Temperature Calculation: Principles and Applications
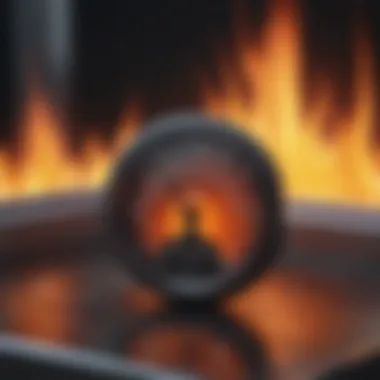
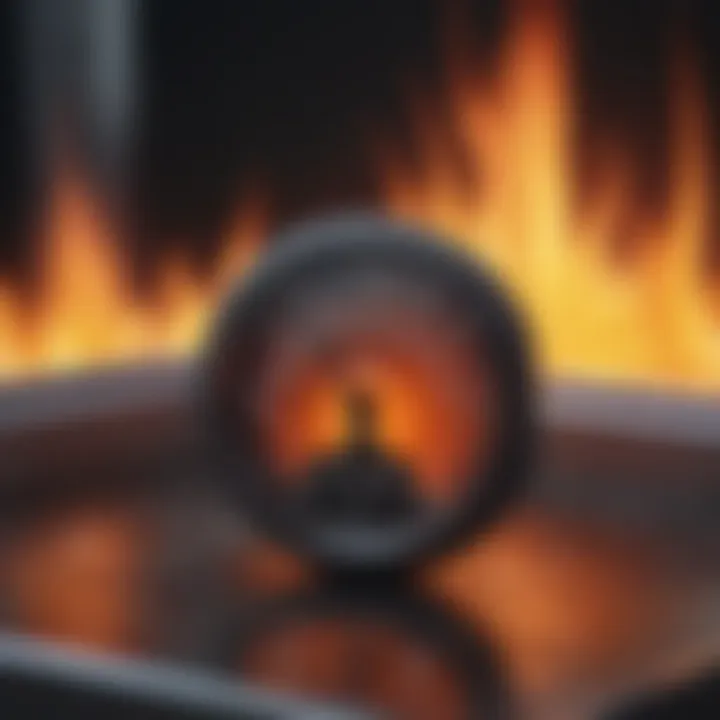
Intro
Temperature calculation is a crucial aspect of scientific inquiry, playing a significant role in diverse fields such as physics, chemistry, and engineering. It serves as a foundation for understanding thermal energy and its effects on physical systems. This article aims to explore the principles of temperature measurement, the methodologies used in its calculation, and the broad applications across various disciplines.
In the quest for precise temperature assessment, different scales come into play, namely Celsius, Fahrenheit, and Kelvin. Each scale, while serving the same broad purpose, has its unique contexts and applicability, influencing how temperature data is interpreted and utilized. Understanding these temperature scales is fundamental in both theoretical and experimental science.
Real-world applications of temperature calculations are numerous and impactful. From climate science, where global temperature trends are analyzed to understand climate change, to engineering applications that require precise temperature control in industrial processes, the implications of proper temperature measurement cannot be overstated.
By merging complex scientific principles with practical insights, this article seeks to clarify the intricacies of temperature calculation for a diverse audience. Whether for students seeking foundational knowledge, researchers exploring advanced concepts, or professionals applying these insights in their work, this review aims to provide a thorough analysis of this essential topic.
Recent Advances
Temperature calculation has seen significant developments in recent times, pushing the boundaries of what is conceivable in various scientific fields. Advances in technology and research methodologies contribute greatly to our understanding and precision in measuring temperature.
Latest Discoveries
Recent studies highlight emerging techniques in temperature measurement, including nanoscale thermometry. Nanosensors now allow for extremely precise temperature measurements at microscopic scales, proving invaluable in fields such as materials science and nanotechnology.
Additionally, novel approaches, such as infrared thermography, have enhanced non-contact temperature assessment, broadening their application in medical diagnostics and industrial monitoring. These advances are critical in improving accuracy and expanding the scope of temperature measurement.
Technological Innovations
Technological innovations have also reshaped the landscape of temperature calculation. The integration of artificial intelligence in climate modeling is one notable advancement, bolstering accuracy in predicting temperature fluctuations. AI algorithms analyze vast datasets, identifying patterns that human calculations might overlook.
Furthermore, the development of smart thermometers has revolutionized how temperature data is collected and interpreted in both clinical and home settings. These devices often sync with mobile applications to provide real-time monitoring, catering to the increased demand for health data management.
Methodology
Understanding the principles underlying temperature calculation requires a systematic approach. This section outlines the essential components of research design and data collection techniques associated with temperature measurement.
Research Design
An effective research design is fundamental in exploring temperature calculations. Typically, studies are structured to evaluate the measurement process's accuracy and precision. Controlled experiments often form the backbone of temperature research, allowing for manipulation of variables while keeping others constant.
Data Collection Techniques
Various data collection techniques are used in temperature-related studies. Instruments such as thermocouples, resistance temperature detectors, and bimetallic sensors provide different means for measuring thermal energy. Each method has its strengths and weaknesses, which must be carefully considered in the context of the research goals.
Data on temperature changes can be collected through systematic sampling, where measurements are taken at regular intervals to monitor trends over time. This approach is essential for applications in climate science, where consistent and accurate data collection is crucial for understanding long-term climate variations.
Understanding Temperature
Temperature is a fundamental aspect of both physics and chemistry. It serves as a measure of the average kinetic energy of particles in a substance. Understanding temperature is vital for several reasons, as it influences various scientific and practical applications.
Firstly, temperature affects physical state changes. For instance, it determines whether a substance is solid, liquid, or gas. Secondly, it plays a crucial role in chemical reactions. The rate and outcome of a reaction can be significantly altered by temperature fluctuations. Lastly, temperature is pivotal in engineering applications, where precise measurements are essential for safety and efficacy.
In this article, we will detail the principles, methods, and various applications of temperature calculations, providing a comprehensive understanding of why temperature is a primary concern in science and technology.
Definition of Temperature
Temperature can be defined as a quantitative measure of how hot or cold an object is. It is a scalar quantity, often measured in degrees Celsius (Β°C), Fahrenheit (Β°F), or Kelvin (K). The Kelvin scale is the SI unit of temperature, where 0 Kelvin represents absolute zero, the point at which all molecular motion ceases. Temperature is not merely a sensation; it quantifies thermal energy, making it easier to compare different energy states of various substances.
Importance of Temperature in Science
Temperature plays a crucial role in several scientific disciplines. In chemistry, it affects reaction rates, equilibrium, and solubility. For example, an increase in temperature often accelerates chemical reactions, impacting kinetics and thermodynamics dramatically. In physics, temperature is critical for understanding phenomena like heat flow and energy transfer. In more applied disciplines like environmental science, temperature influences weather patterns and climate change. Understanding temperature is essential for developing models that predict environmental changes and their impacts.
Thermal Energy: A Brief Overview
Thermal energy refers to the energy that comes from the temperature of matter. It depends on the movement and arrangement of atoms and molecules. As temperature increases, the thermal energy of a substance also increases. This energy is responsible for the sensations of heat we feel, and it plays a vital role in energy transfer processes such as conduction, convection, and radiation.
In summary, temperature provides insight into thermal energy, influencing a wide range of scientific research applications. Understanding and calculating temperature effectively are crucial components for advancements in numerous fields.
Temperature Scales
Understanding temperature scales is a fundamental aspect of temperature calculation. This section will illuminate how different systems of measurement represent thermal states, bridging concepts in various scientific realms. Grasping these scales is critical not only for academic purposes but also for practical applications in industries such as engineering, meteorology, and environmental science. Each scale has its unique features and historical significance, providing essential insights into their usage and relevance in scientific discourse.
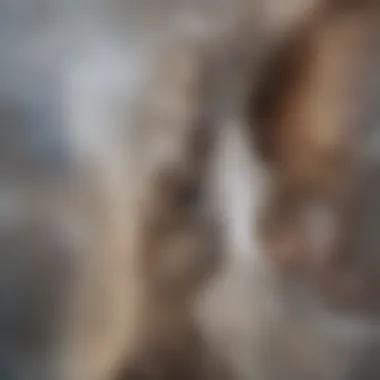
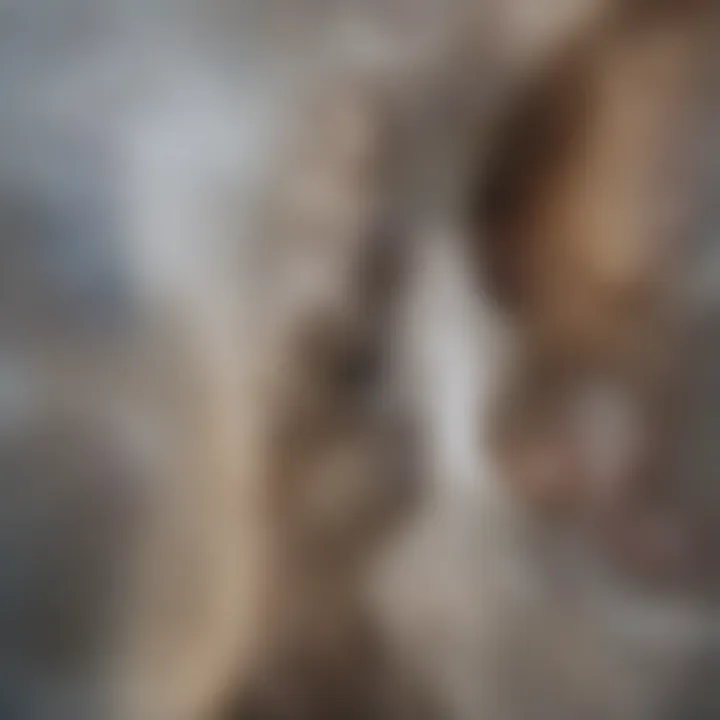
Celsius Scale
The Celsius scale, named after Anders Celsius, is widely used around the world. It defines the freezing point of water at 0 degrees and the boiling point at 100 degrees under standard atmospheric pressure. This scaleβs direct correlation to the properties of water makes it particularly intuitive for daily applications.
One key benefit of the Celsius scale is its straightforward application in scientific contexts, especially in chemistry and physics. Many scientific experiments and measurements operate within this temperature system, allowing for easy communication of results. Furthermore, the Celsius scale aligns well with human experiences, as many environmental temperatures fall comfortably within its range.
Fahrenheit Scale
The Fahrenheit scale, devised by Daniel Gabriel Fahrenheit, primarily serves the United States and a few Caribbean nations. In this scale, the freezing point of water occurs at 32 degrees, while the boiling point is at 212 degrees. This delineation might seem complex, but it was designed to correspond to everyday temperature assessments.
Understanding Fahrenheit is essential when working or communicating in regions that predominantly use this scale. It requires converting values if dealing with scientific data based on the Celsius or Kelvin scales. Thus, proficiency in converting this scale enhances clarity in contexts like climate studies or international collaborations.
Kelvin Scale
The Kelvin scale represents the absolute temperature scale and is indispensable in scientific disciplines. It begins at absolute zero, the point where molecular motion ceases, defined as 0 Kelvin. The Kelvin scale increments are congruent with the Celsius scale, where a change of 1 degree Celsius equals a change of 1 Kelvin.
The significance of the Kelvin scale extends to fields such as thermodynamics and quantum physics. Many scientific equations utilize temperature in Kelvin, promoting consistency and understanding when discussing absolute temperatures. Moreover, its universal acceptance in scientific applications supports global collaboration among researchers.
Comparison of Temperature Scales
When comparing these temperature scales, it is important to recognize their unique characteristics and applications. Here are some key points:
- Celsius:
- Fahrenheit:
- Kelvin:
- Freezing point: 0 Β°C
- Boiling point: 100 Β°C
- Commonly used worldwide except in the U.S.
- Freezing point: 32 Β°F
- Boiling point: 212 Β°F
- Used predominantly in the U.S.
- Freezing point equivalent: 273.15 K
- Boiling point equivalent: 373.15 K
- Used in scientific applications for absolute measurements.
Understanding these comparisons allows for better decision-making when selecting a temperature scale for specific purposes. Conversions between these scales require knowledge of their formulas and context, which are vital for accuracy and effectiveness in temperature calculations.
The comprehension and application of these temperature scales will pave the way for deeper exploration of the principles and methodologies involved in temperature calculations.
Principles of Temperature Calculation
Understanding the principles of temperature calculation is vital in various scientific disciplines. These principles lay the groundwork for accurate temperature measurements and allow researchers to analyze thermal phenomena effectively. They enable the distinction between various temperature scales, aiding in the conversion and application of temperature data in real-life situations. Accurate calculations are not only crucial in laboratory settings but also have implications in engineering, climate science, and even daily activities.
Basic Calculation Principles
The fundamentals of temperature calculation focus on how temperature is defined and measured. Temperature, as a measure of thermal energy, is intrinsically linked to the kinetic energy of particles in a substance. Each temperature scaleβCelsius, Fahrenheit, and Kelvinβhas its unique zero point and increment. In daily practice, understanding these scales is essential.
To conduct basic temperature calculations, one must apply the appropriate scale for the context. For example, in scientific research, the Kelvin scale is often preferred because it starts at absolute zero, where molecular motion theoretically ceases.
Key Concepts:
- Thermal Equilibrium: This concept refers to the state where two objects in contact reach the same temperature. This is vital in experiments that involve comparing thermal properties.
- Heat Transfer: Understanding how heat moves between substances is also key to accurate calculations. Heat can transfer through conduction, convection, or radiation, affecting temperature readings.
Conversion Formulas
Converting between different temperature scales involves specific formulas that relate one scale to another. To assist with these conversions, the following formulas are commonly used:
- Celsius to Fahrenheit:
[ F = \left( C \times \frac95 \right) + 32 ] - Fahrenheit to Celsius:
[ C = \left( F - 32 \right) \times \frac59 ] - Celsius to Kelvin:
[ K = C + 273.15 ] - Kelvin to Celsius:
[ C = K - 273.15 ]
These formulas are crucial for scientists and engineers. Accurate temperature readings often necessitate conversion to ensure the data is in the desired format for further analysis.
The vast compatibility across different measurement standards stabilizes communications and operations in scientific fields. Understanding these conversions diminishes errors in data interpretation.
Application of Calculational Principles
The practical applications of temperature calculation principles extend far beyond the classroom. In chemistry, precise temperature measurements are essential for reactions occurring at certain thermal thresholds. For instance, reaction rates can vary significantly with temperature changes.
In engineering, temperature calculations contribute to materials science, affecting how materials respond under different conditions. Engineers must predict how structures react to weather, ensuring safety and reliability.
Daily life also revolves around temperature calculations. From adjusting room temperature on a thermostat to understanding weather forecasts, these principles seep into common activities.
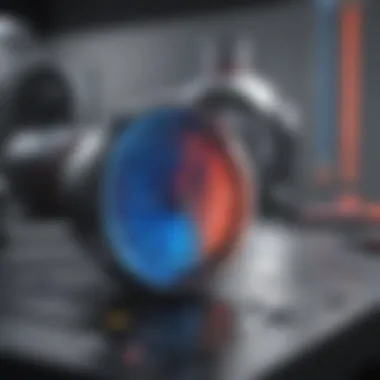
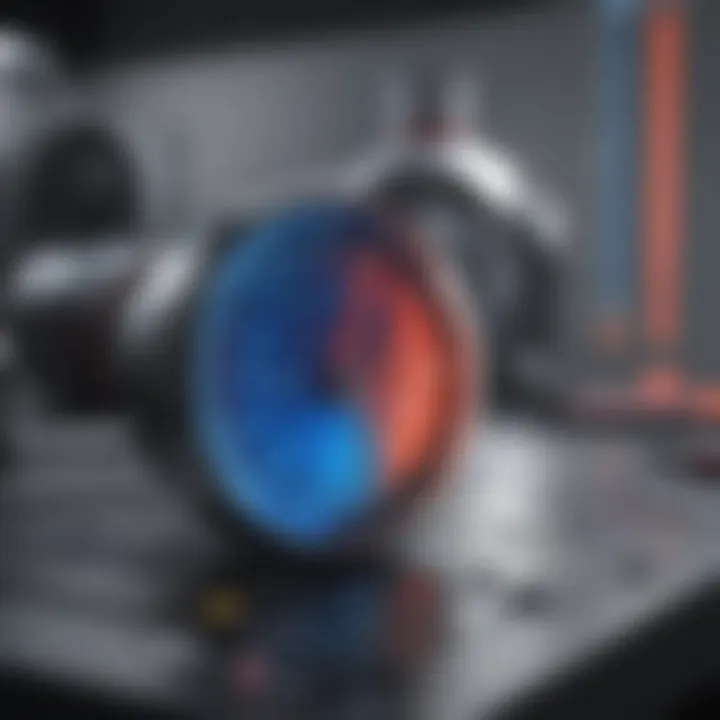
In summary, the principles of temperature calculation serve as a framework. They guide accurate measurement, conversion, and application across disciplines, enhancing our understanding of thermal dynamics in both theoretical and practical contexts.
Methods for Temperature Calculation
Methods for temperature calculation are crucial in understanding how temperature data is obtained and utilized in various applications. These methods can be categorized into experimental and calculated techniques, each offering its own benefits and considerations. Proper selection of a method ensures accurate temperature readings, which are essential in scientific research, engineering, and everyday life.
Experimental Methods
Thermocouples
Thermocouples are a widely used type of temperature sensor. They measure temperature by converting thermal energy into an electrical voltage. This unique characteristic allows for quick and accurate readings. Thermocouples are valued for their wide temperature range and durability, making them suitable for various environments, including extreme temperatures.
A notable advantage of thermocouples is their fast response time. They can quickly adjust to changes in temperature, which is vital in many applications where constant monitoring is needed. However, thermocouples may have limitations in accuracy, especially at lower temperatures, where calibration becomes more critical.
Resistance Temperature Detectors
Resistance Temperature Detectors (RTDs) function based on the principle that the electrical resistance of certain materials changes with temperature. This property allows RTDs to provide precise readouts, making them popular in laboratory settings and industrial applications.
The key feature of RTDs is their high accuracy and stability over time. This makes RTDs an excellent choice for long-term temperature measurements. One disadvantage is that RTDs tend to be more expensive than thermocouples and can be less robust in harsher conditions, which may limit their use in specific environments.
Calculated Methods
Thermal Equilibrium
Thermal equilibrium refers to the state where two systems in contact with each other cease to exchange heat energy. This concept is essential for accurate temperature calculations. By ensuring that the systems reach thermal equilibrium, one can derive precise temperature measurements without external influences affecting the results.
The significant characteristic of thermal equilibrium is its reliance on basic thermodynamic principles, making it a fundamental concept in temperature measurement. However, a limitation is that achieving this state requires time, making it impractical in rapidly changing conditions where instant data is more valuable.
Heat Transfer Principles
Heat transfer principles describe how thermal energy moves from one body to another. Understanding these principles is vital to effectively calculating temperature changes within a system. By applying equations associated with conduction, convection, and radiation, one can analyze how heat flows and predict temperature variations in different materials.
This method is beneficial because it allows for broader applications across engineering and physics. However, one challenge is that it often requires detailed knowledge of material properties and environmental factors which may complicate calculations.
"Choosing the right method for temperature measurement can significantly impact the accuracy and reliability of data in various applications, be it in scientific research or everyday use. "
Applications in Various Fields
Understanding temperature calculation is essential in numerous disciplines. The ability to measure, analyze, and apply temperature data affects various scientific and practical endeavors. Knowledge of temperature influences chemical reactions, climate modeling, engineering designs, and even daily activities. This section outlines how temperature calculation finds its relevance in different areas, emphasizing its importance for researchers, educators, and other professionals.
Temperature Calculation in Chemistry
In chemistry, temperature is a critical variable influencing reaction rates. Various chemical processes are temperature-sensitive. For instance, many reactions proceed faster at elevated temperatures due to increased molecular activity. The Arrhenius equation illustrates this effect, showing the relationship between temperature and reaction rate.
Temperature calculation also aids in determining products of reactions under specific conditions. Accurate measurements enable chemists to predict equilibrium constants, which depend on temperature. For example, the reaction of ammonium chloride decomposition varies significantly based on temperature changes. This knowledge is essential for both theoretical and applied chemistry.
Role in Climate Science
Climate science relies heavily on precise temperature calculations. Researchers monitor global temperatures to assess climate change impacts. By understanding historical temperature trends, scientists can make informed predictions about future changes. Temperature data is used in models that project climate patterns and identify potential warming effects.
A significant part of climate science is related to temperature anomalies. These anomalies help in understanding variations in the Earthβs climate system. For instance, the difference between current temperatures and long-term averages can indicate significant environmental changes or natural events. Therefore, temperature calculations are vital for climate researchers who seek to mitigate and adapt to changing climate conditions.
Implementations in Engineering
In engineering, temperature calculations are critical for design and manufacturing processes. Buildings, vehicles, and machinery must withstand specific temperature ranges. Engineers use temperature data to select materials that function correctly under expected thermal conditions.
For example, thermal expansion must be considered when designing bridges and railways, as materials expand or contract with temperature changes. In electronics, temperature management is vital for maintaining performance and ensuring safety. Engineers often utilize sensors and controls to monitor and adjust temperature in manufacturing processes. This minimizes defects and enhances the overall quality of products.
Daily Life Applications
Temperature calculation is not limited to scientific fields; it also plays an essential role in daily life. For example, cooking involves precise temperature measurements to ensure food safety and optimal flavor. Understanding when to apply heat and how much is crucial in culinary arts.
In healthcare, temperature monitoring is a routine practice. Body temperature readings can indicate health issues or infections. Thermometers are common tools used in homes and medical settings. Thus, accurate temperature reading is vital in maintaining health and well-being.
In summary, temperature calculation is fundamental across various fields. Its applications extend from intricate scientific research to daily life practices. Understanding this subject is vital for effective decision-making and advancement in many disciplines.
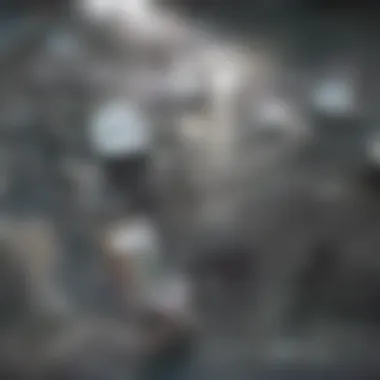
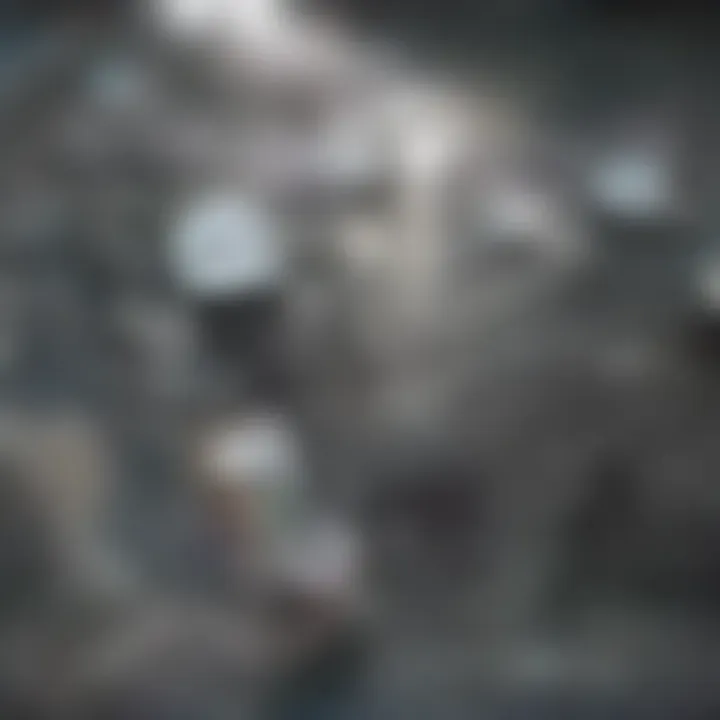
Challenges in Temperature Calculations
Temperature calculation is not merely a straightforward task of reading values from a thermometer. It incorporates various challenges that stem from measurement uncertainties and environmental influences. Understanding these challenges is important as they can significantly affect the accuracy and reliability of temperature measurements and calculations in various applications.
Measurement Uncertainties
In temperature measurement, uncertainty is a crucial factor that can lead to incorrect interpretations of results. This uncertainty may arise from multiple sources, including the limitations of the measuring instruments, human error, and external factors like temperature gradients.
- Instrument Resolution: Not all thermometers have the same precision. For example, a standard mercury thermometer may only provide readings to the nearest degree, while a digital thermometer can offer precision up to two decimal points. This discrepancy can result in errors during calculations, particularly in research where high accuracy is required.
- Calibration Issues: Instruments need regular calibration to maintain their accuracy. If a thermometer is not calibrated correctly, the measured temperature may consistently deviate from the actual value. This situation is often observed in older instruments that havenβt been checked against a known standard in a long time.
- User Error: Even experienced researchers can make mistakes while taking measurements. This can include misreading scales or not allowing enough time for the thermometer to stabilize. Simple oversights like these can introduce significant inaccuracies.
Accurate measurement is not just a technical requirement but fundamental in ensuring that results can be reproduced and reliable.
To mitigate these uncertainties, it is vital to use high-quality equipment and follow protocol for calibration and measurement.
Environmental Influences
Environmental conditions can also pose significant challenges in temperature calculations. The accuracy of measurements can be heavily influenced by a variety of external factors, including:
- Ambient Temperature: The temperature of the environment around the measuring device can affect the readings. For example, if a thermometer is exposed to direct sunlight, it may record a higher temperature than the actual air temperature.
- Surface Contact: When measuring the temperature of a surface, poor thermal contact between the temperature sensor and the object can lead to inaccurate readings. Factors such as surface texture, ambient flows, and even dust can cause discrepancies.
- Humidity and Pressure: Changes in humidity and atmospheric pressure can also influence temperature readings. High humidity may alter the thermal properties of a substance, leading to a skewed measurement, especially in environments such as laboratories where precise temperatures are critical.
In light of these environmental influences, strategies for effective temperature calculation must include controlling external conditions whenever possible. This means conducting experiments in controlled environments or compensating for known variables during calculations.
In sum, overcoming the challenges associated with temperature calculations requires a combination of proper equipment usage, regular maintenance, and awareness of external factors influencing the measurements. Understanding these aspects is essential for ensuring that temperature data remains reliable across various scientific fields.
Recent Advances in Temperature Measurement Technology
The realm of temperature measurement has evolved significantly due to technological advancements. As temperature plays a crucial role in numerous scientific fields, improving measurement techniques is paramount. This section discusses two key areas: digital thermometers and infrared thermometry. Both contribute to more accurate, efficient, and versatile temperature readings.
Digital Thermometers
Digital thermometers have become a staple in both clinical and industrial settings due to their precision and ease of use. These devices employ electronic sensors to provide quick and accurate readings. Unlike traditional mercury thermometers, digital models eliminate the risk of hazardous material leakage and improve safety.
Key Benefits:
- Speed: Digital thermometers typically provide readings in a matter of seconds. This rapid response time is valuable in both medical and laboratory environments.
- Precision: Many digital thermometers are designed with precision sensors, ensuring close to exact readings. This is essential in research settings where even slight temperature variations can affect outcomes.
- Versatility: Digital models come in various forms, including infrared and probe types. This flexibility allows for a wide range of applications, from human medical use to industrial processes.
Considerations when choosing a digital thermometer include battery life, range of measurement, and calibration options. These factors can influence the device's performance and applicability in specific situations.
Infrared Thermometry
Infrared thermometry is another important advancement in temperature measurement. This technique utilizes infrared radiation emitted from an object's surface. It allows for non-contact measurement, which is particularly beneficial in situations where direct contact is impractical or dangerous.
Key Benefits:
- Non-Contact Measurement: Infrared thermometers can measure temperature from a distance. This is crucial in applications such as monitoring electrical equipment or hazardous materials.
- Instantaneous Results: These devices often provide immediate feedback, making them ideal for scenarios that require quick decision-making.
- Wide Range of Applications: Infrared thermometry is employed in diverse fields, from food safety and quality control to HVAC assessments and medical diagnostics.
Nevertheless, users should be aware of potential limitations, such as emissivity variations and the influence of environmental factors on readings. Understanding these aspects is essential for obtaining accurate results.
In summary, recent advancements in temperature measurement technology, particularly digital thermometers and infrared thermometry, have significantly enhanced the ability to monitor and analyze temperature with greater efficiency and accuracy. These advancements are indispensable in various scientific and practical applications.
Ending
In this section, we will summarize the importance of temperature calculation, highlighting its role in various scientific fields and its relevance to practical applications in everyday life. Temperature serves as a fundamental concept in physics and chemistry, influencing both theoretical frameworks and experimental practices. Understanding how to accurately calculate and convert temperatures among different scales is crucial for researchers and practitioners alike, ensuring precise results in experiments, climate modeling, and engineering tasks.
Effective temperature calculation integrates principles of thermal energy with the appropriate methodologies to yield meaningful results. This approach helps to delineate the nuances in measurement uncertainties and environmental influences that may affect the accuracy of results. Recognizing these challenges, as discussed previously, enables professionals to adopt more appropriate techniques and tools for temperature measurement.
Furthermore, the ongoing advancements in technology and methodology ensure that temperature calculations evolve to meet the demands of new scientific inquiries and applications. By fostering a deep understanding of temperature principles and methodologies, we equip ourselves to innovate within our respective fields.
Summary of Key Points
In summation, the key points discussed throughout the article encompass:
- The definition and significance of temperature in scientific contexts.
- Various temperature scales, including Celsius, Fahrenheit, and Kelvin, highlighting their conversion formulas and applications.
- The principles underlying temperature calculations, emphasizing both experimental and calculated methods.
- Application of temperature calculations across various fields, from chemistry to climate science and engineering.
- Challenges within the realm of temperature measurements, considering uncertainties and environmental factors.
- Recent technological advancements made in measuring temperature effectively.
Understanding these key elements enhances not only theoretical knowledge but also practical competencies in temperature measurement and calculation.
Future Directions
Looking forward, ongoing research and technological innovations hold promise in transforming the landscape of temperature measurement and calculations. Several avenues may shape this field:
- Integration of AI and Machine Learning: The use of artificial intelligence can optimize the calibration of temperature sensors, improve data accuracy, and facilitate better predictions in climate modeling.
- Development of Smart Sensors: Innovations in sensor technology are expected to yield more precise, reliable, and faster responses in diverse settings, from industrial applications to daily life.
- Interdisciplinary Approaches: Collaborative efforts among fields such as physics, engineering, and environmental science could lead to comprehensive solutions addressing temperature-related challenges in research and real-world applications.
By focusing on these advancements, future studies will not only build upon existing methodologies but also steer the course of temperature calculation towards greater accuracy and efficiency, ultimately enhancing its applicability across multiple domains.