Understanding Antibodies: Structure and Function
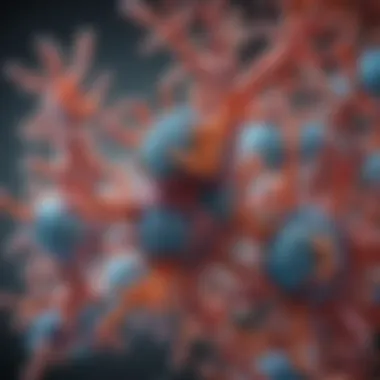
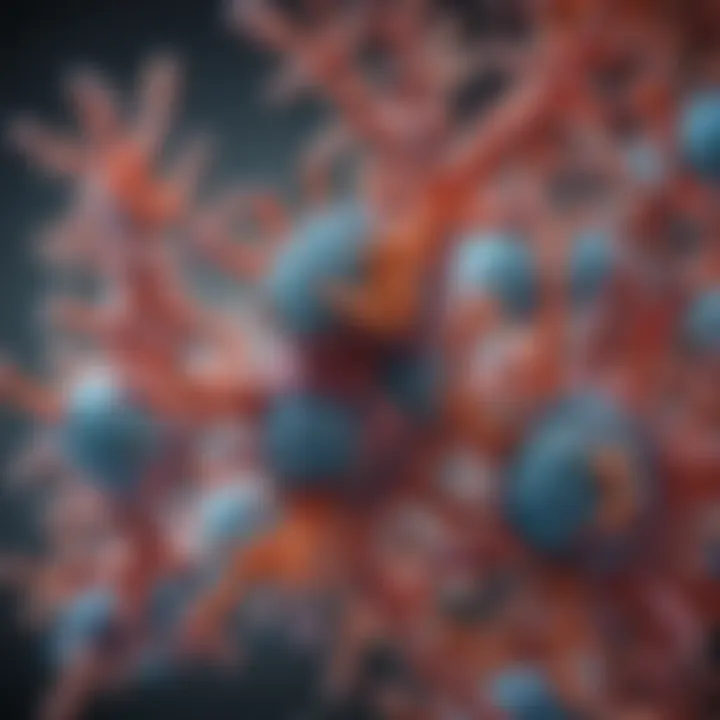
Intro
Antibodies are not just mere proteins; they are complex structures that play a pivotal role in our immune system. When our body encounters pathogens, these tiny warriors spring into action, identifying and neutralizing threats like viruses and bacteria. But what exactly makes up these remarkable molecules? Understanding their composition is fundamental to grasping techniques in medical research and therapies that leverage these proteins for various applications.
In this exploration, we will dig into the molecular architecture of antibodies, examining how components such as amino acids, chains, and domains contribute to their overall functionality. The intricacies behind their formation, variations, and the latest advancements in antibody research will be laid out in a coherent manner, aiming to enlighten students, researchers, educators, and professionals alike.
Recent Advances
The field of antibody research has witnessed significant growth in the past few years, driven by constant innovations and breakthroughs.
Latest Discoveries
Recent studies have shed light on the unique structural features of antibodies that aid in their function. For instance, researchers have discovered that the flexibility of certain regions in antibodies optimizes their ability to bind with antigens—those harmful invaders. This flexibility stems from the extraordinary arrangement of amino acids that form the antibody chains, leading to a more robust immune response.
Furthermore, advancements in understanding the glycosylation patterns of antibodies have opened new doors in medical therapies. These sugar modifications, found on antibodies, can influence their efficacy and how long they survive in circulation. By manipulating these patterns, scientists aim to enhance the therapeutic potential of monoclonal antibodies used in various treatments, such as cancer and autoimmune diseases.
Technological Innovations
Innovations in technology have revolutionized the way we study and produce antibodies. Techniques like next-generation sequencing (NGS) and phage display libraries enable researchers to rapidly identify antibodies with high specificity and affinity. These methods are critical in discovering new therapeutic antibodies tailored for individual patient needs, making treatments more personalized and effective.
Methodology
A detailed exploration of antibodies necessitates a rigorous methodology to ensure reliability and accuracy in findings.
Research Design
Most antibody studies are designed to assess both structural characteristics and functional capabilities. Researchers might utilize X-ray crystallography to visualize three-dimensional structures, which helps in understanding how antibodies interact with antigens. This structural insight is invaluable in drug design and development, pushing forward the agenda of precision medicine.
Data Collection Techniques
Data collection in antibody research can encompass a variety of techniques:
- Enzyme-linked immunosorbent assay (ELISA) to quantify antibodies in samples.
- Flow cytometry for analyzing the physical and chemical characteristics of cells that produce antibodies.
- Mass spectrometry to analyze protein structures and identify post-translational modifications such as glycosylation.
Each technique provides a piece of the puzzle, helping to construct a comprehensive understanding of antibody composition and function.
As we navigate through the detailed components of antibody structure, including chains and domains, we will uncover the vital roles each plays in the immune response. By mapping out these intricacies, we can appreciate how advancements in research are paving the way for enhanced medical therapies and diagnostics.
Prologue to Antibodies
Antibodies are vital components of the immune system, acting as sentinels that detect and respond to foreign invaders like bacteria and viruses. Understanding their composition provides insight into how the body protects itself from disease. This section aims to shed light on the foundational elements of antibodies, emphasizing their significance in immunology and medicine.
Antibodies, also known as immunoglobulins, play a crucial role in our immune response. Their structure allows them to recognize specific antigens, marking pathogens for destruction. In this dense realm of biological complexity, these proteins exemplify not only how the body identifies harm but also how it repairs itself following an injury or infection.
What Are Antibodies?
Antibodies are proteins produced predominantly by a type of white blood cell known as B cells. Their basic purpose is to bind to antigens—unique molecules found on the surface of pathogens. By doing so, antibodies prevent the pathogens from causing further harm. The antibody-antigen interaction is often likened to a lock and key, where the specific shape of the antibody allows it to fit perfectly with its corresponding antigen.
Structurally, antibodies are composed of four polypeptide chains: two heavy chains and two light chains. This configuration creates a Y-shaped molecule that enhances functionality. The precision of the antibody's binding ability is derived from its unique structure, crafted through a complex genetic recombination process.
The Role of Antibodies in the Immune System
Antibodies serve multiple functions within the immune system, and some of those include:
- Neutralization: They bind to pathogens and neutralize their harmful effects.
- Opsonization: This involves marking pathogens for destruction by other immune cells, like macrophages.
- Activation of Complement System: Antibodies can initiate a complement cascade, further amplifying the immune response.
"Antibodies not only neutralize pathogens but also collaborate intricately with other components of the immune system to ensure a robust response to infections."
The variety and adaptability of antibodies allow the immune system to respond to a wide range of threats. Each antibody can specifically recognize and bind to a unique antigen, providing a tailored defense mechanism. Understanding how they function deepens one’s grasp of immunity and the body’s defense strategies, which is essential for fields ranging from medicine to biomedical research.
In summary, antibodies are more than just proteins; they are the frontline warriors of the immune system. With their multifaceted roles and remarkable specificity, they underscore the complexity and efficiency of biological defense mechanisms.
Molecular Structure of Antibodies
The molecular structure of antibodies is a cornerstone of understanding their role in the immune response. By delving into the intricate design of these molecules, we uncover how their composition leads to functionality. Each component, from chains to regions, plays a pivotal role in the antibody’s ability to recognize and neutralize various pathogens. As antibodies type up the first line of defense, their structural design is not merely decorative; it directly influences their effectiveness in immune response, which is crucial for the development of effective vaccines and treatments.
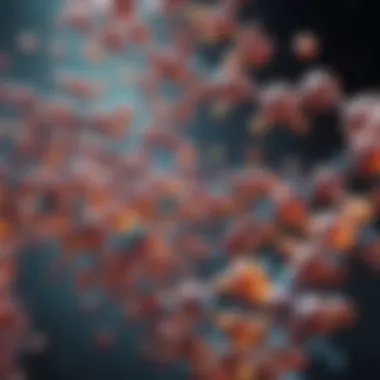
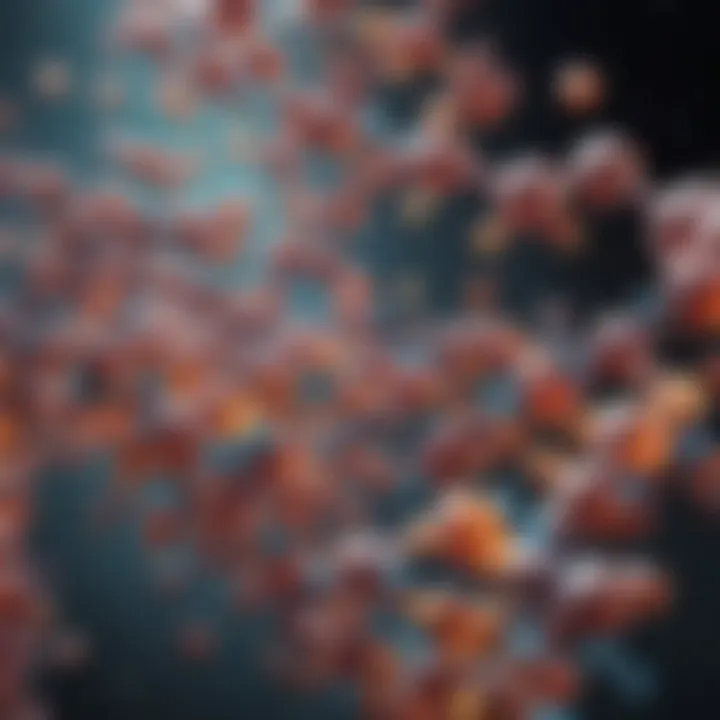
Basic Structure: Heavy and Light Chains
Antibodies are complex proteins with a basic structure that consists of two types of chains: heavy and light. Each antibody, or immunoglobulin, is shaped like a Y, with the arms of the Y composed of light chains and the trunk made of heavy chains. The difference in their lengths and structures is essential for the overall function of the antibody.
- Heavy Chains: These chains are the backbone of the antibody, contributing significantly to size and the specific class of immunoglobulin, such as IgG or IgM. They are larger and provide the structural rigidity necessary to withstand various conditions within the body.
- Light Chains: Lighter in weight, these chains define the location where antibodies bind to antigens. Each immunoglobulin has two light chains, which can be of two types (kappa and lambda) that, while not necessary for the antibody’s function, can influence how the immune system reads the antibody.
The individual units of heavy and light chains are held together by non-covalent interactions and disulfide bonds, creating a stable structure. Each antibody's unique sequence of amino acids gives it the specificity to bind to a particular antigen, making the structure of these chains crucially significant in the immune response.
Variable and Constant Regions
Within the structure of antibodies lie two distinct regions: variable and constant regions. Both of these serve critical roles, but they are quite different in function.
- Variable Regions: Located at the tip of the 'Y', the variable regions are where antibodies recognize and bind antigens. These areas exhibit a high level of diversity due to the genetic rearrangements that occur during B cell development. Essentially, this variability allows the immune system to adapt and recognize countless different pathogens. The specificity in this part of the antibody is what helps in distinguishing between a virus and a bacterium.
- Constant Regions: The rest of the antibody, below the variable regions, is known as the constant region. This area has less variability and determines the class of the antibody (IgG, IgA, etc.). The constant region plays a role in mediating immune responses, including opsonization and complement activation.
Thus, the dynamic between variable and constant regions is crucial. While the variable region gives the antibody its unique specificity, the constant region indicates how the immune system will interact with the pathogen.
The Role of Disulfide Bonds
Disulfide bonds are crucial players in maintaining the integrity of antibody structure. These covalent bonds form between sulfur atoms of cysteine residues located in the heavy and light chains. Their role includes:
- Stability: By linking the chains together, disulfide bonds create a strong foundation that helps the antibody maintain its shape even under various physiological conditions.
- Conformation: They are essential for achieving the correct folding of the antibody, which is vital for its function. An incorrectly folded antibody won't bind to its antigen, rendering it ineffective.
“Without the proper conformation, the efficacy of antibodies to neutralize threats diminishes significantly.”
Overall, understanding the molecular structure of antibodies provides greater insight not only into how these proteins function but also into how they can be manipulated for therapeutic purposes, highlighting the intricate interplay between structure and function in the immune response.
Amino Acids: Building Blocks of Antibodies
The significance of amino acids in the formation of antibodies cannot be overstated. These organic molecules serve as the fundamental building blocks, making up the intricate structure of antibodies and influencing their functionality. Without a deep understanding of amino acids, one cannot fully appreciate how antibodies operate within the immune system.
Antibodies are primarily composed of two types of protein chains: heavy chains and light chains. These chains are further made up of sequences derived from various amino acids. Each amino acid contributes unique properties, influencing everything from the shape of the antibody to its ability to bind with antigens. For instance, variations in amino acid sequences lead to diverse antibody specificities, which are crucial for the immune response.
Moreover, the arrangement of amino acids dictates the three-dimensional conformation of the antibody, which is essential for its biological activity. When antibodies encounter their corresponding antigens, the unique binding sites created by these amino acids enable a tailored response, allowing our immune system to fend off countless pathogens effectively.
Types of Amino Acids Involved
A diverse range of amino acids can be found within antibodies, but certain types play especially vital roles:
- Hydrophobic Amino Acids: These amino acids are crucial for maintaining the overall structure of proteins. Examples include leucine and isoleucine, which help stabilize the protein’s core.
- Polar Amino Acids: Amino acids like serine and threonine are involved in the antibody's interaction with other molecules due to their ability to form hydrogen bonds.
- Charged Amino Acids: Positively charged amino acids, such as lysine and arginine, often participate in the binding with negatively charged regions of antigens, facilitating specific interactions critical for immune response.
- Aromatic Amino Acids: These include tyrosine and phenylalanine. Their unique side chains can interact with other aromatic compounds, playing a part in the protein's stability and specificity.
When considering these types, it's clear the diversity of amino acids bolsters a vast array of functional capabilities of antibodies, tailoring their responsiveness to a myriad of pathogens.
Peptide Bonds and Their Formation
Peptide bonds serve as the connectors between amino acids, forming the backbone of any protein structure, including antibodies. In simple terms, a peptide bond is a covalent bond that links the amino group of one amino acid to the carboxylic group of another. This process is facilitated through a dehydration reaction, where a water molecule is released.
This bond formation occurs during protein synthesis in ribosomes and involves ribosomal RNA and various enzymes. As amino acids are strung together, they form polypeptides, which eventually fold into specific structures that grant antibodies their unique functionality.
"The formation of peptide bonds is fundamental in constructing the diverse arsenal of antibodies, paving the way for the specificity and binding capacity that the immune system requires to function effectively."
Understanding how peptide bonds are formed and the role they play in protein structure is crucial for appreciating how antibodies can distinguish between multiple pathogens. The very structure determined by the peptide bonds allows the immune system to generate a response that is not only prompt but also tailored to eliminate a wide range of invading microorganisms.
Antibody Classes and Their Distinctions
Antibodies, the vigilant guardians of our immune system, can be classified into several distinct classes. This classification is deeply significant because it highlights their various functions and capabilities. In understanding these categories, we can better appreciate how the immune system tailors its responses with precision. Each class of antibody plays a unique role, confronting diverse threats in the body — from simple invaders to more complex pathogens.
The major classes of antibodies include Immunoglobulin G (IgG), Immunoglobulin A (IgA), Immunoglobulin M (IgM), Immunoglobulin E (IgE), and Immunoglobulin D (IgD). These classes differ in their structure, function, and location within the body, each essential for immune response and function.
IgG, IgA, IgM, IgE, and IgD
The five primary classes of antibodies serve distinct roles.
- IgG: This predominant antibody class makes up about 75-80% of serum antibodies. It is paramount in secondary immune responses, efficiently neutralizing pathogens and facilitating opsonization. IgG can traverse the placenta, providing passive immunity to the fetus, a significant perk during early development.
- IgA: Found in mucosal areas, like the gut and respiratory tract, IgA plays a crucial preventive role against infections in these regions. It also exists in bodily secretions like saliva and breast milk, highlighting its importance in safeguarding infants.
- IgM: This is the first antibody type produced in response to an infection. With its pentameric structure, it can effectively agglutinate pathogens and activate the complement system, serving as an initial line of defense before the body ramps up production of IgG.
- IgE: Although present in low concentrations, IgE is key to mediating allergic reactions. It binds to allergens and activates mast cells, causing the release of histamines, which contribute to allergic symptoms.
- IgD: The least understood class, primarily found on the surface of B cells, IgD plays a role in the initiation and regulation of B cell activation. Its specific function remains somewhat elusive, but it likely serves as an immune system marker.
Functional Differences Among Classes
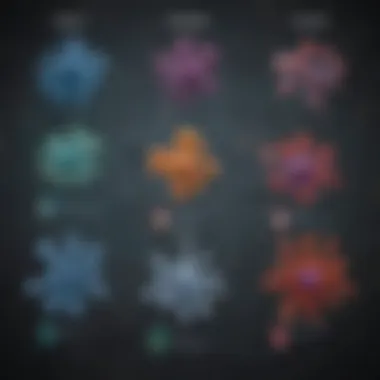
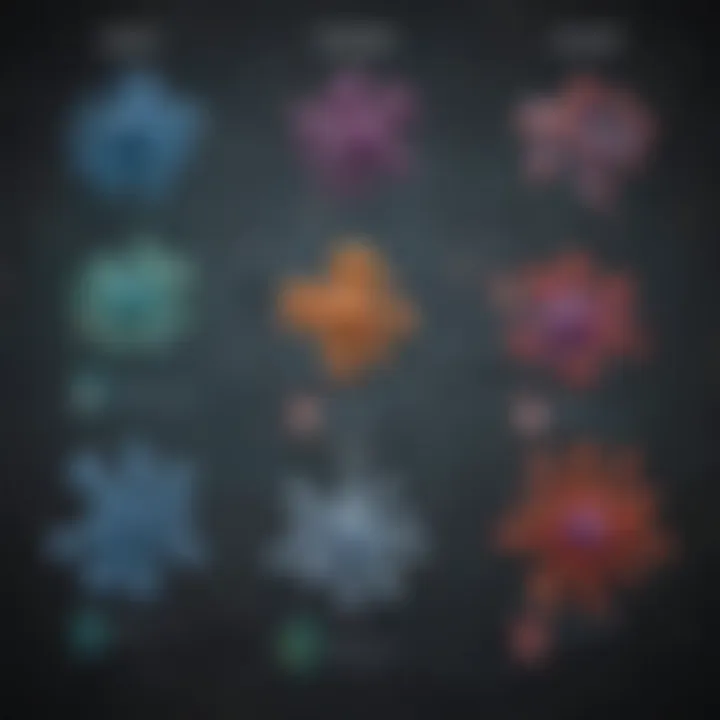
Understanding the functional differences among antibody classes enriches our grasp of how the immune system operates.
- Immune Response Timing: IgM is essential in the early stages of an immune response, providing the initial defense, while IgG's presence indicates a memory response, prompting a faster reaction during subsequent exposures.
- Location and Concentration: IgA dominates mucosal immunity, protecting entry points, unlike IgE, which binds to parasites and triggers local inflammation, while IgG is predominantly found in serum, efficiently circulating throughout the body.
- Versatility: IgG’s ability to cross the placenta and its presence in various fluids makes it versatile, whereas IgE's specialized role is more focused, offering protection against specific pathogens and allergens.
Understanding these distinctions is not simply academic; it translates directly to applications in medical research and therapeutic interventions. Each class of antibody presents unique opportunities for vaccine development, understanding allergic responses, and designing monoclonal antibodies for targeted treatments.
As research advances, the insight into antibody classes not only informs basic immunology but also opens doors for innovative clinical therapies, ultimately enhancing health outcomes for many.
Production and Maturation of Antibodies
The journey of antibodies doesn't merely start at their formation; it involves a complex cascade of events that ensure they are appropriately tailored to combat pathogens. Understanding the production and maturation of antibodies is essential for grasping how our immune system defends the body against infections. The intricate mechanisms guiding these processes allow B cells, the primary players in antibody production, to generate highly specialized antibodies that recognize and eliminate invaders effectively.
B Cells and Their Role
B cells, a type of lymphocyte, are the backbone of antibody production. They originate from hematopoietic stem cells in the bone marrow, but their role matures in a well-orchestrated manner. Once they differentiate, each B cell eventually develops a unique receptor — the B cell receptor (BCR). This receptor is remarkably pivotal because it dictates which antigen the B cell can bind to.
Once matured, B cells circulate through the lymphatic system and peripheral blood, patrolling for foreign antigens. When they encounter a pathogen, such as a virus or bacterium, the specific B cell that recognizes that antigen is activated. This activation prompts a myriad of responses:
- Proliferation: The activated B cell begins to divide, creating a clone of identical cells.
- Differentiation: Some of these clones become plasma cells, realizing their potential for antibody secretion, while others remain as memory B cells.
- Antibody Secretion: Plasma cells churn out vast quantities of antibodies that are released into the bloodstream to seek out and neutralize pathogens.
To sum it up, B cells are not just about creating antibodies; they are essential for adapting the immune response to diverse and evolving threats. Their ability to undergo somatic hypermutation allows for refining the affinity of antibodies, ensuring that the body can mount an effective response against the multitude of pathogens.
Clonal Selection and Expansion
Clonal selection is an elegant process underpinning the immune response. When a B cell, equipped with a unique BCR, meets its matching antigen, it sets off a cascade known as clonal expansion. This is key to scaling up the immune response quickly and efficiently.
Here’s how the clonal selection process unfolds:
- Antigen Encounter: Only B cells with BCRs specific to the pathogen's antigen will engage and trigger an immune response.
- Activation Signaling: Following antigen binding, additional signals from T helper cells are crucial. These signals are essential for full activation, especially if the antigen is a protein.
- Clonal Expansion: Once fully activated, the B cell undergoes rapid division. Each division generates a clone that can produce the same specific antibody. This creates a massive army of identical B cells.
- Differentiation: Ultimately, a subset of cells will differentiate into memory B cells, which persist long after the infection is resolved, ready to respond more swiftly should the same pathogen appear again.
The efficiency of clonal selection and expansion ensures that the body can tackle infections head-on. It ’s like having a highly specialized team of soldiers, each trained for a specific foe, ready to leap into action. The ability to create diverse antibodies rapidly is paramount in our constant battle against infectious diseases.
"In the grand symphony of immune response, B cells and their antibodies play the lead solo, conducting the immune orchestra to eliminate threats effectively."
In summary, the production and maturation of antibodies hinge on the intricate roles of B cells and the polymorphic nature of clonal selection. These mechanisms not only provide immediate defense but also establish a memory system that enhances the speed and potency of future responses.
Antibody Diversity and Specificity
Antibody diversity and specificity are critical aspects of the adaptive immune response. They determine how effectively antibodies can recognize and bind to a wide array of pathogens, from viruses to bacteria. This section elucidates the mechanisms that generate such diversity and the principles of specific binding, which are pivotal in maintaining effective immune surveillance.
Mechanisms Behind Antibody Diversity
At the heart of antibody diversity lies a fascinating biological process known as V(D)J recombination. This complex mechanism takes place in the bone marrow, where B cells undergo several rounds of shuffling segments of DNA that encode for the variable regions of antibodies. These regions are responsible for binding antigens—those pesky invaders like viruses or toxins.
In essence, the random combination of gene segments creates a vast library of unique antibodies.
There are three main ways this diversity is achieved:
- Somatic recombination: This is the initial phase where randomly selected gene segments, termed Variable (V), Diversity (D), and Joining (J), are pieced together. This iterative process can result in billions of potential combinations that contribute to the antibody repertoire.
- Somatic hypermutation: After initial activation, B cells can further enhance their specificity through somatic hypermutation. In this process, mutations in the variable region of the antibody genes occur at an elevated rate, allowing for fine-tuning of the antibody’s affinity for its target.
- Class switch recombination: While not directly related to the creation of diverse binding sites, class switch recombination allows B cells to switch the constant region of the antibody they produce, thus changing the function of the antibody without altering the specificity for the antigen.
These mechanisms combine, producing a nearly limitless array of antibodies tailored to recognize countless antigens. This diversity is crucial because it helps ensure that our immune system can potentially respond to a large variety of pathogens.
Affinities and Specific Binding
The concept of affinity is fundamental to understanding how antibodies interact with antigens. Affinity refers to the strength of the interaction between a single binding site of an antibody and an antigen. Higher affinity means that the antibody binds more tightly to the antigen.
Antibodies demonstrate specificity, which means they preferentially bind to particular antigens based on the unique shape and chemical properties of their binding sites. The relationship between the structure of an antibody and its binding specificity is a complex dance of molecular features that allows for:
- High selectivity: This ensures that antibodies can differentiate between similar structures. For instance, they can distinguish between a harmless molecule and a potential pathogen, preventing unnecessary immune responses.
- Discriminating common and rare antigens: The ability to bind specific antigens while ignoring others is vital in preventing autoimmune responses, where the immune system mistakenly targets the body’s own tissues.
The affinity of an antibody for its target can also influence the effectiveness of vaccines and therapeutic monoclonal antibodies. This is why researchers are increasingly focused on leveraging these concepts in the engineering of more effective antibodies for clinical use.
As research continues into the mechanisms that underpin this diversity, exciting discoveries could lead to novel treatments and further advances in immunology.
Clinical Relevance of Antibodies
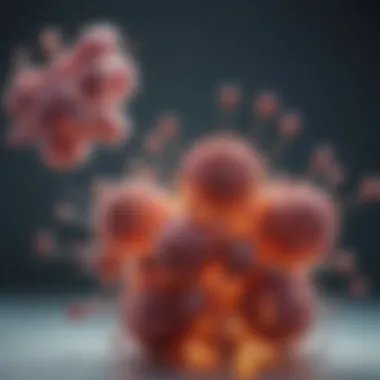
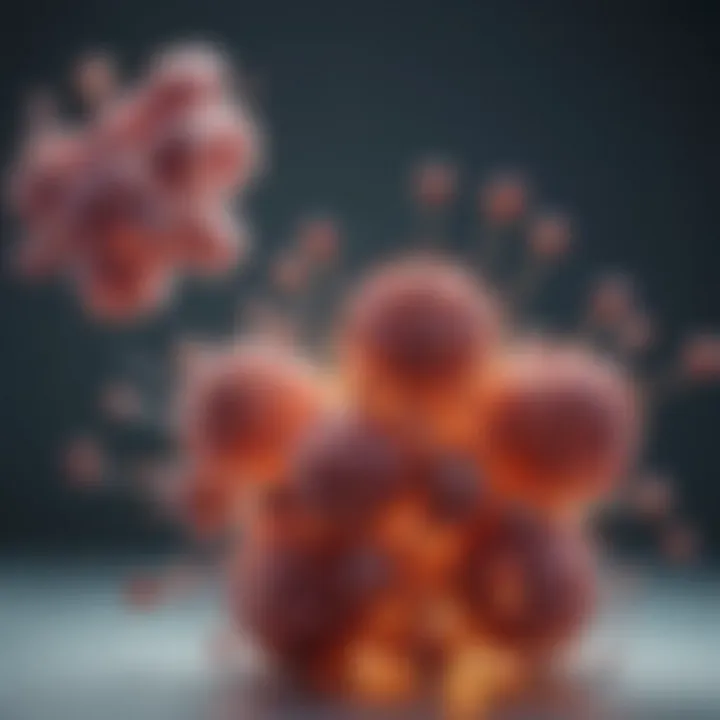
Antibodies occupy a central role in modern medicine and research, transcending their biological function to become powerful tools in clinical applications. Understanding their clinical relevance reveals the breadth of their utility and the implications for health and disease management. This section delves into three major facets: their use in vaccines, their functionality in diagnostic procedures, and the emergence of therapeutic monoclonal antibodies.
Use in Vaccines
Vaccination is one of the most crucial public health achievements, and antibodies play a pivotal role in this process. When a vaccine is administered, it introduces a harmless portion of a pathogen (like proteins or inactivated viruses) into the body. This stimulates the immune system, prompting the production of antibodies that are specific to these antigens.
The antibodies generated provide a tailored defense against future encounters with the actual pathogen. A clear example of this is the influenza vaccine; year after year, changes in the circulating virus require updates in the vaccine formulation to ensure that the antibodies elicited remain effective against new strains. This adaptive aspect preserves public health by curtailing outbreaks.
"Vaccines are like a rehearsal for your immune system, preparing it for when the real performance arrives."
Beyond personal health, the collective immunity gained through vaccination (known as herd immunity) is vital. It protects vulnerable populations who may not be eligible for vaccines. This dual impact underscores the essential role antibodies play in immunization strategies.
Antibodies in Diagnostic Procedures
Diagnostic tests utilizing antibodies have transformed the way diseases are detected and monitored. Several types of assays, such as enzyme-linked immunosorbent assay (ELISA) and immunofluorescence, rely on the specificity of antibodies to identify antigens. This specificity allows for the detection of diseases ranging from infections to various cancers.
Consider a test for HIV; antibodies produced in response to the virus can be detected in blood samples, confirming infection. Such tests are crucial for early diagnosis, which can significantly improve treatment outcomes. Furthermore, innovations like point-of-care testing leverage antibodies for rapid results, making diagnostics more accessible.
In laboratory settings, antibodies also assist in research to explore disease mechanisms, paving the way for new treatments and understanding complicated biological pathways. Their versatile applications in diagnostics cannot be overstated, as they are integral in the timely identification of health issues.
Therapeutic Monoclonal Antibodies
The landscape of treatment protocols across various conditions, especially cancer and autoimmune disorders, has been revolutionized by monoclonal antibodies. These lab-generated antibodies are designed to bind specifically to targets, such as malignant cells or inflammatory proteins, disrupting the processes leading to disease.
For instance, Trastuzumab, used in the treatment of HER2-positive breast cancer, exemplifies this therapeutic approach. It binds to the HER2 protein on cancer cells, inhibiting their growth and signaling the immune system to eliminate them. This precision improves patient outcomes and minimizes damage to healthy cells, contrasting traditional therapies like chemotherapy.
Developments in biopharmaceuticals further underscore the potential of monoclonal antibodies. Targeting unique pathways or molecules has led to personalized therapies, tailoring treatments to the genetic makeup of an individual's cancer.
Emerging Research and Future Directions
Emerging research on antibodies signifies a turning point in our understanding of their composition and function. As the complexity of the immune system unfolds, innovative approaches and scientific inquiries are leading to breakthroughs that could have profound impacts on health care and therapeutic strategies. The ongoing investigation into antibody engineering is shaping the future of immunology and redefining avenues for treatment.
Advances in Antibody Engineering
Antibody engineering is at the frontier of biotechnological achievement. Researchers are utilizing techniques like recombinant DNA technology and phage display to design antibodies with enhanced specificity and affinity. By creating humanized antibodies, which are tailored to reduce immunogenicity, scientists aim for better outcomes in treatments.
One notable advance is the development of bispecific antibodies, which can bind to two different epitopes simultaneously. This capability opens up a treasure trove of possibilities for targeted therapy. For instance, in cancer treatment, bispecific antibodies can redirect T cells to cancer cells, effectively guiding the immune response to where it’s most needed.
Moreover, the use of nanobodies, derived from camelids, presents another promising direction. These smaller, single-domain antibodies retain their functionality while offering advantages such as easier production and improved tissue penetration. Their unique structure allows for enhanced targeting of antigens, making them prime candidates for diagnostic and therapeutic usage in complex diseases.
"The evolution of antibody engineering promises to unlock new horizons in personalized medicine and improve patient outcomes in an array of conditions."
Potential for New Therapeutics
Looking toward the horizon of antibody research, the potential for new therapeutics is both exciting and vast. Enhanced understanding of antibodies can lead to innovative treatments for diseases that previously lacked effective options. For example, research into monoclonal antibodies has revolutionized treatments for conditions like rheumatoid arthritis and certain cancers. With the capability to inhibit pathological mechanisms, these antibodies serve as targeted therapies, reducing side effects and increasing efficacy.
Emerging therapies also include the promises of vaccines coupled with antibody-based interventions. As seen in recent developments, combining these approaches can improve immune responses significantly. For instance, mRNA vaccine technology, popularized during the COVID-19 pandemic, may also inform antibody development for other infectious diseases and even cancer.
Another avenue worth mentioning is the potential for antibody-drug conjugates (ADCs). These allow antibodies to deliver cytotoxic agents directly to tumor cells, thus minimizing collateral damage to healthy tissues. Research in this area is critical, as it offers a pathway toward more effective cancer therapies with fewer adverse effects.
Collectively, the advances in antibody engineering and the push for new therapeutics underscore a pivotal shift in medicine. The integration of cutting-edge science and innovative thinking is instrumental in overcoming challenges and enhancing the effectiveness of antibody-mediated treatments. As we forge ahead, continuing research remains crucial in unlocking the full potential of antibodies in healthcare.
Epilogue
In wrapping up this in-depth exploration of antibodies, it becomes clear that understanding their composition is crucial not only for grasping how they function but also for appreciating their impact on health and disease. The interplay of heavy and light chains, combined with the complex architecture of variable and constant regions, creates a system that is remarkably adaptable. This adaptability is what allows antibodies to recognize and neutralize a vast array of pathogens.
Summary of Antibody Composition and Function
Antibodies are primarily proteins constructed from unique sequences of amino acids. These molecules can be broadly categorized into five distinct classes—IgG, IgA, IgM, IgE, and IgD—each tailored to fulfill specific roles within the immune system.
- IgG is the most abundant class in circulation, playing a key role in long-term immunity.
- IgA guards mucosal surfaces, protecting against pathogens in areas like the gut.
- IgM serves as the initial line of defense during an immune response.
- IgE is critical in mediating allergic reactions and responses to parasites.
- IgD remains less understood but is believed to aid in B-cell activation.
By recognizing the diversity within antibody composition, we highlight their unique functions that collectively defend the body against countless threats.
The Importance of Continued Research
As we journey deeper into the molecular intricacies of antibodies, continuously advancing our understanding is essential. This research has critical implications:
- Enhancing Therapeutics: Innovations in antibody engineering, such as monoclonal antibodies, extend our capabilities in treating diseases ranging from cancer to autoimmune disorders.
- Vaccine Development: Insights into antibody responses guide vaccine formulations, potentially leading to more effective immunizations.
- Disease Diagnostics: Antibodies are fundamental in diagnostic procedures, allowing for the detection of various diseases with precision.
The quest for knowledge doesn’t stop here. As scientists delve into the subtleties of antibody interactions and their genetic foundations, the potential for breakthroughs in medicine remains immense. With a richer understanding of these proteins, we unlock doors to novel treatments and better health outcomes for all.