoherence resulting from external noise.
Devices in Use
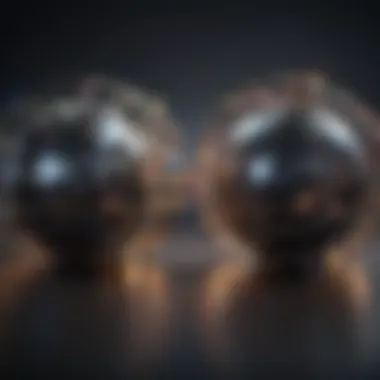
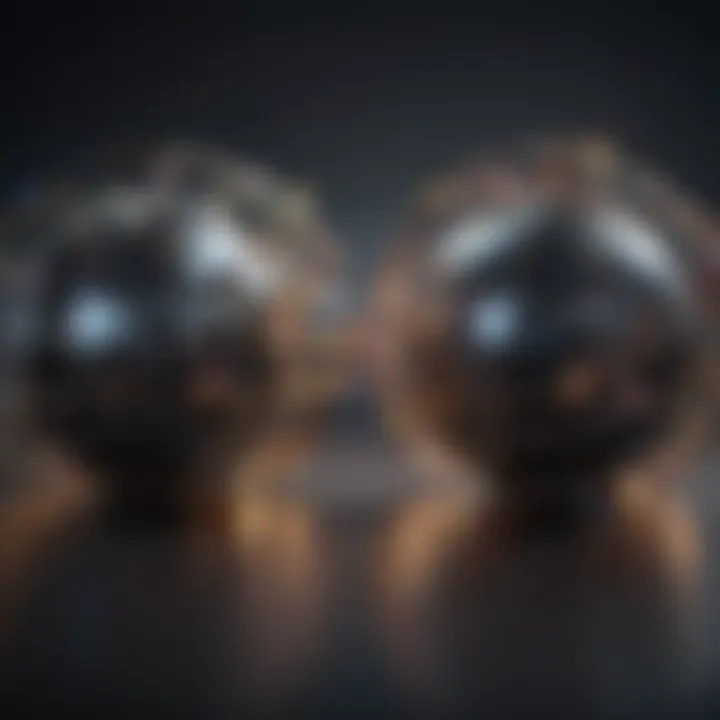
Various devices harness the power of superconducting qubits, notable among them are IBM's Quantum Hummingbird and Google's Sycamore processors. These tools have enabled significant advancements in quantum experiments. Their primary characteristic is the ability to integrate many qubits in a relatively small space, leading to complex operations that were previously unattainable.
However, the unique feature here is the requirement for extremely low temperatures to maintain superconductivity, which could pose challenges for scaling. This cooling requirement can make superconducting qubits less practical in certain scenarios, despite their high computational capabilities.
Trapped Ion Qubits
Technology Overview
Trapped ion qubits utilize individual ions that are suspended in a vacuum and manipulated using electromagnetic fields. The ions serve as qubits, with their quantum states controlled by lasers. This technology highlights the flexibility and precision achievable in manipulating qubit states, making it a strong contender in the quantum computing landscape.
A significant characteristic of this method is its inherent accuracy; the isolation from environmental factors allows for prolonged coherence times, essential for accurate quantum computations. However, the complexity of the trapped ion systems can be a limitation, requiring sophisticated setups and calibration.
Advantages and Limitations
The advantages of trapped ion qubits are striking. They tend to have longer coherence times compared to their superconducting counterparts, which is a substantial benefit in maintaining quantum states for computation. However, the primary limitation lies in scalability. While individual qubits can be controlled with great precision, arranging many ions in a way that allows for effective interaction remains a significant hurdle.
Plus, the space requirements for the apparatus often lead to practical constraints in developing larger systems. This balance of advantages and limitations presents an ongoing challenge and an area ripe for innovation as researchers strive to optimize trapped ion technology for practical use in quantum computing.
Challenges in Qubit Implementation
The journey into qubit computing is marked not only by its potential but by the hurdles that practitioners must navigate. Challenges in qubit implementation represent a crucial area of focus in this landscape. Understanding these obstacles is paramount because they can determine the feasibility and success of quantum systems. Each qubit, as a unit of quantum information, plays an essential role, yet their intrinsic properties make them susceptible to unique challenges that traditional computing does not experience.
There are several key elements intertwined with these challenges:
- Decoherence and Error Rates: When qubits are in play, their stability is fleeting. Decoherence refers to the loss of quantum coherence, a state where qubits can exhibit distinct quantum behaviors. This process can jeopardize the fidelity of quantum calculations and ultimately lead to errors that are hard to correct. Understanding decoherence is critical as it directly relates to the reliability of quantum computations.
- Scaling Qubit Systems: With a growing interest in maximizing qubit utilization, it is vital to address scaling systems effectively. As the number of qubits increase, so do the complexities involved in maintaining their interactivity and operational integrity. The increasing demand for computations that can only be solved by quantum methods propels researchers toward refining how qubits can be grouped and harnessed without succumbing to instability.
These challenges serve as hurdles, yet they also open avenues for innovation. Overcoming them could pave the way for effective implementation of quantum technology in various domains.
Decoherence and Error Rates
Decoherence is akin to watching a finely tuned clock become erratic. Once a qubit is put to work, it can quickly lose its quantum state due to interaction with its surrounding environment. The challenge here is twofold: mitigating these effects while keeping a keen eye on error rates. High error rates can cripple quantum computations, leading to unreliable outputs. Various strategies are being explored to combat these issues, such as error-correcting codes and sophisticated isolation techniques. Innovations in material science also offer hope for creating qubits that are inherently more robust against decoherence.
Scaling Qubit Systems
Scaling qubit systems introduces a plethora of complications. As systems become more expansive, the intricacies of qubit interactions complicate maintenance efforts.
Integration Challenges
Integration is at the heart of scaling, yet it often proves to be a thorny issue. Combining different types of qubits can lead to inconsistencies and vulnerabilities. Each type comes with its set of characteristics, which can affect how they perform together. Effective integration involves creating a coherent framework where multiple qubit types can function seamlessly. The robustness of this integration is vital, as it impacts system performance.
- Key Characteristic: One significant aspect of integration challenges is the need for communication protocols that can efficiently manage qubit states across various platforms.
- Unique Features: This integration often requires advanced materials and precise calibration techniques to ensure coherence is maintained over larger systems.
The implications of these integration challenges underscore the necessity for advanced engineering solutions that can facilitate a stable and scalable qubit architecture.
Maintaining Qubit Fidelity
Keeping qubit fidelity high amidst these challenges is another urgent need. Fidelity refers to the accuracy with which a qubit maintains its quantum state during calculations. Higher fidelity equates to less error, ultimately yielding a more reliable quantum processor.
- Key Characteristic: Fidelity maintenance often relies on the meticulous control of environmental conditions surrounding the qubits. Even subtle fluctuations can have outsized effects.
- Unique Features: Researchers employ careful isolation techniques and shielding measures to protect qubits from noise and interference, ensuring they can perform as intended are paramount.
The quest for maintaining high fidelity is intricate and requires innovative approaches. These efforts are fundamental to advancing the practical application of quantum computing, illustrating the delicate balance between maintaining operational integrity and harnessing qubit potential.
Recent Advances in Qubit Research
Recent developments in qubit research are crucial in understanding how quantum computing is evolving. The field has made significant progress, exploring innovative designs and boosting international cooperation for advancing technology. These strides not only enhance computational speed but also refine the accuracy and reliability of quantum operations. Greater focus on research funding indicates that investment in quantum technology is on the rise, a signal that it's taken seriously by global players.
Innovative Qubit Designs
In the realm of quantum technology, innovative qubit designs are the lifeblood of progress. Researchers have been experimenting with various methods to enhance qubit performance, such as topological qubits and photonic qubits. Topological qubits promise resistance to decoherence, a common problem in quantum systems, by encoding information in the braiding of quasiparticles. Consequently, they could lead to more stable operations, propelling quantum computing closer to reality.
Photonic qubits, on the other hand, take advantage of light's properties. With light (photons) as carriers of qubits, they exhibit rapid information transmission capabilities. Using photons in quantum computers could unravel the complexities of integrating qubits, allowing for networking quantum devices over distances, which is a profound leap forward. Implementation of these designs is often challenging, yet the benefits can be transformative.
International Collaborations
International partnerships have become a cornerstone in qubit research, enhancing the acceleration of breakthroughs in this field.
Notable Projects
One project making waves is the Quantum Internet Alliance, which connects various institutions for developing quantum networks. This project stands out for its global reach, pooling resources and expertise from Europe, the US, and beyond. It showcases a key characteristic of international collaboration: sharing knowledge across borders to design systems that rely on quantum principles. The collaborative effort taps into each participant's strengths, making it a suitable option for accelerating advancements.
However, one downside is that coordinating goals among diverse teams and aligning different priorities can sometimes slow progress.
Research Funding
Research funding plays an essential role in propelling advancements in qubit technology. National governments and private firms are increasingly willing to invest, highlighting the significance placed on quantum research. The National Quantum Initiative Act in the United States, for example, demonstrates governmental support by funneling significant resources into quantum projects.
This funding is vital because it empowers researchers to explore bold, risky ideas that might not attract traditional funding sources. However, with so many skimming the surface, one challenge remains: not all funded projects yield viable results; failures can sometimes dilute the zeal for ongoing support.
Advancing knowledge in qubit designs, fostering international realities, and securing important funding are just some pieces of the puzzle within the broader story of quantum computing. As the landscape continues to evolve, such elements help shape what is next for this fascinating field.
Applications of Qubit Computing
The potential applications of qubit computing have garnered significant interest in various fields, showcasing its ability to transform traditional practices. This section aims to unpack the vital roles that qubits can play, focusing on cryptography and drug discovery, two sectors where quantum computing is poised to make a considerable impact. As the world transitions towards more secure and efficient technologies, understanding these applications becomes imperative.
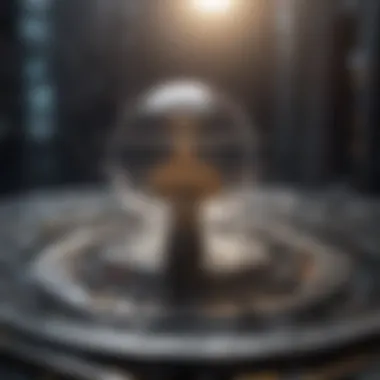
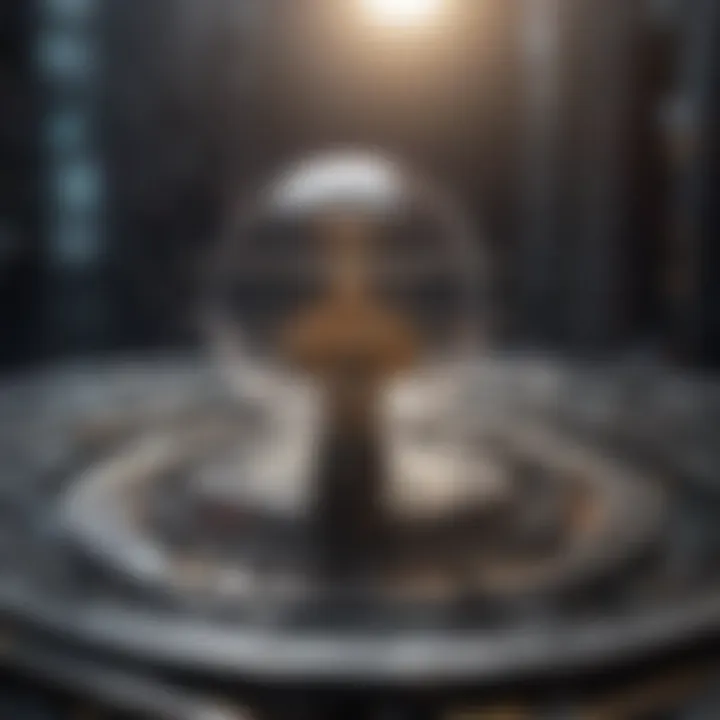
Cryptography and Security
Cryptography, the art and science of securing communication, stands to gain immensely from qubit technologies. In an era where digital communication is a double-edged sword, the inherent strengths of quantum mechanics offer solutions that classic encryption methods fall short of.
Quantum Key Distribution
One of the crown jewels of quantum cryptography is Quantum Key Distribution (QKD). QKD utilizes the principles of quantum superposition and entanglement to create secure communication channels. The unique feature of QKD is its ability to detect eavesdropping. If someone tries to intercept the quantum signals, the act of measurement alters them, alerting the sender and receiver to potential intruders. This makes QKD not only secure but also a fascinating solution in securing modern digital communications.
The applicability of QKD becomes particularly salient when considering the impending arrival of quantum computers, which threaten to break many widely-used encryption methods. For organizations looking to future-proof their security infrastructure, QKD is rapidly becoming a preferred choice.
- Advantages of QKD include its robust security features against any interception attempts.
- However, disadvantages may stem from implementing and maintaining the systems required for its operation, making it a costly investment at this time.
Impacts on Current Security Protocols
The implications of quantum computing on current security protocols deserve scrutiny. As quantum computers evolve, they could potentially decipher encrypted information in ways previously deemed impossible. Traditional encryption methods like RSA and ECC may soon be on the chopping block.
The key characteristic of these impacts lies in their transformative nature; the very foundation of how organizations handle sensitive information may need a complete overhaul. This necessity fosters a growing interest in developing quantum-resistant algorithms, ensuring that data integrity remains intact.
A unique feature of this upheaval is the adaptation period that current infrastructures must undergo. The race is on for researchers and tech providers to establish reliable replacements that can withstand quantum threats while maintaining efficiency.
- Advantages of updating security protocols include enhanced safety measures and technological robustness.
- Disadvantages often come in the form of transitional difficulties, requiring legacy systems to adapt or be replaced fully.
Drug Discovery and Materials Science
Qubit computing isn't limited to the realm of information security; it holds promising applications in the fields of drug discovery and materials science. The capacity to simulate complex molecular reactions could significantly reduce the time and cost associated with these fields.
Simulating Molecular Interactions
Current methods of simulating molecular interactions can be prohibitively slow and expensive. However, the use of quantum computers allows for a level of detail and speed that classical computers struggle with. The ability to analyze and predict molecular behavior accurately helps researchers innovate faster.
The significance of this application lies in its potential. By using qubits to model interactions at a quantum level, researchers could isolate effective treatments for diseases or evaluate new materials with unprecedented accuracy. This ability highlights why quantum simulation is being actively pursued as a game changer in fields such as pharmaceuticals.
- Advantages entail faster research cycles and a possible increase in discovery rates.
- On the contrary, the disadvantages revolve around the complexity of research methodologies needed to incorporate quantum computing effectively into traditional practices.
Accelerating Research Processes
The acceleration afforded by qubit computing doesn't stop at simulation; it extends into the overall research process itself. By integrating quantum algorithms into research workflows, scientists can sift through vast amounts of data more efficiently, identifying patterns that would elude classical methods.
With the increasing competition in drug development and materials innovation, exploiting this capability is vital. The potential to explore countless hypotheses and refine solutions quickly makes it appealing for companies aiming for breakthroughs.
A unique feature of this advancement is its multidisciplinary nature, as quantum computing intersects with biology, chemistry, and materials science to produce collaborative breakthroughs.
- Advantages include quicker results and higher-quality predictions that can lead to substantial economic savings.
- Disadvantages might include a reliance on still-developing quantum technologies, which means that integration into existing systems can still be risky or uncertain.
Future Directions in Qubit Computing
In the rapidly evolving landscape of quantum computing, the future directions in qubit computing hold immense weight for the entire technological spectrum. Qubit computing is not merely an advanced computational paradigm; it is a frontier, where theoretical physics meets applied engineering, and the implications ripple through various industries. The exploration of potential advancements helps illuminate pathways that can lead to more effective solutions across computing problems, cryptograph security, and complex simulations, among others.
Predicted Technological Trends
Looking ahead, it's vital to engage with the predicted technological trends that might shape the qubit computing landscape. Several focal points are emerging:
- Increased Qubit Scale-Up: Efforts focused on increasing the number of qubits packed into a system are ongoing. With this scaling comes the enormous challenge of maintaining qubit coherence and interconnectivity. Manufacturers are experimenting with diverse architectures, such as topological qubits, which aim not only for quantity but for quality.
- Integration of Quantum and Classical Systems: It’s plausible to envision future systems where classic and quantum computing cooperate seamlessly. This mixed approach could allow businesses to utilize quantum processors for highly complex tasks while maintaining classical systems for everyday operations.
- Improved Error Correction Techniques: As qubit systems evolve, it is inevitable that research will delve deeper into error correction codes. The way errors are mitigated will greatly affect the reliability of quantum computations in real-world applications.
"The coalescence of classical and quantum systems may provide the bridge to making quantum computing practical and broadly adopted."
Ethical Considerations and Societal Impacts
As we keep pushing the technological envelope, ethical considerations and societal impacts of qubit computing become more pronounced. With greater power comes greater responsibility, and it’s essential to dissect how emerging technologies can affect the social fabric.
Responsibility in Innovation
The responsibility in innovation theme addresses the onus that developers, researchers, and corporations carry as they advance qubit computing technology. This responsibility manifests in several ways:
- Transparency in Research: Disclosing findings can foster trust within the community and among the general public, helping alleviate fears fueled by misunderstanding.
- Mitigating Misuse Potential: The applications of quantum computing, especially in cryptography, can pose threats if misused. Hence, there must be guidelines and best practices that govern its use.
Despite these challenges, upholding responsibility in innovation is fundamentally a beneficial choice. The innovation that prioritizes ethical considerations can result in technology being viewed more favorably by society. This commitment to ethical innovation also positions organizations as leaders in promoting a secure, sustainable technological future.
Preparing for Quantum Changes
The notion of preparing for quantum changes navigates the adaptations society must embrace as quantum technology develops. Key aspects include:
- Education and Awareness Initiatives: Teaching the next generation about quantum principles will be crucial. However, not all educational bodies will keep pace, leading to gaps in understanding.
- Regulatory Frameworks: Governments and regulatory entities need to establish guidelines that can monitor and foster the use of qubit computing without stifling innovation.
The intrinsic value in preparing for quantum changes lies in its capacity to bridge the knowledge gap about emerging tech and implement protocols ensuring responsible usage. It may be viewed as both a challenge and an opportunity—an opportunity to redefine the interfaces between technology and daily life.
Closure
The conclusion serves as the cornerstone of this article, wrapping up the myriad concepts discussed while driving home the significant implications of qubit computing. As we have journeyed through the definition and intricacies of qubits, from their historical background to their transformative applications, it becomes evident that this field is more than just a technological advancement—it’s a potential game-changer that straddles the line between theory and application.
Recapitulating Key Insights
To recap, we have explored several key insights:
- Definition and Function of Qubits: Qubits, holding the promise of vastly improved computational power, challenge the classical binary understanding of data. Two different states can coexist through superposition, allowing for more complex computations.
- Quantum Principles: Essential concepts such as entanglement not only enrich the theoretical framework but also provide pathways for practical applications in fields such as cryptography and artificial intelligence.
- Types of Qubits: Various types, including superconducting and trapped-ion qubits, present unique strengths and weaknesses. Understanding these differences guides researchers in selecting the most appropriate qubit for specific applications.
- Challenges: While the potential is enormous, the hurdles in implementation—decoherence and scaling challenges —must be adequately addressed for quantum computing to become mainstream.
- Applications and Future Directions: The impact of qubit computing on sectors like drug discovery and cybersecurity highlights immense opportunities. The ethical dimensions and societal implications also warrant careful consideration as these technologies evolve.
The Path Ahead
As we look ahead, the future of qubit computing appears vibrant but daunting. The overwhelming consensus among scientists is that overcoming current limitations will restore faith in quantum technologies. Here are a few anticipated trends and considerations moving forward:
- Increased Investment in Research: More funds will likely be funneled into qubit research, enabling innovations that can bridge gaps in current technologies.
- Interdisciplinary Collaboration: Fields beyond computer science, such as material science and psychology, are expected to contribute to advancements in quantum technologies. This diversification will result in enriched methodologies and perspectives.
- Ethical Frameworks: As qubit computing matures, developing ethical frameworks will be imperative. Responsible innovation will not only be about harnessing computational power but also ensuring it serves the greater good.
- Educational Initiatives: Strengthening educational programs on quantum technologies will equip future generations with the tools to navigate this complex landscape, fostering a more informed populace capable of engaging with arising issues and opportunities.