Synthetic Gas: Exploring Production and Applications
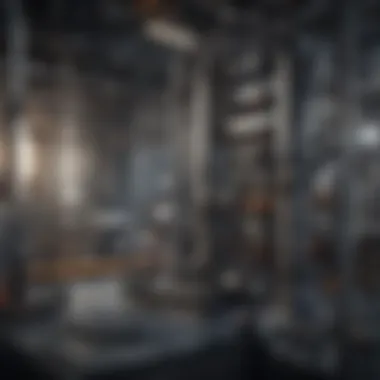
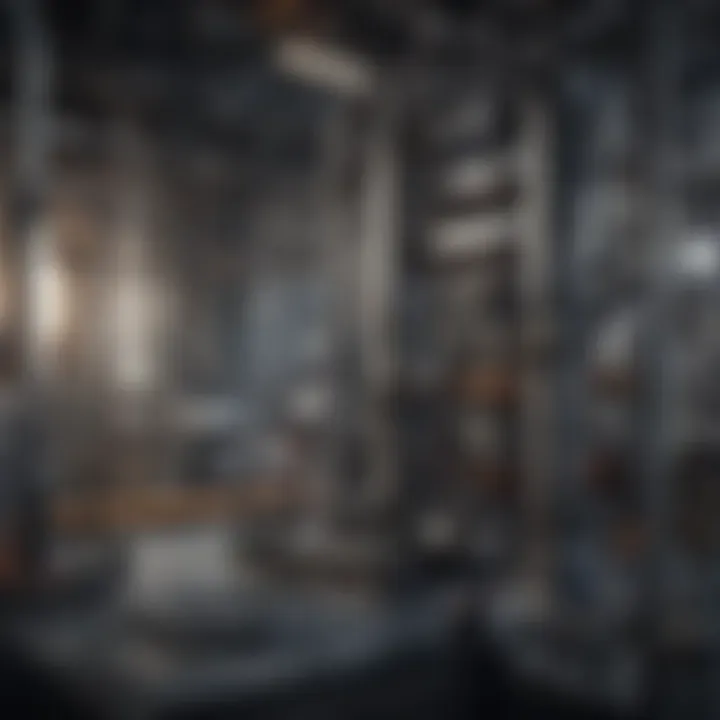
Intro
Synthetic gas, commonly known as syngas, emerges as a focal point in the conversation about future energy solutions. It serves as a transitional fuel, bridging the gap between traditional fossil fuels and more renewable energy sources. With rising environmental concerns, the production and utilization of synthetic gas present an intriguing alternative that warrants detailed review. This exploration aims to provide clarity on the various aspects of synthetic gasβfrom its production methodologies to applications, and its place in the broader context of energy transition.
Recent Advances
Latest Discoveries
Recent years have seen significant advances in the field of synthetic gas. Researchers have identified novel feedstocks for syngas production, expanding the horizons of resource utilization. From agricultural waste to municipal solid waste, the variety has increased, highlighting the importance of circular economy principles. This diversity in feedstocks contributes not only to energy security but also to waste management solutions.
Moreover, advancements in catalysis and gasification technology have led to higher efficiency in syngas production. Techniques such as plasma gasification and supercritical water gasification are gaining attention for their higher thermal efficiency and lower emissions compared to conventional methods.
Technological Innovations
The integration of artificial intelligence and machine learning in the production of synthetic gas stands out as a transformative trend. These technologies help optimize operational parameters, reducing costs and improving yield. Predictive maintenance, enabled by data analytics, ensures higher uptime and reliability in syngas facilities.
Innovations in carbon capture and storage (CCS) technologies also play a critical role. By incorporating CCS with synthetic gas production, it is possible to mitigate the environmental impacts associated with carbon dioxide emissions. This aligns harmoniously with global climate objectives, enhancing the appeal of synthetic gas as a cleaner alternative to conventional fuels.
"Synthetic gas generation using renewable feedstocks, combined with carbon capture, can significantly lower greenhouse gas emissions, supporting global sustainability efforts."
Methodology
Research Design
To properly delve into the realm of synthetic gas, a comprehensive research design is essential. This involves both qualitative and quantitative methodologies to capture a holistic view of the subject matter. Academic research, industry reports, and case studies form the backbone of credible information, ensuring varied perspectives are represented.
Data Collection Techniques
Data collection techniques encompass a range of methods. Surveys and interviews with industry professionals provide insights into real-world applications and emerging trends. Comprehensive literature reviews aid in understanding historical and current technologies related to synthetic gas production.
Additionally, experimental studies focusing on new feedstocks and production technologies help validate theoretical models and yield practical data. This multifaceted approach allows for a nuanced understanding of synthetic gas, setting the stage for subsequent exploration of its applications, implications, and future potential.
Understanding Synthetic Gas
The study of synthetic gas is critical as it addresses various aspects of modern energy needs. Synthetic gas, often referred to as syngas, serves as a pivotal element within the global energy landscape. It bridges classical fossil fuel reliance with emerging renewable energy sources. Understanding this subject is crucial for multiple groups, including policymakers, educators, and professionals in energy sectors.
Synthetic gas is generated through different production methods, each with distinct environmental impacts and applications. Recognizing these methods allows for informed decisions regarding energy production and usage. Moreover, synthetic gas plays a role in reducing greenhouse gas emissions, making it relevant in discussions related to environmental sustainability.
Definition and Composition
Synthetic gas is primarily composed of hydrogen and carbon monoxide, produced through processes such as gasification or reforming. Its chemical structure allows it to convert into other fuels, such as methane or methanol. The composition varies with production processes, leading to different applications across industries.
Some common components of synthetic gas include:
- Hydrogen (): A clean fuel source, often used in fuel cells or as a raw material for producing other chemicals.
- Carbon Monoxide (CO): Crucial in processes like Fischer-Tropsch synthesis, converting it into liquid hydrocarbons.
- Carbon Dioxide (CO2): Generated during production, but can be captured and reused.
The balanced composition of synthetic gas makes it suitable for a variety of applications, enhancing its attractiveness as an alternative energy source.
Historical Context
The origins of synthetic gas date back to the early 19th century, when it was first produced for illumination. Coal gas, as it was called then, was essential for street lighting before the advent of electricity. Over time, the scope of synthetic gas has broadened significantly.
In the 20th century, advancements in technology led to the establishment of gasification processes. World War II saw increased interest in syngas production due to its potential as a fuel alternative. Many countries turned to synthetic gas as a reliable energy source during periods of fuel shortages by tapping available feedstocks like coal and biomass.
As industries now prioritize sustainability, recent decades have witnessed a renewed focus on synthetic gas. Innovations in production techniques and greater emphasis on environmental goals have positioned synthetic gas at the forefront of the energy transition. This historical evolution underlines its importance and relevance in contemporary energy discussions.
Production Methods
Understanding the production methods of synthetic gas is crucial. These methods determine the efficiency, cost-effectiveness, and environmental impact of synthetic gas generation. The production techniques are diverse, each with unique characteristics. They influence how synthetic gas can be produced from various feedstocks such as natural gas, biomass, and waste materials. Furthermore, innovations in production methods play a significant role in achieving a more sustainable energy landscape.
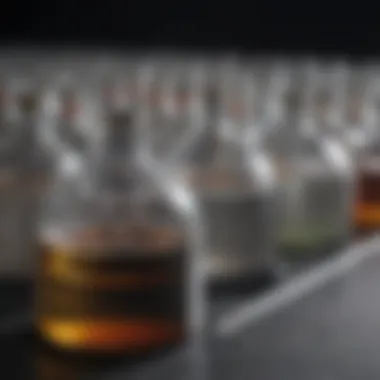
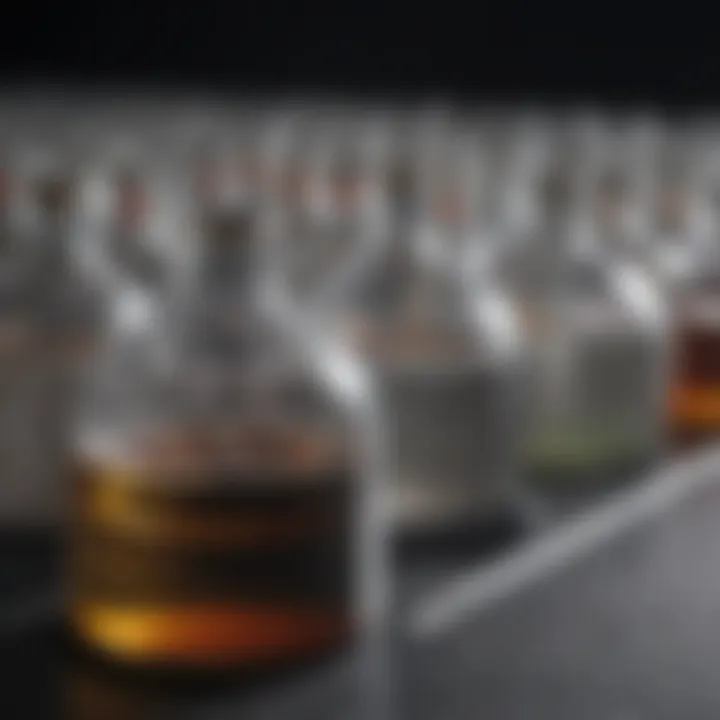
Gasification Processes
Gasification is a critical process in the production of synthetic gas. It involves the conversion of carbon-based materials into a gas mixture rich in hydrogen and carbon monoxide, which are essential components of synthetic gas. There are three main types of gasification processes: Fixed Bed Gasification, Fluidized Bed Gasification, and Entrained Flow Gasification.
Fixed Bed Gasification
Fixed Bed Gasification is a method where the feedstock is placed in a stationary bed and exposed to high temperatures in the presence of a limited amount of oxygen. This method is significant because it allows for the production of synthetic gas from solid feedstocks like coal and biomass. The main characteristic of this method is its simple design. It is often seen as a beneficial choice due to its low operational costs. However, Fixed Bed Gasification has disadvantages, such as lower efficiency compared to other methods and potential tar production, which can complicate downstream processing.
Fluidized Bed Gasification
Fluidized Bed Gasification involves suspending solid feedstock in a bed of hot, inert materials. This process enables better heat and mass transfer, making it highly efficient. The key characteristic of this method lies in its ability to handle a wide range of feedstocks, including low-quality coal and biomass. Its popularity arises from its high throughput and flexibility. However, Fluidized Bed Gasification can be complex in design and operation, leading to higher initial investment costs. Nonetheless, the potential for continuous operation makes it an attractive option for synthetic gas production.
Entrained Flow Gasification
Entrained Flow Gasification is a fast and efficient process, where feedstocks are converted into synthetic gas in a suspension state. It typically operates at high pressures and temperatures, resulting in high conversion efficiency. The main advantage of Entrained Flow Gasification is its effectiveness with a variety of feedstocks including biomass and liquid fuels. Its unique feature includes the ability to produce high-quality syngas with low contaminants. However, the drawbacks involve substantial energy requirements for heating and the need for fine feedstock preparation.
Reforming Techniques
Reforming techniques are essential for converting hydrocarbons into a more useful form of synthetic gas. The most common methods include Steam Methane Reforming, Autothermal Reforming, and CO2 Reforming.
Steam Methane Reforming
Steam Methane Reforming (SMR) is the most widely used method for hydrogen production. In this process, methane reacts with steam to produce hydrogen and carbon monoxide. A major advantage of SMR is its established technology, which makes it highly scalable and efficient. This method is particularly beneficial for industries looking to produce hydrogen for synthetic fuels. However, reliance on natural gas can pose challenges, particularly concerning price volatility and greenhouse gas emissions.
Autothermal Reforming
Autothermal Reforming combines the processes of steam reforming and partial oxidation. This method provides a more integrated approach to hydrogen production, balancing heat and reactant requirements. The main appeal of Autothermal Reforming lies in its ability to produce hydrogen more efficiently than traditional methods. However, the complexity of managing the reaction can lead to operational difficulties, which may hinder its widespread adoption.
CO2 Reforming
CO2 Reforming involves the reaction of methane with carbon dioxide to produce hydrogen and carbon monoxide. This technique not only generates useful products but also helps in reducing CO2 emissions. The key characteristic of CO2 Reforming is its role in carbon capture and utilization. This makes it a beneficial method in terms of environmental impact. However, challenges exist in terms of reaction efficiency and the need for specific catalysts, which can limit its application in certain contexts.
Fermentation and Anaerobic Digestion
Fermentation and anaerobic digestion represent alternative pathways for synthetic gas production, focusing primarily on biological processes.
Fermentation converts organic materials, like sugars, into gases such as methane or hydrogen through microbial action. This method is particularly valuable for utilizing biomass and organic waste. Anaerobic digestion, on the other hand, breaks down organic materials in the absence of oxygen, producing biogas rich in methane. Both share advantages of using waste resources and reducing environmental impact. However, they traditionally yield lower energy densities compared to other methods and may involve longer processing times.
"The choice of production method significantly influences the economic viability and environmental sustainability of synthetic gas generation."
The selection of production methods is crucial for synthetic gas technologies. Each technique offers different advantages and challenges, shaping the future of energy production.
Feedstocks for Synthetic Gas Production
Feedstocks are the foundational materials used in the production of synthetic gas. They play a crucial role in determining the efficiency, sustainability, and economic viability of synthetic gas generation. Understanding the various types of feedstocks is essential for grasping the overall dynamics of synthetic gas as an energy source. Each feedstock carries unique properties, benefits, and challenges that impact its integration into synthetic gas production systems.
Natural Gas
Natural gas is considered one of the primary feedstocks for synthetic gas production. Its high hydrogen content makes it a favorable option, providing a cleaner energy alternative compared to other fossil fuels. The extraction and processing of natural gas typically involve established infrastructure. This accessibility ensures its role as a key material in gasification and reforming processes. However, reliance on natural gas also presents challenges. The extraction process can contribute to environmental degradation, and price fluctuations may affect the economic stability of projects reliant on this feedstock.
Biomass Resources
Biomass resources, including agricultural residues, energy crops, and organic waste, offer a renewable alternative for producing synthetic gas. The utilization of biomass not only provides an approach to waste management but also can significantly lower greenhouse gas emissions compared to fossil fuels. The conversion of biomass into synthetic gas can be achieved through processes such as gasification and anaerobic digestion. However, challenges exist in feedstock collection, logistics, and pre-treatment. These factors can influence the overall efficiency and cost of biomass-to-gas processes.
Coal as a Feedstock
Coal has been used historically as a feedstock for synthetic gas production. It contains a high carbon content, which can result in significant energy yield in gasification processes. Nonetheless, coal is associated with substantial carbon emissions, raising concerns over its environmental impact. The shift towards cleaner energy sources is decreasing the attractiveness of coal. Despite this, some technologies are being developed to mitigate emissions while utilizing coal, but these are often costly and complex to implement.
Waste Materials
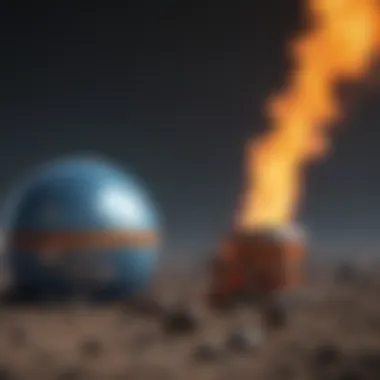
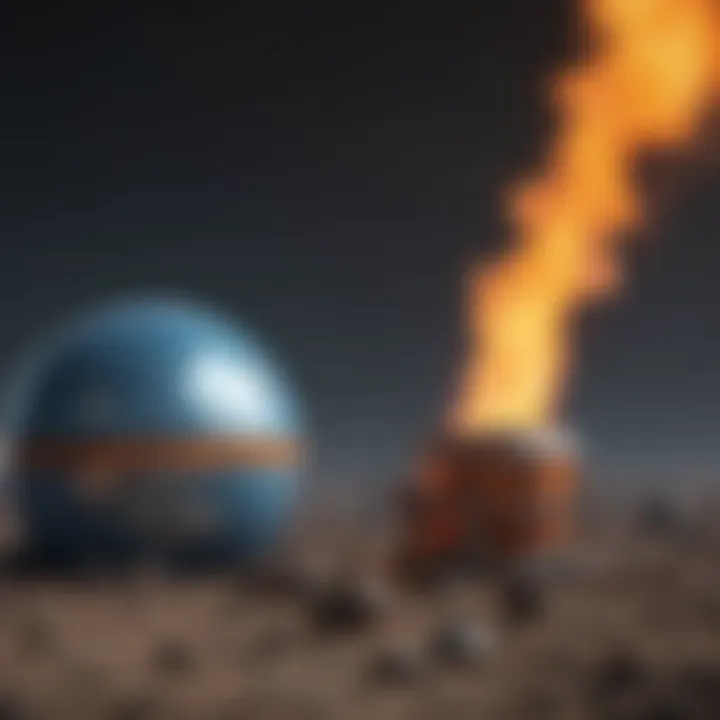
The use of waste materials as a feedstock for synthetic gas production introduces an innovative approach to waste management. Organic waste, such as that from landfills or agricultural processes, can be converted into synthetic gas through various methods including gasification and anaerobic digestion. This method not only reduces landfill mass but also provides an energy source that can contribute to a circular economy. Key considerations with waste materials include contamination and the variability in composition, which can affect processing efficiency. However, the benefits often outweigh these hurdles, making it a valuable feedstock in the renewable energy landscape.
"The transition to synthetic gas diversifies energy sources and promotes a more sustainable future."
Applications of Synthetic Gas
Synthetic gas, commonly known as syngas, plays a crucial role across various sectors. Its versatility allows it to be utilized for energy production, chemical manufacturing, and as a fuel source in specialized applications. Understanding these uses is vital in grasping the importance of synthetic gas within the broader context of renewable energy and sustainable practices.
Energy Generation
In energy production, synthetic gas serves as an efficient alternative to traditional fossil fuels. It can be used for electricity generation in gas turbines or internal combustion engines. One important benefit is its higher conversion efficiency, which can lead to reduced emissions when compared to burning coal directly. Furthermore, syngas can facilitate the integration of renewable energy sources by acting as a reliable energy carrier.
The flexibility in its generation process is also noteworthy. Synthetic gas provides an ability to balance energy supply and demand. As such, it can complement renewable resources like solar and wind energy, where energy generation can be intermittent. This ability positions synthetic gas as a valuable component of the future energy mix.
Chemical Production
Chemical production is another significant application of synthetic gas. It can be a feedstock for the synthesis of various chemicals, notably methanol and hydrogen.
Methanol
Methanol is one of the primary products derived from synthetic gas. It is an essential building block in the manufacture of numerous chemical products, including formaldehyde and acetic acid. Methanol has a high octane rating, making it suitable for blending with gasoline and could serve as a transportation fuel in the near future.
A key characteristic of methanol is its ease of transport and storage when compared to other fuels. Its production process can leverage various feedstocks, including biomass and CO2 captured from industrial processes. This adaptability is a distinct advantage, especially as industries look for solutions to reduce their carbon footprint. However, while methanol presents several benefits, considerations around its safety and environmental impact must be managed carefully.
Hydrogen
Hydrogen is another vital use of synthetic gas. It holds substantial promise in a range of applications, including fuel cell technology and as a clean energy carrier. Hydrogen can be produced through the reforming of syngas, where its abundance in the universe lends it significant potential in the energy stage of our resources.
One of the key benefits of hydrogen is its capacity to produce energy with water as the only by-product when combusted or used in fuel cells. This unique feature positions hydrogen as a clean energy solution that aligns with global ambitions to reduce emissions and dependency on fossil fuels. However, generating hydrogen can involve significant energy inputs, which raises concerns regarding the overall sustainability of its production.
Fuel Cells
The use of synthetic gas in fuel cells marks a pivotal advancement in energy technology. Fuel cells convert chemical energy directly into electricity through an electrochemical reaction, without combustion. This process is more efficient than traditional power generation and produces virtually zero pollutants, making it a clean energy alternative.
Fuel cells powered by synthetic gas are particularly suitable for transportation and stationary applications. They offer high energy density and scalability. While the market for fuel cells is growing, challenges remain in terms of cost and the development of infrastructure required for widespread adoption.
"Synthetic gas serves as a bridge between current energy technologies and future sustainable solutions."
As synthetic gas finds its footing in these various applications, it is evident that its development could influence energy strategies globally and drive the transition towards a more sustainable energy system.
Environmental Impact
The production and utilization of synthetic gas have significant implications for the environment. Understanding these impacts is crucial for assessing its role in energy transition and sustainability. Synthetic gas, often referred to as syngas, is produced from various feedstocks, which can influence its overall environmental footprint.
Carbon Emissions
When comparing synthetic gas to fossil fuels, one of the primary concerns is carbon emissions. Synthetic gas can be produced from renewable feedstocks like biomass, which inherently results in a lower carbon footprint compared to traditional fossil fuels. However, the reality is complex.
- Source of Feedstock: If the feedstock used for production is from renewable resources, carbon emissions are markedly reduced.
- Production Method: The method of production also impacts emissions. For example, gasification processes may emit more carbon than fermentation methods.
- Lifecycle Analysis: A thorough lifecycle analysis reveals how emissions differ at each stage of production, from feedstock harvesting to gas utilization.
Efforts to optimize production techniques are necessary to minimize these emissions. Advances in technology are crucial for developing cleaner synthesis routes.
Comparative Analysis with Fossil Fuels
The environmental impact of synthetic gas becomes more palpable when directly compared to fossil fuels.
- GHG Emissions: Research indicates that syngas typically results in lower greenhouse gas emissions when the production is managed well.
- Resource Availability: Fossil fuels are finite, while synthetic gas can be produced from a broader range of materials, including waste and biomass. This approach promotes a circular economy and reduces dependency on depleting resources.
- Air Quality: When combusted, syngas generally results in fewer pollutants emitted into the air compared to traditional fossil fuels. This shift can lead to improved air quality, particularly in urban areas reliant on fossil fuel energy.
"Transitioning to synthetic gas could be essential for reducing our reliance on fossil fuels while still utilizing existing infrastructure."
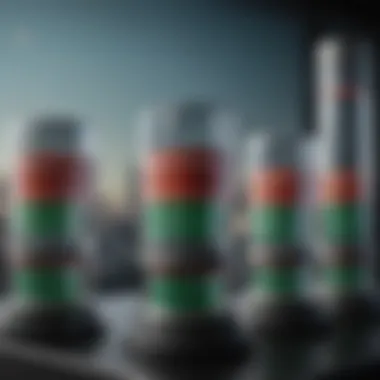
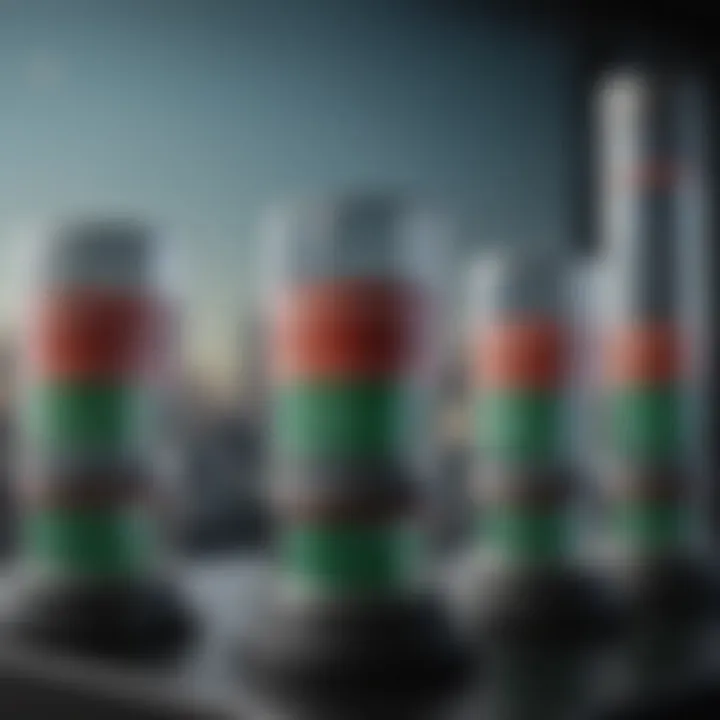
Role in Renewable Energy Integration
Synthetic gas holds promise as a versatile energy carrier that can facilitate the integration of renewable energies into the existing energy system. Here are several key points to consider:
- Storage Capability: Unlike direct electricity, synthetic gas can be stored and transported more easily. It allows for better management of energy supply and demand.
- Compatibility: Syngas can be used in existing gas infrastructure, enabling a smoother transition from fossil fuel-based systems.
- Renewable Syngas: The production of synthetic gas from renewable sources promotes energy diversity and supports the growth of the renewable sector.
Thus, synthetic gas could play a role in balancing intermittent renewable energy sources and ensuring energy security.
The analysis of environmental impacts associated with synthetic gas demonstrates both opportunities and challenges. As the sector evolves, continuous innovations in technology and shifts in policy will be necessary to enhance the sustainability of synthetic gas, minimizing its ecological footprint while maximizing its applications in the emerging sustainable energy landscape.
Economic Considerations
The economic factors surrounding synthetic gas are critical to understand its viability and potential for growth in the energy sector. As energy demands rise and the need for cleaner alternatives to fossil fuels becomes urgent, synthetic gas presents an appealing solution. This section explores various components of economic considerations, including cost analysis of production methods, market trends, and investment opportunities. The information provided here will show how synthetic gas can not only be a key player in the energy transition but also a commercial entity worth investing in.
Cost Analysis of Production Methods
The cost of producing synthetic gas can vary significantly based on the method employed. Each production technique has its own financial implications that influence decision-making.
- Gasification Process Costs: Gasification is one of the most common methods. The costs are generally high due to equipment and operational expenses. Factors such as the feedstock type significantly affect overall expenses.
- Reforming Techniques: While steam methane reforming and autothermal reforming are widely used, they have varying costs based on feedstock pricing and market demand. Steam methane reforming, for instance, can utilize natural gas efficiently. In contrast, CO2 reforming might involve higher operational costs.
- Fermentation and Anaerobic Digestion: These methods tend to be less expensive but require a steady supply of biomass. The pricing also fluctuates based on the feedstockβs availability and the local market.
Overall, a detailed analysis is essential for industries considering investment in synthetic gas production. Companies must carefully weigh production methods and their resultant costs against potential profit margins.
Market Trends and Opportunities
The synthetic gas market is evolving, influenced by shifting energy policies and environmental concerns. Identifying current trends provides insight into where opportunities lie.
- Shift Towards Sustainability: There is growing recognition of synthetic gas as a cleaner alternative to traditional fuels. This trend aligns with global efforts to reduce greenhouse gas emissions.
- Technological Advancements: Innovations in production methods are improving efficiencies and reducing costs. Companies investing in new technologies often find themselves in favorable positions.
- Government Policies: Subsidies and incentives for cleaner energy sources create fertile ground for synthetic gas. Regulations favoring reduced carbon emissions drive interest in synthetic gas solutions.
These trends suggest a favorable market environment for synthetic gas. Companies leveraging these aspects stand to benefit, not only economically but also in their sustainability missions.
Investment in Synthetic Gas Technology
Investment in synthetic gas technology offers a blend of risk and reward. As the market grows, attracting funding becomes essential for scaling operations and driving innovation.
- Venture Capital Interest: Many startups in the synthetic gas field have attracted venture capital investment. Investors see the potential for both financial returns and positive environmental impact.
- Long-Term Viability: Investors recognize synthetic gas as part of the longer-term energy solution. By committing resources today, they can position themselves in an emerging market that is likely to grow.
- Partnership Opportunities: Collaborations with established energy companies can provide both funding and expertise. These partnerships can mitigate risks and enhance innovation potential.
Future Outlook of Synthetic Gas
The future of synthetic gas holds significant importance, particularly in the context of a global shift towards sustainable energy solutions. This section will explore various aspects of synthetic gas, including technological advancements, regulatory frameworks, and comparative trends that may shape its landscape. The relevance of this topic cannot be overstated; as the world grapples with climate change and resource depletion, synthetic gas emerges as a versatile alternative. It is crucial to understand how developments in this field may influence energy practices and policies in the coming years.
Technological Innovations
Technological innovations are at the heart of synthetic gas development. Advances in production techniques are making synthetic gas more viable and efficient. One such innovation is the improvement in gasification and reforming processes. Enhanced catalytic materials and reactor designs significantly increase conversion efficiency. For instance, recent research into high-temperature gasification technologies is showing potential for higher yields from various feedstocks.
Another notable trend is the integration of automation and digitalization in production facilities. Technologies such as artificial intelligence and machine learning can optimize operations, leading to better resource management and reduced costs. Furthermore, innovations in carbon capture and utilization technologies can enhance the sustainability of synthetic gas production.
Overall, these technological advancements not only enhance production efficiency but also contribute to minimizing the ecological footprint of synthetic gas, aligning it more closely with global sustainability goals.
Policy and Regulation Perspectives
The role of policy and regulation cannot be ignored when evaluating the future of synthetic gas. Governments globally recognize the need for cleaner energy solutions. Many countries are introducing policies that encourage the development and use of synthetic gas. This includes subsidies for research and innovation in synthetic gas technologies and regulations that favor cleaner alternatives over fossil fuels.
Moreover, international agreements aimed at reducing greenhouse gas emissions create a supportive environment for synthetic gas. Countries are under pressure to meet their climate targets, thus enhancing the demand for cleaner fuel options. Synthetic gas, with its lower carbon profile, stands to benefit from these regulatory frameworks.
However, regulatory uncertainties still pose challenges. The dynamics of how policies are shaped can impact investment decisions and market stability. Clear and supportive regulations will be essential for unlocking the potential of synthetic gas and ensuring it plays a vital role in future energy systems.
Comparative Future Trends
In assessing the comparative future trends of synthetic gas, it is essential to view it within the broader context of the global energy transition. Synthetic gas presents a flexible alternative to traditional fossil fuels and other renewable sources. This flexibility could allow it to adapt to diverse energy demands and emerge as a key player in future energy markets.
For instance, as economies gradually decarbonize, sectors that are difficult to electrify, like heavy industry and long-haul transportation, may turn to synthetic gas solutions. It can be a bridge fuel that combines the benefits of natural gas with lower environmental impacts.
Additionally, market trends suggest a growing investment in synthetic gas technologies. As countries intensify their efforts to transition to low-carbon economies, the demand for synthetic gas may increase. The alignment of synthetic gas initiatives with national energy strategies will likely shape future energy landscapes positively.
"Synthetic gas represents not just a fuel option, but a transformative approach in our quest for a sustainable energy future."