Understanding Brain Structure and Function: An In-Depth Exploration
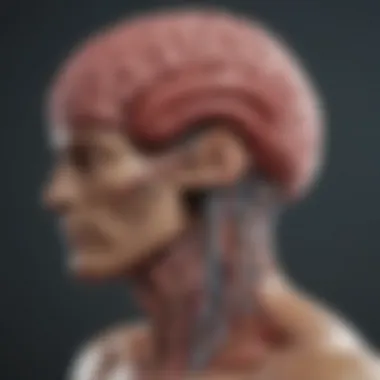
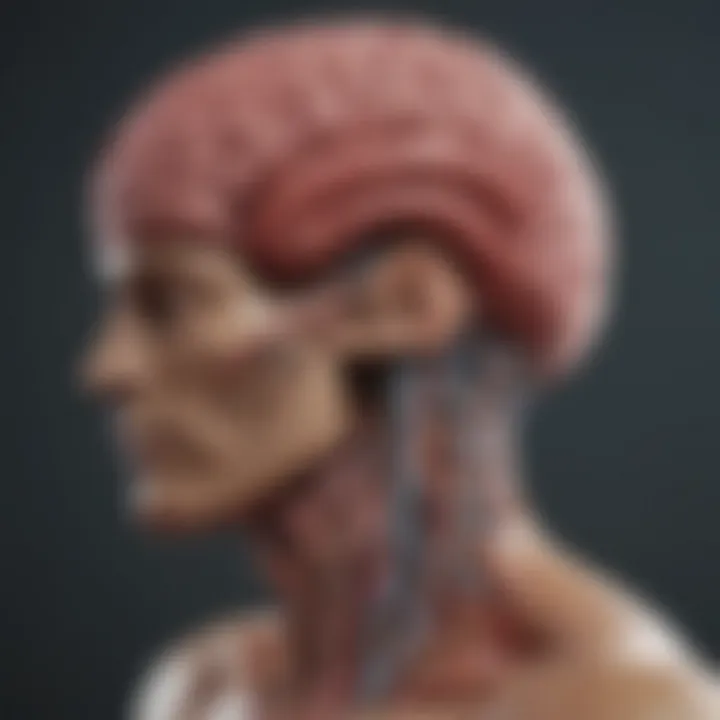
Intro
The brain is an extraordinary organ, serving as the central hub for thoughts, emotions, and behaviors. Its structure is complex and multifaceted, integrating a variety of regions and neural pathways that work collaboratively. Understanding the brain's anatomy and functions is essential in fields like psychology, neuroscience, and medical research. This article aims to unpack these intricate elements, providing a detailed exploration of how the brain operates and its critical role in human experience.
The narrative will cover significant brain regions, explore the interactions within neural networks, and delve into cognitive processes. This exploration is not just for scientists but is designed to be accessible for educators, students, and anyone interested in the workings of the brain.
Recent Advances
In recent years, the field of neuroscience has experienced remarkable growth, revealing new insights into brain structure and function. Researchers have made significant strides in understanding the intricate anatomy of the brain, uncovering how different regions play distinct roles in cognitive and behavioral expression.
Latest Discoveries
Innovative research has established critical links between specific brain regions and various cognitive functions. For instance, studies have highlighted the role of the prefrontal cortex in decision-making and social behavior. Discoveries regarding neuroplasticity have demonstrated that the brain is capable of reorganizing itself in response to new experiences or injuries, which was previously thought to be impossible after early development.
Additionally, advancements in brain imaging technologies, such as functional Magnetic Resonance Imaging (fMRI) and Positron Emission Tomography (PET), have allowed scientists to observe brain activity in real-time. These tools have opened new avenues for understanding how functional connectivity varies across different cognitive tasks.
Technological Innovations
With the rise of artificial intelligence and machine learning, researchers are now starting to utilize these technologies in neuroscience. AI can analyze complex data patterns, assisting in the identification of neural markers associated with various mental conditions. Furthermore, new methods, such as optogenetics, allow scientists to control specific neurons with light, leading to groundbreaking experiments that provide deeper insights into neural circuits.
The integration of such technologies not only furthers our understanding of brain mechanisms but can also enhance treatment options for neurological disorders.
Methodology
A comprehensive understanding of brain structure and function necessitates rigorous methodology. The following explores various approaches utilized in contemporary neuroscience research.
Research Design
Neuroscience research often employs a multidisciplinary approach, combining psychology, biology, and advanced technology. Studies might be experimental, observational, or longitudinal, dependent on the research question at hand. Experimental designs allow for controlled manipulation of variables, providing clarity in understanding cause and effect relationships.
Data Collection Techniques
Data gathered in neuroscience can come from various sources, including:
- Neuroimaging: Techniques that visualize the brain and its activity.
- Electrophysiological recordings: Measuring electrical activity of neurons.
- Behavioral assessments: Observing changes in behavior as a response to various stimuli.
These diverse techniques contribute to a comprehensive understanding of the brain's functionality, ensuring that researchers can analyze complex neural interactions.
"The brain is the organ of destiny. It holds within its humming vortex the electrical switches that set into motion the processes of our lives." - Walter Russell
In summation, the field of neuroscience is evolving rapidly. The ongoing discoveries and technological advancements pave the way for a deeper understanding of how we think, learn, and interact with the world. As we explore the anatomy and functions of the brain, it is essential to continuously engage with current research and innovations, as they inform our understanding and shape future applications in both science and medicine.
Foreword to Brain Structure and Function
Understanding brain structure and function is essential for grasping how our bodies and minds operate. The brain, as the control center of the central nervous system, plays a pivotal role in everything we do, from basic survival functions to complex cognitive abilities. This article explores various aspects of the brain, its anatomy, and how these structures interact to produce behavior, thought, and emotion. Integrating knowledge from neuroscience can enhance our appreciation of human biology and inform treatment strategies for neurological disorders.
Importance of Understanding the Brain
Knowledge of the brain's architecture and mechanisms provides insight into both health and illness. For instance, understanding neural pathways can aid in identifying where dysfunction occurs in various disorders like Alzheimer's disease or stroke. This understanding can also enhance learning and memory strategies, making it valuable not only for healthcare professionals but also for educators and students.
Furthermore, the brain's adaptability, known as neuroplasticity, has significant implications for rehabilitation following injury. Programs designed to leverage this plasticity can help individuals recover lost functions, ultimately promoting better quality of life. In short, grasping the intricacies of brain functioning can lead to more effective therapies and improved educational approaches for diverse populations.
Historical Perspective on Neuroscience
The study of the brain has a deep-rooted history, rich with initial theories and groundbreaking discoveries. From ancient civilizations that attributed mental processes to supernatural forces to modern scientists dissecting brain functions, the evolution of neuroscience reflects broader societal shifts in understanding human behavior.
In the 19th century, the advent of techniques such as the microscope allowed for detailed study of brain tissues. Figures like Santiago Ramón y Cajal established foundational knowledge about the neuron and highlighted the importance of individual brain cells in overall function.
As the field has advanced into the 21st century, innovations in neuroimaging technologies, such as fMRI and PET, have transformed our ability to study brain activity non-invasively. These modern methods continue to expand our knowledge base and offer practical applications in medicine and psychology.
Emerging fields like neuroinformatics, which combines neuroscience with technology, demonstrate the current trend towards integrating diverse disciplines for comprehensive insights into brain function. Understanding these historical underpinnings enables us to appreciate the current state of brain research and anticipate future developments.
Basic Brain Anatomy
Understanding basic brain anatomy is crucial for grasping how complex neural networks operate within the brain. The anatomical structure provides a framework for differentiating between various functions and processes that occur in the brain. Recognizing the roles of different brain regions helps in comprehending both health and illness states.
Major Brain Regions
Forebrain
The forebrain is a primary region of the brain, comprising important structures like the thalamus and hypothalamus. Its contribution to overall brain function is significant, as it is central to higher cognitive processes such as thinking, memory, and decision-making. A key characteristic of the forebrain is the cerebral cortex, which governs complex behaviors. This choice is beneficial for this article, as understanding the forebrain allows for insights into human behavior and thoughts. However, its complexity can pose challenges when trying to isolate specific functions.
Midbrain
The midbrain serves as a narrow bridge between the forebrain and hindbrain. It plays a vital role in auditory and visual processing, contributing to reflexive movements and coordination. The midbrain's key characteristic is its integration of sensory information, which is beneficial for enhancing our understanding of how the brain processes diverse sensory data. One unique feature of the midbrain is the substantial gray matter, which facilitates rapid response to stimuli. However, the intricacies of its functions may sometimes lead to misinterpretations of its role in overall brain anatomy.
Hindbrain
The hindbrain consists of the brainstem and cerebellum, crucial for survival functions such as heart rate, breathing, and motor control. A standout characteristic of the hindbrain is its role in instinctive actions and compensatory movements, showcasing its importance in maintaining basic life functions. The hindbrain is a beneficial choice for this article since it provides insights into fundamental neural mechanisms. Its unique feature lies in the cerebellum's ability to enhance movement precision, yet this might potentially overshadow the significance of the brainstem in explaining some cognitive deficits.
Cerebrum
The cerebrum, the largest brain region, is where most higher-level cognitive functions occur. Its structure includes two hemispheres responsible for various functions like reasoning and problem-solving. The cerebrum's prominent role in this article is its impact on understanding personality and behavior. A unique characteristic is that it houses specialized areas for different functions such as language and spatial awareness. However, the extensive range of its capabilities can produce challenges when attempting to pinpoint the exact origin of some cognitive abilities.
Cerebellum
The cerebellum is involved in coordination, precision, and timing of movements. It manages a range of motor tasks and contributes to balance. One key characteristic of the cerebellum is its capacity to process and regulate motor control, which is especially relevant in discussing brain function. The cerebellum's unique feature includes its right and left hemispheres that help in comparing intended movements with actual outcomes. However, its focus on movement might detract from discussions about cognitive aspects, a limitation worth mentioning.
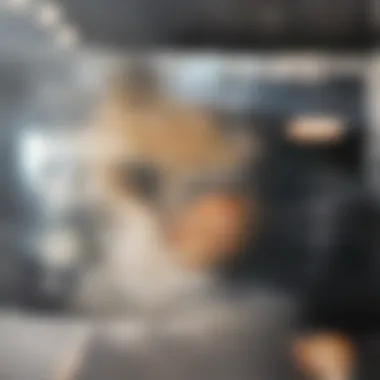
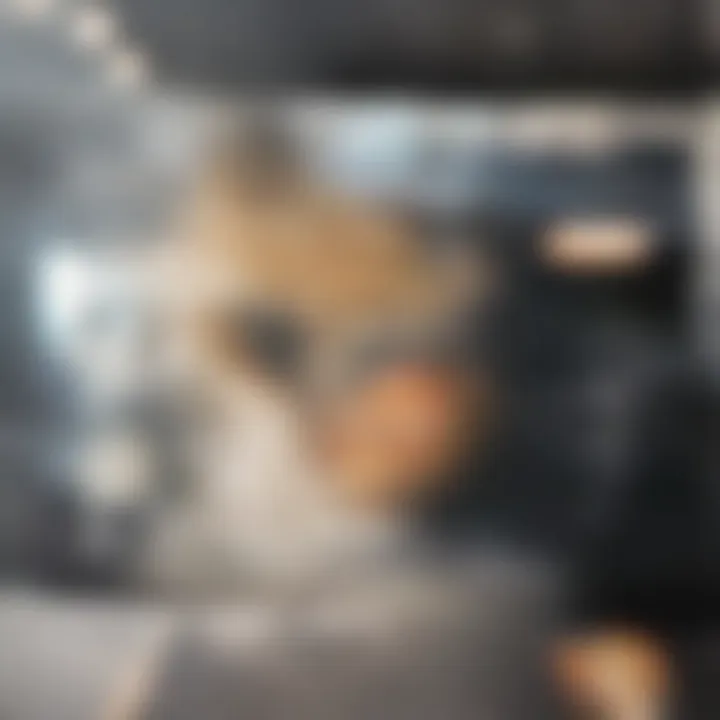
Neurons and Glial Cells
Structure of Neurons
Neurons are the fundamental units of the brain, specialized for biochemical signaling. Their structure includes a cell body, dendrites, and an axon, enabling electrical impulses to propagate. A significant characteristic of neuron structure is its ability to transmit signals rapidly, making it essential for communication within the nervous system. This merits inclusion in this article because understanding neuron structure elucidates how information is processed and stored in the brain. The unique feature of dendritic branching promotes complex signaling pathways, fostering learning and adaptation, yet it also creates vulnerability to neurological disorders.
Functions of Glial Cells
Glial cells, often overlooked, provide vital support to neurons. They are involved in maintaining homeostasis, providing insulation, and supporting neuronal health. A primary characteristic of glial cells lies in their abundance, often outnumbering neurons. This is relevant for this article, emphasizing the intricacy of brain operations. The unique feature of glial cells includes their involvement in neurotransmitter uptake and recycling, which enhances neuronal efficiency. However, their role is sometimes misunderstood or underestimated, which might lead to incomplete narratives about brain functions.
Neurotransmission Processes
Neurotransmission is the process whereby signals are passed between neurons through neurotransmitters. The efficiency of this process is essential for proper functioning of the brain. A key characteristic of neurotransmission processes is their role in modulating mood and emotions, making it a significant focus in this article. This also clarifies how various chemical imbalances can lead to disorders. The unique aspect is that neurotransmitters can have varying effects based on their receptor types, which presents both advantages in therapeutic settings and complications due to the diversity of responses. Understanding neurotransmission processes underscores the complexity of interactions within the brain, which is vital for appreciating its overall functionality.
Functional Areas of the Brain
Understanding the functional areas of the brain is essential for comprehending how various cognitive, emotional, and physical processes are managed by different brain regions. Each area plays a specific role and contributes to how we interact with and respond to our environment. The interplay of these areas is not only fascinating but also relevant to fields such as psychology, education, and health sciences.
Cerebral Cortex Functional Zones
Motor Cortex
The motor cortex is a vital component of the cerebral cortex. It is primarily responsible for the planning, control, and execution of voluntary movements. This area is divided into several regions that correspond to different parts of the body. One key characteristic of the motor cortex is its organization, wherein more delicate tasks correspond to larger areas of the cortex. This detail makes it a crucial aspect to discuss in this article, as it showcases the brain's versatility.
One unique feature of the motor cortex is its adaptability. Research indicates that it can reorganize itself in response to learning new skills or recovering from injury. This adaptability is advantageous because it highlights the brain's capacity for growth and change, which is a cornerstone of neuroplasticity.
Somatosensory Cortex
The somatosensory cortex serves to process sensory information from the body. This includes sensations such as touch, temperature, pain, and proprioception. It is located directly behind the motor cortex and is arranged similarly, which reflects the interconnected nature of sensory and motor functions. Its role in this article is to illustrate how sensory inputs shape our interactions with the environment.
A distinctive characteristic of the somatosensory cortex is its complex mapping of the body's surface. Regions that are more sensitive, such as the face and hands, occupy larger portions of this area. This aspect provides an insight into how our brain prioritizes sensory information, which is essential for understanding bodily awareness.
Visual Cortex
The visual cortex is responsible for processing visual information received from the eyes. It allows us to interpret shapes, colors, and motion, contributing significantly to our visual perception. This area is a popular topic in neuroscience as it illustrates how the brain interprets the external world.
A notable feature of the visual cortex is its layer structure, which segregates different types of visual information. For instance, certain layers are specialized for detecting motion while others focus on color. This specialization provides advantages in interpreting complex visual stimuli, thus enhancing our environmental understanding.
Auditory Cortex
The auditory cortex processes sound information, enabling us to perceive and interpret auditory stimuli. It is crucial for understanding speech, music, and environmental sounds. The auditory cortex stands out due to its role in language processing, making it significant for this article.
A unique aspect is its bilateral representation; sounds are processed in both hemispheres of the brain. This redundancy provides a protective advantage, ensuring that auditory processing remains intact even if one area is damaged.
Subcortical Structures
Limbic System
The limbic system is integral to our emotional responses and memory formation. It includes structures such as the amygdala and hippocampus. What makes this area particularly interesting is its role in connecting emotions with memory, thus affecting how we experience and recall events.
One essential aspect is its influence on behavior; emotional stimuli often enhance memory retention. This interconnectedness makes the limbic system a valuable topic in understanding the brain’s role in behavior.
Basil Ganglia
The basil ganglia play a key role in motor control, but they also impact various cognitive functions, including habit formation and reward processing. Their functions extend beyond simple movement regulation, which is crucial for understanding conditions like Parkinson's disease.
A key characteristic is their ability to facilitate smooth and coordinated movements. Their dysfunction can lead to movement disorders, highlighting their importance in both health and disease contexts.
Hypothalamus
The hypothalamus governs many autonomic functions, including hunger, thirst, and temperature regulation. This small but mighty structure plays a pivotal role in homeostasis. Its diverse responsibilities make it an essential focus for this article.
A unique feature of the hypothalamus is its role in the endocrine system; it controls hormone secretion by interacting with the pituitary gland. This regulatory function exemplifies its importance in maintaining balance within the body.
Understanding the functional areas of the brain helps to provide a clearer picture of how cognitive and physical activities are orchestrated, making it a vital topic of study in neuroscience.
Throughout this section, we have explored essential areas and how they contribute to our overall brain function. By understanding these functional zones, we can appreciate the complexity of the human brain and its remarkable ability to adapt and respond to various stimuli.
Brain Plasticity and Adaptation
Brain plasticity, often referred to as neuroplasticity, is a fundamental concept in neuroscience. It describes the brain's ability to adapt and reorganize itself throughout life. This adaptability is critical for learning, memory, and recovery from injuries. Understanding brain plasticity sheds light on how experiences, learning, and development influence our cognitive capabilities and behavioral responses. This is particularly relevant in educational settings, clinical applications, and personal development. Through understanding this topic, we can cultivate methods that promote optimal brain function and recovery following injuries.
Concept of Neuroplasticity
Neuroplasticity refers to the brain's capacity to change and rewire itself in response to various stimuli. This includes structural changes in neurons as well as modifications in functional connections between different brain areas. Neuroplasticity plays a vital role in memory formation. It also aids in skill acquisition, allowing individuals to adapt to new environments or tasks. Changes may occur at multiple levels, such as synaptic strengthening or the formation of new neural pathways. Overall, neuroplasticity underscores the brain's remarkable flexibility and highlights the importance of fostering cognitive challenge and engagement.
Factors Influencing Plasticity
Neuroplasticity is not a static trait; it is influenced by various factors as follows:
Age
Age significantly affects brain plasticity. Young brains exhibit higher plasticity compared to older adults. This characteristic makes them more adaptable to new information. For example, children learn languages more easily than adults due to their brain's heightened ability to form new connections. However, older adults can still experience plasticity, albeit at a reduced rate. Facilitating learning experiences in later life can promote cognitive function and enhance quality of life.
Experience
Experience shapes brain structure and function remarkably. Repeated practice or exposure to new skills can lead to strengthened neural connections. For example, musicians have different brain configurations compared to non-musicians due to years of practice. Experience can serve as a mechanism for brain enhancement, allowing for better cognitive outcomes and behavioral changes. Each unique experience can contribute positively or negatively to brain health, making it essential to engage in enriching activities.
Injury Recovery
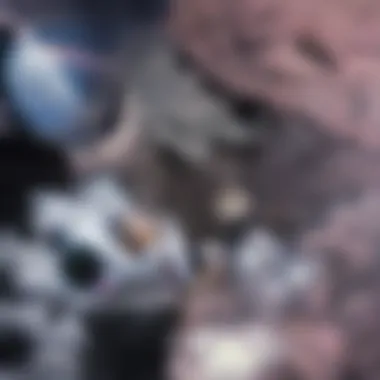
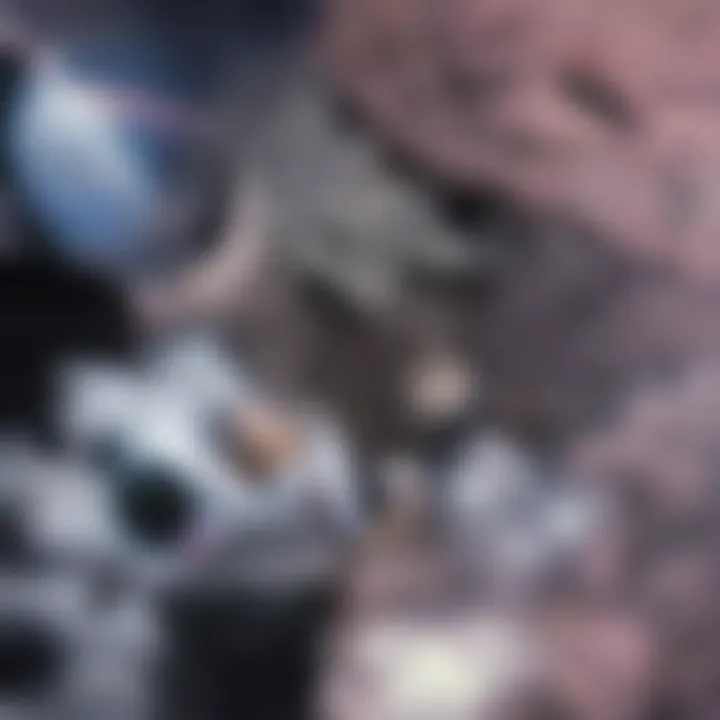
Injury recovery processes rely heavily on the brain's plastic capacity. After a brain injury, such as a stroke, neuroplasticity enables the brain to reorganize functions and compensate for lost abilities. Rehabilitation strategies often aim to stimulate plasticity through targeted therapies. However, the extent of recovery can be influenced by various factors, including the severity of the injury, the age of the individual, and the timing of interventions. Promoting an environment that supports recovery can significantly enhance outcomes for patients undergoing rehabilitation.
"The brain is much more adaptable than once believed. Knowing how to leverage this adaptability can lead to better outcomes in both health and education."
In summary, understanding brain plasticity and its influencing factors gives insight into cognitive development, learning, and recovery processes. It emphasizes the importance of continuous learning and experience, regardless of age, to maximize brain potential.
Cognitive Functions and the Brain
Understanding cognitive functions is essential to grasp how the brain operates. These functions encompass a range of processes critical for everyday life, such as memory, language processing, and emotional regulation. This section aims to elaborate on these aspects, highlighting their significance in maintaining cognitive health and enhancing educational approaches in neuroscience.
Memory Systems
Memory is foundational for learning and identity. Without it, meaningful experiences and knowledge would fade away. The brain has various memory systems that differ in duration and function. Each system plays a unique role in how we process and recall information.
Short-term Memory
Short-term memory allows individuals to hold a limited amount of information for a brief period, typically seconds to minutes. This memory type is vital for day-to-day activities, such as remembering a phone number just long enough to dial it.
One key characteristic of short-term memory is its limited capacity—often cited as the magical number seven, plus or minus two. This limitation makes it a popular point of discussion in cognitive psychology. However, its transient nature can be disadvantageous when trying to retain information effectively in learning environments.
Advantages of short-term memory include its speed and efficiency in handling immediate tasks. However, its inability to store information for longer periods can lead to quick forgetting, highlighting the need for strategies to transfer information to long-term memory.
Long-term Memory
Long-term memory refers to the system that enables the safe storage of information over extended periods, sometimes for a lifetime. This system is crucial for acquiring knowledge and skills. The strength of long-term memory lies in its capacity to store vast amounts of information.
The unique feature of long-term memory is that it can be explicit (declarative) or implicit (procedural). This flexibility allows individuals to recall facts or perform tasks without conscious thought. The challenges with long-term memory may arise from decay or interference, emphasizing the importance of regular review and practice to reinforce memories.
Long-term memory's advantages enhance learning, enabling us to build a rich tapestry of experiences and knowledge. However, the downside is that retrieval of information can sometimes be inconsistent, depending on various factors like stress and motivation.
Working Memory
Working memory involves the ability to hold and manipulate information actively. This cognitive function is often compared to a mental workspace where information can be processed and used. It is vital for tasks such as problem-solving and planning.
A key characteristic of working memory is its capacity to support complex cognitive tasks by temporarily storing relevant information. This feature makes it a beneficial choice for understanding cognition in real-time situations. However, it can also lead to cognitive overload if too much information is processed simultaneously.
Working memory contributes significantly to academic performance, especially in subjects requiring calculation or logical reasoning. The disadvantage lies in its limited duration; without continual focus, this information can easily be lost.
Language Processing
Language processing is a fundamental cognitive function that involves various brain areas dedicated to understanding and producing language. It is crucial for communication and facilitates social interaction.
Broca's Area
Broca's Area, located in the left frontal lobe, is primarily responsible for speech production and language processing. This area is key in forming grammatically correct sentences.
One major feature of Broca's Area is its role in expressive aphasia, a condition where individuals struggle to speak fluently while comprehending language. This characteristic reinforces its significance in understanding speech mechanics. The disadvantage is that damage can severely impair communication abilities.
Wernicke's Area
Wernicke's Area is situated in the temporal lobe and is vital for language comprehension. This region helps in processing and interpreting spoken and written language.
The key characteristic of Wernicke's Area is that damage here may lead to receptive aphasia, where individuals can speak fluently but have difficulty understanding language. This dysfunction highlights the complexity of language processing and the importance of both areas working together. The downside is that the affected individuals may create nonsensical sentences, complicating communication.
Language Acquisition
Language acquisition refers to the process by which individuals learn languages, often beginning in early childhood. This is crucial for cognitive development and social communication.
The unique characteristic of language acquisition is its critical period, suggesting that there is an optimal time for learning languages. This aspect makes the topic engaging in educational contexts, emphasizing how early exposure benefits language comprehension later in life. The disadvantages include difficulty in learning languages later in life due to decreased plasticity in the brain.
Emotional Regulation
Emotional regulation is the ability to manage and respond to emotional experiences effectively. This function is essential in navigating relationships and adapting to changing environments.
Amygdala
The Amygdala is a small, almond-shaped structure located within the temporal lobes. It plays a crucial role in processing emotions, particularly fear and pleasure.
One key characteristic of the Amygdala is its direct involvement in the fight or flight response. This essential feature is beneficial for survival as it prepares the body to react to perceived threats. However, overactivity can lead to heightened anxiety and stress responses.
PFC Involvement
The Prefrontal Cortex (PFC) is essential for higher-order functions, including decision making and impulse control. Its involvement in emotional regulation allows individuals to moderate emotional responses by assessing situations rationally.
A unique feature of PFC involvement is its role in long-term planning and social behavior. This characteristic marks it as a popular area of research when discussing emotional stability. However, impairments in the PFC can result in difficulty managing emotions and making sound decisions.
Impact on Behavior
The impact of emotional regulation on behavior is profound. Effective regulation leads to better social interactions and mental health. In contrast, poor emotional control is linked to various psychological disorders like anxiety and depression.
The key characteristic is the influence of emotions on decision-making processes, emphasizing the interplay between cognition and feelings. This relationship is critical for comprehending human behavior. However, the challenge arises when emotional dysregulation leads to impulsive actions that can negatively affect personal and social dynamics.
In summary, cognitive functions encompass an array of processes pivotal for our everyday capabilities. From memory systems to emotional regulation, each function is interconnected, revealing the complexity of the brain's operations.
Neurological Disorders and Brain Function
Understanding the complexities of the brain involves examining not only its structure but also how certain disorders can alter its functions. Neurological disorders can profoundly affect cognitive abilities, movement, and emotional regulation. This section will delve into various common neurological disorders, exploring their characteristics and implications on brain functionality. Recognizing these issues provides insight into the broader context of neuroscience and the challenges faced by affected individuals.
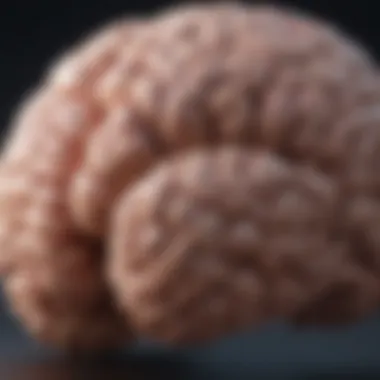
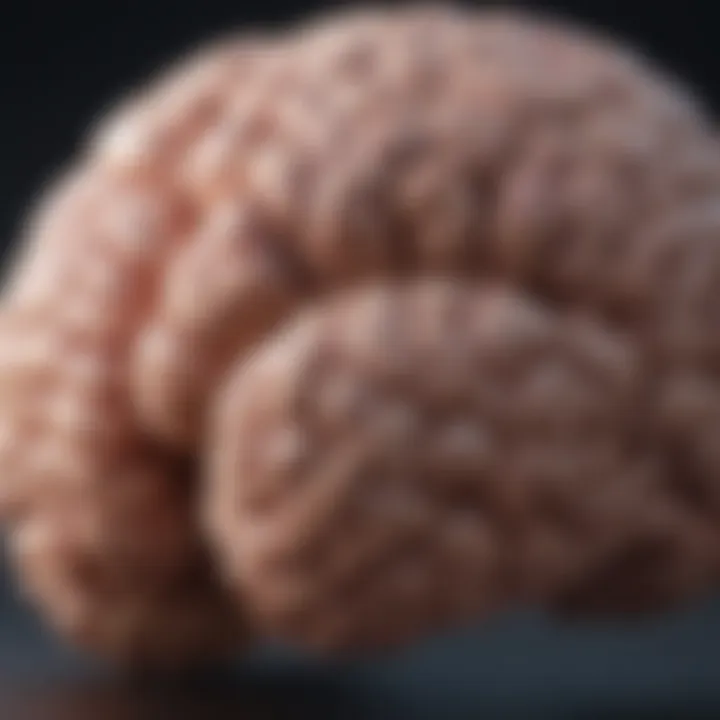
Common Neurological Disorders
Neurological disorders encompass a wide range of conditions. Among these, Alzheimer's disease, Parkinson's disease, and multiple sclerosis are prominent. Each disorder brings unique challenges and affects the brain's functionality in different manners.
Alzheimer’s Disease
Alzheimer's Disease is a progressive disorder that leads to memory loss and cognitive decline. The key characteristic of this condition is the buildup of amyloid plaques and tau tangles in the brain. This disrupts communication between neurons and leads to cell death. Its inclusion in this article is invaluable because of its growing incidence in the aging population. The unique feature of Alzheimer's is how it impacts memory, changing not only how knowledge is processed but also how individuals relate to their past experiences. Understanding Alzheimer's can shed light on the mechanisms of memory and the brain's overall functioning.
Parkinson’s Disease
Parkinson’s Disease primarily affects motor function, often causing tremors, stiffness, and balance issues. The disease is characterized by the loss of dopamine-producing neurons in the substantia nigra, a critical area of the brain that regulates movement. It serves as a significant choice for discussion in this article because of its profound implications on daily life for those affected, as well as its connection to broader neurological studies. A unique feature of Parkinson’s is its non-motor symptoms, which include changes in mood and cognition. Exploring these aspects provides a holistic view of the individual's experience with this disorder.
Multiple Sclerosis
Multiple Sclerosis is an autoimmune disorder where the immune system attacks the protective myelin sheath of nerve fibers. This disruption leads to communication problems within the nervous system. The key characteristic of multiple sclerosis is its unpredictable nature, displaying symptoms that vary widely between individuals. Including this disorder enriches the article further as it highlights how immune responses can influence brain activity and overall function. Its unique feature lies in the diversity of symptoms—from fatigue to difficulties in coordination—reminding readers of the interconnectedness of physical and cognitive functions.
Impact on Cognitive and Motor Functions
Neurological disorders can lead to significant challenges in both cognitive and motor functions. Memory loss and impaired judgment are common in conditions like Alzheimer’s, while motor control issues feature prominently in Parkinson’s. Additionally, multiple sclerosis brings about a combination of cognitive challenges and physical deficits. Understanding how these disorders affect various brain functions is crucial for developing effective treatments and supports. As these conditions progress, they present a compelling call for ongoing research and advance understanding in neuroscience.
"The brain is not simply an organ; it is a complex network of interrelated systems influenced by both structure and disorder."
Current Research Trends in Neuroscience
In the field of neuroscience, ongoing research is quintessential for expanding our understanding of the brain's structure and function. These trends primarily revolve around innovations in brain imaging technology and emerging fields of study. They represent not only advancements in scientific methodologies but also significant implications for diagnosis, treatment, and understanding neurological conditions. The trends allow researchers to uncover new insights into brain activity, cognitive functions, and the underlying mechanisms of neurological disorders, thereby enriching our overarching comprehension of the human brain.
Innovations in Brain Imaging
Advancements in brain imaging are crucial for visualizing brain activity and structure. These innovations facilitate unprecedented access to neural processes, enhancing our understanding of both healthy and diseased states. Technologies such as fMRI, PET scans, and optogenetics each contribute uniquely to psychological and medical research.
fMRI
Functional magnetic resonance imaging (fMRI) is a non-invasive imaging technique that measures brain activity by detecting changes in blood flow. This method is essential for understanding the relationship between brain function and cognition. A key characteristic of fMRI is its ability to provide real-time images of brain activity, making it a valuable tool for research in various neuroscience fields.
One unique feature of fMRI is its capacity to capture dynamic changes in brain activity associated with different tasks. Its strengths include high spatial resolution and non-invasive nature, allowing for repeated measurements in the same subject. However, the temporal resolution is limited compared to other techniques, which can present challenges when studying rapidly changing processes in the brain.
PET Scans
Positron emission tomography (PET) scans are another key imaging method used to observe metabolic processes in the brain. The primary function of PET scans is to visualize how the brain uses glucose, linking metabolic activity to cognitive function. This characteristic provides valuable insights into disorders like Alzheimer’s disease, where metabolic activity is altered.
A significant feature of PET scans is their capacity to measure neurotransmitter activity and receptor density. This capability is beneficial for understanding drug effects on the brain. However, PET scans require the use of radioactive tracers, which raises concerns about radiation exposure over repeated scans.
Optogenetics
Optogenetics is a revolutionary technique involving the use of light to control neurons that have been genetically modified to express light-sensitive ion channels. This method allows for precise control of specific neuronal populations, providing insights into how neural circuits operate during behavior.
A distinctive attribute of optogenetics is its capacity to achieve high temporal precision, enabling researchers to study the effects of neuron activation or inhibition in real-time. While this technique is groundbreaking, its application is still primarily in animal models, and translating findings to human studies remains a significant challenge.
Emerging Fields of Study
As neuroscience progresses, new fields are developing, enriching our understanding and offering novel approaches to study the brain. Neuroinformatics, artificial intelligence in neuroscience, and robotics and brain interfaces are highlights of these emerging areas.
Neuroinformatics
Neuroinformatics combines data science with neuroscience, utilizing computational tools to analyze large datasets from brain studies. This field enhances collaboration and data sharing among researchers, promoting a more cohesive understanding of brain function. A key advantage of neuroinformatics is its ability to synthesize complex information from diverse studies, leading to insights that single research efforts may not uncover.
However, the complexity of data integration poses challenges, as it requires sophisticated computational tools and methodologies.
Artificial Intelligence in Neuroscience
Artificial intelligence (AI) is increasingly utilized in the analysis of neurological data. Machine learning algorithms can identify patterns in brain activity, paving the way for advancements in diagnosing conditions and predicting treatment responses. A primary strength of AI in neuroscience is its ability to process vast amounts of data far quicker than human analysis, uncovering hidden correlations.
Nonetheless, ethical considerations surrounding data privacy and the interpretability of AI-driven conclusions remain ongoing discussions within the field.
Robotics and Brain Interfaces
Robotics and brain interfaces focus on creating connections between robotic systems and the human brain. This field has significant implications for rehabilitation following neurological injuries or conditions. A notable characteristic of these technologies is the potential for restoring lost motor functions or enhancing communication in patients with severe disabilities.
The unique feature of these systems is their capacity to translate neural activity into robotic actions, allowing for real-time interaction between human thoughts and machines. Challenges include ensuring reliable interfacing and managing the ethical implications of such technologies in human healthcare.
Neuroscience is evolving rapidly, driven by technological advancements and innovative approaches, presenting ongoing opportunities for discovery and application.
Research trends in neuroscience are not just academic; they have real-world implications that can transform lives. Understanding innovations like fMRI, PET scans, and optogenetics can aid in tackling significant neurological issues today.
Culmination and Future Directions
The conclusion draws together the various aspects of brain structure and function explored throughout this article. It is crucial to acknowledge that our understanding of the brain continues to evolve. The complexities of neural networks, plasticity, and cognitive processes reveal just how intricate the human brain is. With each study, researchers gain new insights that can lead to innovative approaches in education, healthcare, and neuroscience.
The importance of synthesizing knowledge in this area cannot be overstated. A comprehensive grasp of brain anatomy assists in grasping its functionality. Furthermore, acknowledging the interplay between different brain regions can illuminate various cognitive and emotional outcomes. Recognizing the trends in neuroscience not only enriches our comprehension but opens new avenues for intervention and treatment of neurological disorders.
Summarizing Key Insights
Throughout the article, readers have explored several key insights:
- Brain Structure: Various parts of the brain, including the cerebral cortex, limbic system, and subcortical structures, play critical roles in behavior and thought processes.
- Neuroplasticity: The brain's ability to change is fundamental to recovery from injury and adaption to new experiences. Factors such as age and learning experiences can significantly influence this adaptability.
- Cognitive Functions: Memory, language, and emotion are all closely tied to specific brain regions. Understanding these can enhance approaches to teaching and therapy.
Overall, these insights contribute to a better understanding of how complex interactions within the brain result in a rich tapestry of thoughts, behaviors, and emotions.
Prospects for Further Research
As we look to the future, several promising research areas emerge in neuroscience. Some of these include:
- Advanced Imaging Techniques: Innovations such as fMRI and PET scans will allow for more detailed observation of brain activities and structures. These methods can lead to refined diagnostic tools for neurological disorders.
- AI and Neuroscience: The integration of artificial intelligence in studying neural data presents exciting opportunities. This collaboration could revolutionize our understanding of brain function and significantly enhance treatment methods.
- Interdisciplinary Studies: There is an emerging focus on how neuroscience intersects with psychology, education, and biology. These interdisciplinary approaches may yield new insights into human behavior and learning processes.
By pursuing these areas, researchers can contribute to the continuously expanding field of neuroscience. Each discovery may not only enhance medical science but also inform practical applications in everyday life.