Transforming Thermal Energy into Electrical Power
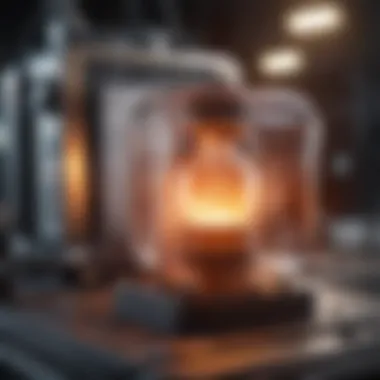
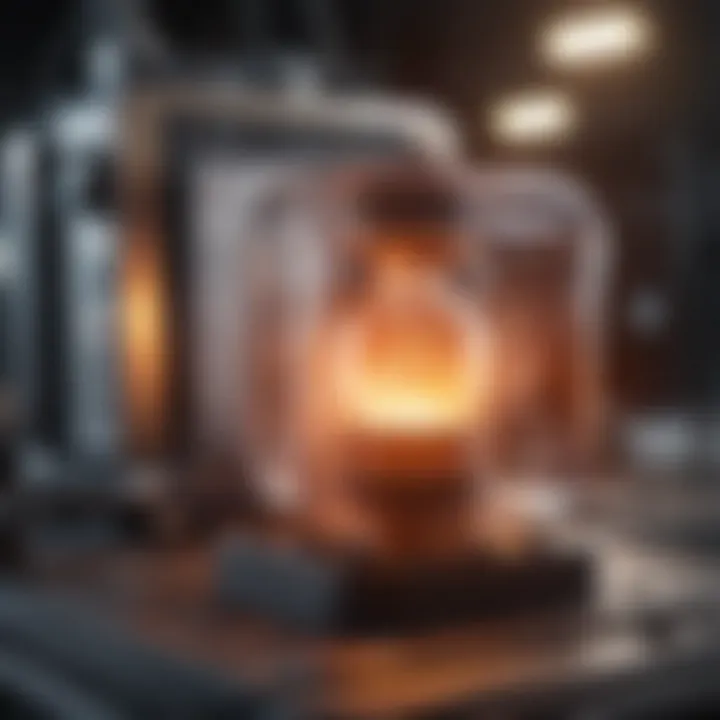
Intro
The conversion of thermal energy into electrical energy is a critical field of study within the realm of energy sciences. This transformation has been gaining prominence, largely due to its implications for sustainable energy solutions. With global energy demands rising, the ability to effectively harness waste heat and convert it into usable electricity presents a significant opportunity. This article will explore the various methods utilized for this conversion, their efficiencies, and the challenges that need to be addressed to optimize these processes for a sustainable future.
Recent Advances
Latest Discoveries
Recent studies have unveiled new materials that enhance the efficiency of thermoelectric generators. Materials like bismuth telluride and skutterudites have been at the forefront, demonstrating improved thermoelectric performance. This is crucial, as higher efficiency means more effective energy conversion from waste heat. New alloys and composites are also emerging, promising significant improvements in the generation of electrical energy from thermal sources.
Technological Innovations
Innovations in heat engine design have diversified the methods available for energy conversion. For instance, the development of micro heat engines is allowing for applications in smaller devices, where traditional engines cannot operate. Additionally, advancements in energy recovery systems are becoming increasingly efficient, effectively capturing and converting excess heat from industrial processes.
"The continuous evolution of materials and technologies holds the key to unlocking the full potential of thermal energy conversion."
Methodology
To understand the advancements in thermal energy conversion, a structured methodology is essential. This involves detailed research design and effective data collection techniques that can illuminate current practices and future directions.
Research Design
Research efforts often focus on experimental setups that allow for comprehensive testing of various materials and processes. Such studies typically involve controlled environments to measure the efficiency of energy conversion methods. Evaluating both thermoelectric generators and heat engines within identical parameters helps create meaningful comparisons.
Data Collection Techniques
Gathering data in this field relies heavily on precise measurement instruments. Techniques such as infrared thermal imaging, thermocouples, and pyrometers are crucial for assessing temperature gradients. This data is essential for optimizing the efficiency of devices designed to convert thermal energy into electrical energy.
Through a thorough exploration of both recent advances and methodologies in this field, it becomes evident that significant progress has been made. The continual evolution of technologies and an ongoing commitment to research will pave the way for greater accomplishments in the electrical energy-generating processes.
Intro to Energy Conversion
Understanding Thermal Energy
Thermal energy is the internal energy present in a system due to the motion of its molecules. The faster the molecules move, the more thermal energy they possess. This form of energy is commonly harnessed through various processes such as combustion, geothermal systems, and solar thermal systems.
Thermal energy plays a vital role in our daily lives and is a major by-product of numerous industrial processes. For example, in power plants, thermal energy generated from burning fossil fuels is converted into electricity. However, not all thermal energy can be efficiently captured, leading to significant wastage. Therefore, a thorough understanding of how thermal energy can be converted is essential for advancing technology and achieving energy efficiency.
Prelude to Electrical Energy
Electrical energy, on the other hand, is the energy caused by moving electric charges. It is one of the most versatile forms of energy and is used to power countless devices and systems worldwide. The generation of electrical energy typically involves the conversion of other forms of energy, such as thermal or mechanical energy.
The infrastructure for electricity production has evolved significantly, with traditional methods involving large power plants. Today, there is a growing interest in decentralized generation methods, such as solar and wind energy. As the demand for cleaner energy solutions rises, understanding the relationship between thermal and electrical energy becomes even more critical.
Both thermal and electrical energy serve as essential components in our energy systems. The efficient transformation of thermal energy into electrical energy is not just a technical challenge; it embodies the larger goal of creating a sustainable and resilient energy future across the globe.
Principles of Energy Conversion
The Laws of Thermodynamics
The laws of thermodynamics are pivotal in energy conversion discussions. They set the framework for understanding system behavior during energy transformations.
- First Law of Thermodynamics (Law of Energy Conservation): This law states that energy can neither be created nor destroyed; it can only be transformed from one form to another. In thermal to electrical conversion, this means that the thermal energy used must equal the sum of electrical energy produced and any losses through heat, sound, or other forms.
- Second Law of Thermodynamics: This law introduces the concept of entropy, stating that energy transformations are not 100% efficient. Some energy is always lost to the surroundings, typically as waste heat. This principle is crucial to consider, as it affects the design of systems like thermoelectric generators and heat engines.
"Energy systems are subject to the inherent limitations set by the laws of thermodynamics. Understanding these limitations enhances our capability to innovate beyond existing technologies."
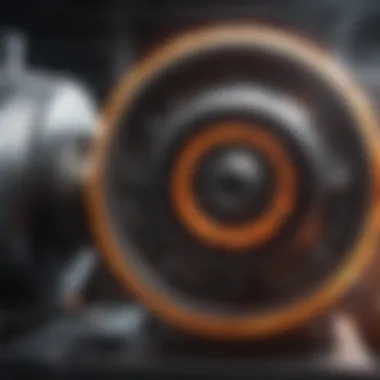
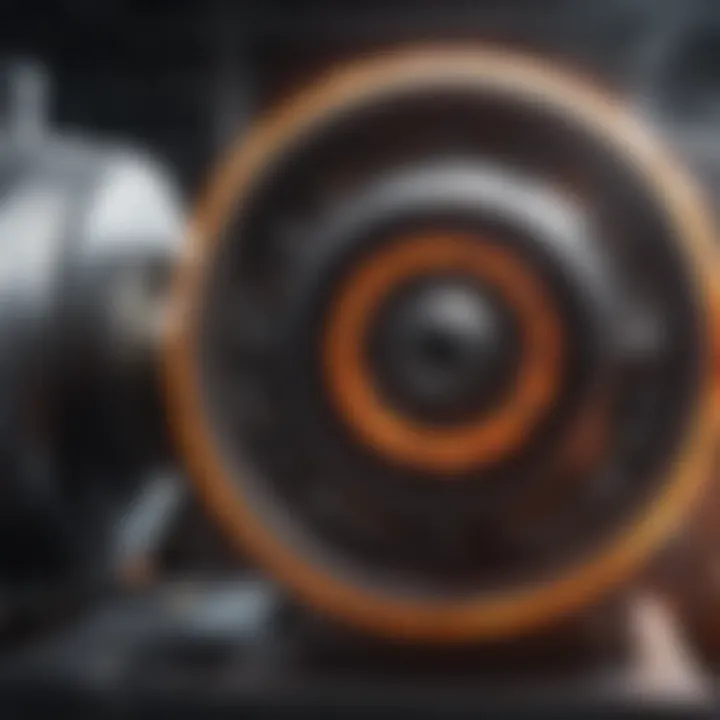
Energy Conservation in Conversions
Energy conservation plays a crucial role in the efficiency of energy conversion methods. When thermal energy is harnessed to generate electricity, it is essential to maximize what can be transformed into usable electrical energy. The following factors are integral:
- Material Properties: Different materials conduct heat and electricity differently. The choice of materials can influence the overall efficiency of the energy conversion process. For example, thermoelectric materials such as bismuth telluride have unique properties that allow for more effective conversion.
- Process Optimization: Effective design involves continuously improving the processes used to convert energy. This can mean better heat exchanges, improved designs for engines, and optimized chemical processes in thermal systems.
- System Integration: Integrating renewable sources of thermal energy, such as solar thermal systems, requires an in-depth understanding of how energy flows within the system. This integration impacts both efficiency and the sustainability of the energy conversion.
By recognizing these principles and considerations, professionals and researchers can develop better systems aimed at converting thermal energy efficiently into electrical energy.
Thermal to Electrical Conversion Techniques
The conversion of thermal energy to electrical energy is an essential area in energy technology. This section explains various techniques used in this conversion process. Understanding these methods is crucial for those involved in energy production, research, and sustainability initiatives. The efficiency and effectiveness of these techniques directly impact our ability to harness energy from thermal sources.
Thermoelectric Generators
Thermoelectric generators (TEGs) play a significant role in converting heat directly into electricity. They operate based on the Seebeck effect, utilizing the temperature difference between two junctions to generate voltage. This feature makes TEGs a favored option in various applications, particularly in waste heat recovery and remote power generation. TEGs are compact and require minimal maintenance, making them a good choice where reliability is essential.
Working Principle
The working principle of thermoelectric generators relies on the relationship between temperature and electric generation. When one side of a thermoelectric material is heated, carriers in the material start to diffuse towards the cooler side. This movement creates a voltage difference, thus generating electrical power. This simplicity is a major advantage, as it does not require moving parts and offers durability. However, the efficiency of TEGs can often be low, which leads to the need for high temperature gradients for optimal performance.
Material Considerations
Material considerations are crucial in TEG performance. The efficiency of thermoelectric materials is largely determined by their thermoelectric figure of merit, known as ZT. Good thermoelectric materials, like bismuth telluride or lead telluride, have a high ZT. Nonetheless, such materials can be expensive and their availability might affect cost-efficiency in large-scale applications. Selecting appropriate materials is essential in balancing performance and cost.
Efficiency Challenges
Efficiency challenges are a significant concern with thermoelectric generators. The typical efficiency of TEGs is around 5-8%, which is much lower compared to conventional energy conversion methods. Additionally, they require high temperature differences to function efficiently, which is not always feasible. Ongoing research focuses on enhancing the efficiency of these devices through better materials and designs, but substantial improvements in this area are still needed.
Heat Engines
Heat engines are another fundamental method of materializing thermal energy into electrical energy. They work by converting thermal energy to mechanical energy, which is then converted to electricity. This method is widely used globally and operates based on the principles of thermodynamics. Heat engines can utilize various heat sources, making them versatile.
Basic Mechanics
The basic mechanics of heat engines involve the intake of heat, expansion of gases, and then work extraction. The process typically includes four stages: intake, compression, power generation, and exhaust. This cyclical process is essential in transforming diverse heat inputs into usable electricity. Although effective, heat engines bear drawbacks, such as being bulkier than other methods and having moving parts, which may raise maintenance concerns.
Types of Heat Engines
Types of heat engines include internal combustion engines, external combustion engines, and steam turbines. Each type employs different mechanisms but shares a common goal of energy conversion. Internal combustion engines power vehicles, while external combustion engines can work with biomass or solar thermal energy. Understanding the characteristics of each type can enhance design and application for specific energy needs.
Performance Metrics
Performance metrics of heat engines are evaluated based on efficiency, thermal efficiency, and power output. The thermal efficiency of a heat engine is often expressed in terms of how well it converts absorbed heat into work. Ideal heat engines have a maximum theoretical efficiency represented by the Carnot efficiency. Real-world engines often fall short of this due to various losses. Assessing these metrics helps determine the suitability of engine types for different applications.
Phase Change Energy Conversion
Phase change energy conversion methods utilize the transition of materials between solid, liquid, or gas phases to generate electricity. This technique capitalizes on the energy released or absorbed during phase changes, such as melting or boiling. These systems can either exploit waste heat or be integrated into renewable energy setups.
Mechanisms of Phase Change
The mechanisms of phase change are critical to this energy conversion strategy. A material that changes phase can absorb large quantities of heat at constant temperature, enabling energy storage and release. This unique ability allows for the capture of energy from thermal resources, turning them into usable electrical power. A challenge lies in finding materials with favorable thermal properties and phase transition characteristics for sustained operation.
Applications in Electricity Generation
Applications in electricity generation using phase change methods include Concentrated Solar Power (CSP) systems and thermal energy storage facilities. CSP systems use mirrors or lenses to concentrate sunlight, heating a substance to produce steam that drives turbines. This approach is popular because it provides clean, renewable energy generation. While effective, initial installation costs can be high, and the availability of sunlight is a limiting factor in certain regions.
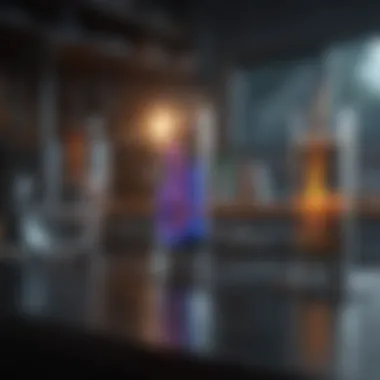
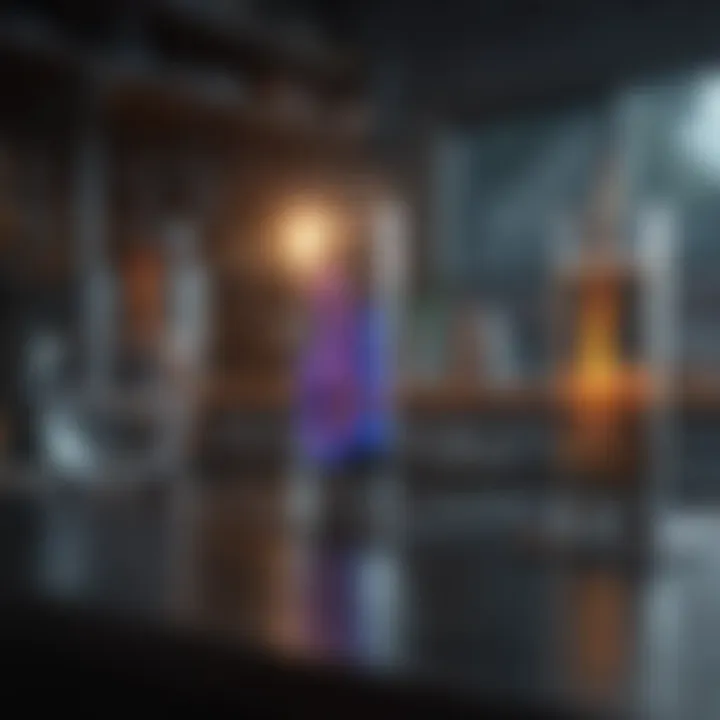
Understanding these thermal to electrical conversion techniques illuminates pathways for advancing energy systems. Each technique brings forth unique benefits and challenges, warranting careful consideration for effective implementation.
Factors Influencing Efficiency
The conversion of thermal energy into electrical energy is a complex process influenced by various factors. Each of these elements plays a crucial role in optimizing efficiency. Understanding these factors can lead to significant advancements in energy conversion technology and enhance sustainability efforts.
Temperature Gradient
Temperature gradient holds a pivotal place in the conversion process. It is the difference in temperature between the heat source and the cold sink. A larger temperature gradient generally enhances the efficiency of heat engines and thermoelectric generators. The second law of thermodynamics indicates that energy transfers are more efficient when there is a substantial differential.
In practical applications, maintaining an optimal temperature gradient can prove challenging. This might require specific materials or improved insulation to prevent heat loss. The efficiency of systems significantly rises with better insulation techniques that limit thermal dissipations, thereby preserving the valuable energy generated.
Material Conductivity
Material conductivity is another key factor that influences energy conversion efficiency. The thermal and electrical conductivity of the materials used directly affects how well these devices can operate. High thermal conductivity materials can transfer heat more effectively, while high electrical conductivity ensures that the generated energy can be efficiently harnessed.
Thermoelectric materials, for instance, require a delicate balance of these properties. Materials like bismuth telluride and lead sulfide have demonstrated promising results in thermoelectric applications, striking an effective balance. However, finding materials that can operate efficiently at various temperature ranges continues to be a significant challenge.
System Design
The design of the energy conversion system greatly influences efficiency. A well-thought-out design considers various aspects such as spatial efficiency, cost, and ease of maintenance. A system designed for optimal fluid dynamics and heat exchange can dramatically increase thermal to electrical conversion.
Moreover, the integration of smart technologies can also enhance efficiency. For example, systems that utilize sensors to monitor real-time performance can adjust parameters to maintain optimal operating conditions. This adaptability can result in significantly less energy waste and improved overall system performance.
"The efficiency of thermal to electrical energy conversion is not solely based on thermodynamic principles but also on material and design innovations."
To summarize, factors influencing efficiency in energy conversion are integral to optimizing performance. Increasing temperature gradients, enhancing material conductivity, and refining system designs are all pathways toward improved energy output. Addressing these challenges equips us with methods to foster sustainable practices and meet our future energy needs.
Real-World Applications
Thermal energy to electrical energy conversion is essential in today's technological landscape. It holds relevance across diverse sectors, shaping how we harness and utilize energy. Understanding these applications helps illustrate how theoretical principles translate into practical use, impacting daily life and industry alike.
Sustainable Energy Solutions
Sustainable energy solutions are pivotal in addressing global energy concerns. Innovations in converting thermal energy to electrical energy play a key role here. Technologies such as solar thermal power plants make use of concentrated solar power. These plants convert sunlight into heat, subsequently generating electricity through steam turbines.
Another promising area is geothermal energy. This method taps into the Earth's internal heat, converting it into usable electrical power. The beauty of geothermal systems lies in their low emissions and minimal environmental impact, aligning perfectly with sustainable practices. Greater implementation of such solutions can significantly contribute to reducing reliance on fossil fuels.
Industrial Applications
Industrial applications of thermal to electrical energy conversion are vast and varied. In manufacturing, heat engines are commonly utilized to enhance efficiency. For instance, large-scale power plants often use steam turbines, effectively converting waste heat from industrial processes into electricity. Such integrations contribute to energy savings and increased productivity.
In addition to traditional means, industries are exploring thermoelectric materials for cooling and energy harvesting. These materials convert temperature differences into electrical power. Industries such as automotive and aerospace are particularly interested in these technologies. The challenge remains to improve the efficiency and affordability of these materials for broader adoption within the sector.
Innovations in Implementations
The field is constantly evolving, and innovations are pivotal for future advancements in thermal to electrical conversion. Researchers are exploring novel materials to enhance the performance of thermoelectric generators. This includes experimenting with nanomaterials and advanced composites that offer increased efficiency.
Another area seeing rapid growth is the integration of Internet of Things (IoT) technologies. Smart monitoring solutions can enhance performance by providing real-time data. This data helps optimize systems, ensuring they operate at peak efficiency while minimizing waste.
"Embracing these innovations is crucial for future-proofing our energy systems."
In summary, the exploration of real-world applications for converting thermal energy into electrical energy reveals its importance across various sectors. From sustainable energy solutions to industrial practices and innovative implementations, the ongoing development in this field signals a promising approach towards achieving greater energy efficiency and sustainability.
Challenges in Thermal to Electrical Conversion
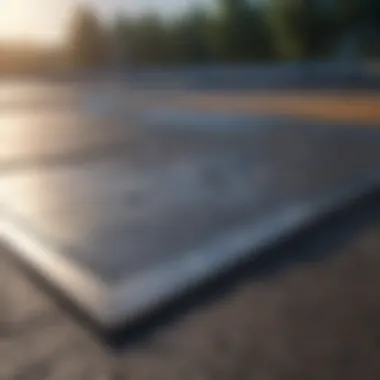
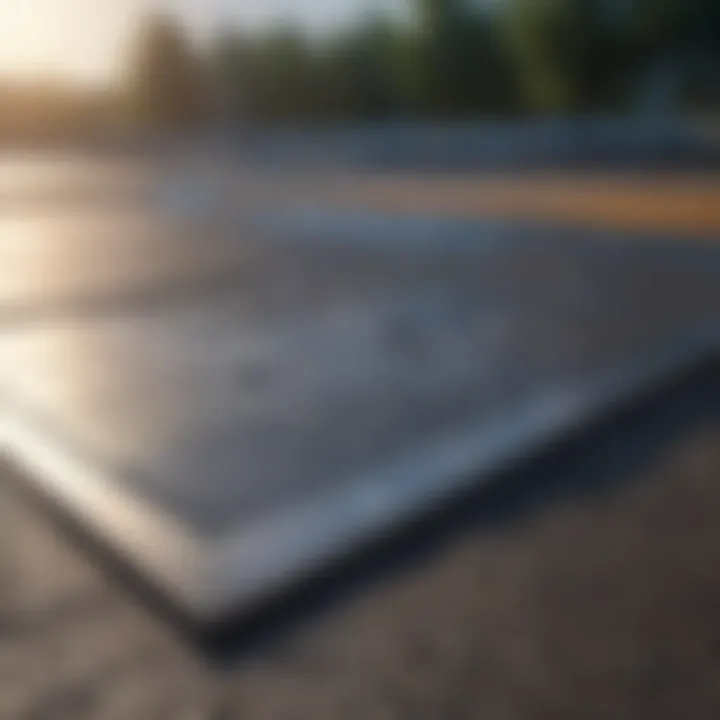
Material Limitations
Materials play a pivotal role in thermal to electrical conversion. Each conversion technique relies on specific materials that can handle temperature variations, conductivity requirements, and mechanical stresses. For instance, thermoelectric generators often utilize bismuth telluride and lead telluride, both of which exhibit good thermoelectric properties. However, the performance of these materials can degrade at high temperatures, leading to efficiency losses.
Additionally, the cost of materials can be a significant barrier. Materials that offer high performance, such as certain alloys or composites, may also be expensive to manufacture or process. Researchers are exploring alternative materials, but discovering options that balance performance, cost, and environmental impact remains a major challenge.
Economic Viability
The economic feasibility of converting thermal energy into electricity is another challenge. High initial investment costs, operating expenses, and maintenance requirements can deter implementation. For industries harnessing this conversion method, return on investment (ROI) is crucial. Convincing stakeholders to adopt these technologies when cheaper, readily available alternatives exist can be difficult.
Moreover, energy markets fluctuate. Tariffs and incentives related to renewable energy impact the competitiveness of thermal-to-electrical systems. Without supportive policies or subsidies, the economic pathway for this technology can be uncertain.
Technological Barriers
Technological challenges in thermal to electrical conversions vary widely. One significant barrier is the efficiency of current technologies. Although advancements such as advanced heat engines and improved thermoelectric materials have been made, overall conversion efficiency remains relatively low in comparison to potential. In many cases, the ideal theoretical efficiency cannot be achieved due to practical limitations.
Furthermore, integrating new technologies into existing energy infrastructures presents complications. Compatibility with conventional systems and the need for skilled personnel to operate and maintain advanced systems are concerns that cannot be overlooked.
"Addressing challenges in thermal to electrical conversion is essential for improving efficiency and fostering sustainable energy solutions."
Moving forward, collaboration between disciplines in research and development remains paramount. Continuous innovation is critical to overcoming these obstacles and realizing the full potential of thermal to electrical conversion.
Future Prospects in Energy Conversion Technology
The future of energy conversion technology is an essential focal point in the contemporary energy landscape. As we develop our understanding of thermal energy transformation into electrical energy, we also unveil a spectrum of possibilities that can reshape industrial applications and consumer practices. Given the increasing emphasis on sustainable energy solutions, the need for efficient energy conversion methods is paramount. This section examines emerging research areas, the potential for sustainable practices, and the global impact of these advancements.
Emerging Research Areas
Research in energy conversion is rapidly evolving, influenced by the need for sustainable energy sources. Current studies focus on several key areas:
- Advanced Materials: The development of new materials can significantly enhance the efficiency of thermoelectric generators. Researchers are exploring nanostructured materials that provide improved thermal and electrical conductivity, thereby maximizing energy conversion efficiency.
- Hybrid Systems: Integrating various energy conversion technologies can create hybrid systems that utilize strengths of each method. For example, combining solar thermal and thermoelectric processes can boost overall system efficiency.
- Waste Heat Recovery: The recovery of waste heat from industrial processes is being closely examined. Techniques that capture and convert this lost energy into electricity can substantially improve energy efficiency.
Research efforts in these areas could drive significant improvements in thermal to electrical energy conversion, potentially leading to innovative approaches and technologies.
Potential for Sustainable Practices
Addressing climate change relies on sustainable practices in energy generation. Future energy conversion technologies hold vast potential to promote sustainability through:
- Reduced Carbon Footprint: By enhancing energy conversion efficiency, emissions can be minimized. This shift not only helps in adhering to environmental regulations but also contributes to corporate responsibility initiatives.
- Decentralized Energy Systems: Technologies such as portable thermoelectric generators can facilitate decentralized energy solutions, reducing the need for extensive power networks. This is especially important in remote areas where energy access is limited.
- Integration with Renewable Sources: Future energy systems are likely to integrate seamlessly with renewable energy sources. For instance, using solar thermal energy with advanced thermoelectric materials can provide a consistent energy supply.
The pursuit of sustainable practices through advanced energy conversion methods can propel society towards a greener future.
Global Energy Impact
Adopting improved energy conversion technologies will have profound implications on a global scale:
"Efficient thermal energy conversion can reduce our reliance on fossil fuels, thereby mitigating environmental impacts on a global scale."
- Energy Security: Enhanced efficiency in energy conversion contributes to energy security. Countries can rely less on imported fuels, leading to a more stable economy.
- Influence on Global Policies: As nations adopt these technologies, shifts in policy may emerge, pushing governments to invest in research and infrastructure conducive to advanced energy systems.
- Job Creation: New industries surrounding advanced energy conversion technologies can generate job opportunities, contributing positively to economic growth.
Understanding and investing in future prospects for energy conversion technology offers a way to align economic growth with environmental responsibility, positioning societies for success in tomorrow's energy landscape.
Finale
Summary of Key Points
- Thermal to electrical energy conversion involves methodologies like thermoelectric generators and heat engines.
- Efficiency is influenced by multiple factors including temperature gradients and material conductivity.
- Real-world applications span sustainable energy solutions to industrial uses, emphasizing the versatility of these conversion techniques.
- Challenges such as material limitations and economic viability must be addressed to enhance the process continually.
Importance of Continued Research
Continuing research in energy conversion technology is required for several reasons. Firstly, innovation helps overcome the current limitations, enhancing existing methods or perhaps creating new ones entirely. Secondly, the burgeoning need for clean energy sources makes it imperative to explore sustainable practices. The transition from fossil fuels to more renewable energy sources is not just preferred but necessary for environmental and economic sustainability. Furthermore, understanding this energy transformation significantly impacts global energy policies, leading to more effective strategies for energy distribution and consumption.