Understanding EDTA's Role in Cell Culture Practices
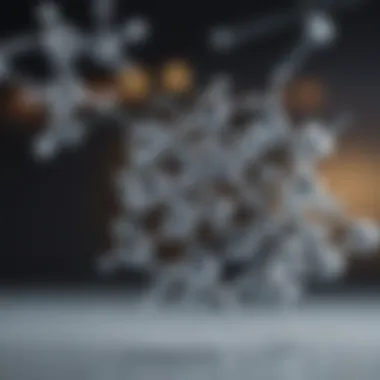
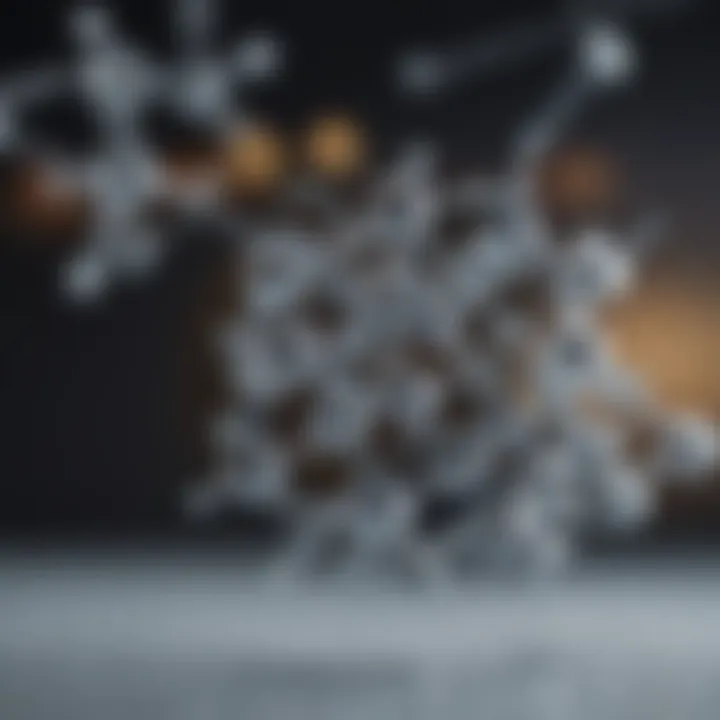
Intro
Ethylenediaminetetraacetic acid, known as EDTA, plays a surprisingly pivotal role in the realm of cell culture. While often overlooked, this chelating agent offers a range of benefits that can significantly enhance experimental outcomes. Researchers and students alike will find that understanding the properties and functions of EDTA in cell culture is essential for successful experimentation and bioprocessing. This article aims to shed light on EDTA's multifaceted applications, highlighting key aspects of its use, practical considerations for effective incorporation, and its overarching impact on cellular studies.
EDTA's primary function in biological applications hinges on its ability to bind metal ions. This attribute complicates the movement of these ions in a cell culture environment, potentially creating an atmosphere conducive to cell growth and viability. As we dive deeper into the nuances of EDTA, we will explore its recent advances, methodologies for its application, and the underlying mechanisms driving its effectiveness. The ultimate goal is to arm both seasoned professionals and budding researchers with knowledge that underscores the significance of EDTA in cell culture practices.
Prologue to EDTA in Cell Culture
The significance of Ethylenediaminetetraacetic acid (EDTA) within the realm of cell culture cannot be overstated. It serves as a cornerstone compound in various biological and biochemical applications. For students and seasoned researchers alike, understanding EDTA's role is fundamental. Not only does it help maintain the integrity of cell cultures, but it also greatly influences experiments in ways that can be both subtle and profound.
Definition and Chemical Structure
EDTA is a chelating agent, which means it has the unique ability to bind metal ions. Chemically, it is recognized as a colorless crystalline solid that is soluble in water. The chemical structure includes four carboxylic acid groups and two amine groups, making it adept at forming complexes with a variety of metal ions such as calcium, magnesium, and iron. This binding action is essential because these metal ions can otherwise precipitate and interfere with cell viability and function. Understanding its formulation is crucial as researchers often manipulate these properties to establish optimal conditions for cell growth and experimentation.
Historical Context
The journey of EDTA started in the early 20th century. Initially synthesized in 1935 by Frederick G. Claiborne, it was primarily studied for its properties in complexing metals in industrial contexts. However, its potential for biological applications came to light when researchers discovered its ability to effectively sequester divalent cations, which are essential in many enzymatic reactions. Over the decades, it has been adopted widely within laboratories, becoming a staple in not just cell culture but also in areas like blood collection and preservation. This historical thread weaves a rich tapestry of EDTA's evolution and emphasizes its significance as a versatile tool in the life sciences.
"EDTA's journey from industrial applications to a central figure in cell culture epitomizes its extraordinary versatility within scientific research."
In summary, EDTA's influence permeates various aspects of cell culture practices. With its chelating properties and rich history, it embodies a blend of chemistry and biological significance that continues to be invaluable in contemporary research.
Chemical Properties of EDTA
Understanding the chemical properties of Ethylenediaminetetraacetic acid (EDTA) is instrumental in appreciating its role in cell culture. EDTA is renowned primarily for its chelating abilities, allowing it to bind metal ions effectively. These properties not only play a pivotal role in maintaining the health of cell cultures but also contribute significantly to experimental outcomes. Proper grasp of these characteristics informs usage, enhances results, and can mitigate unintended consequences in research involving cellular systems.
Chelating Mechanism
Metal Ion Binding
Metal ion binding is one of the hallmark features of EDTA’s chelating mechanism. This property allows it to sequester divalent and trivalent metal ions, which can be detrimental to cell growth if present in excess.
When EDTA binds to these ions, it forms stable complexes that help reduce their bioavailability. This is particularly crucial in cell culture, where metals such as calcium or magnesium can interfere with cellular activities if not carefully regulated. The ability of EDTA to effectively 'pull' ions out of solution provides a buffering effect that maintains optimal growth conditions for cells.
Moreover, the unique ability of EDTA to form multiple coordination bonds enhances its stability in biological systems, making it a widely favored choice among researchers. The advantage lies in its capacity to prevent toxicity, which is essential for maintaining cell viability. However, one must reckon with its potential downsides, such as the possibility of inhibiting enzymatic activities that depend on these metal ions. Therefore, understanding its use in context is invaluable.
"The binding action of EDTA is analogous to how a skilled negotiator ensures that the most volatile elements stay at bay, fostering a productive environment for growth."
Stability Constants
Stability constants provide a quantitative measurement of how tightly a chelate, such as EDTA, binds to a metal ion. This information is not only relevant for chemical interactions but also crucial for practical applications in cell culture. A higher stability constant indicates that a chelate forms a more stable complex with a metal ion, suggesting that the metal is less likely to dissociate and re-enter the bioavailable pool. This can effectively minimize toxic levels in culture media.
The unique feature of EDTA's stability relates to its structure, allowing it to stabilize various metal ions across different pH conditions. What sets it apart is that, compared to many other chelating agents, EDTA tends to have a broader range of stability constants. This characteristic enables its versatile use across various biological scenarios, making it a go-to for many researchers.
Nonetheless, as with metal ion binding, stability constants can present challenges. Depending on the cell type or experiment, an over-stabilized complex might hinder the natural bioavailability of essential ions, once more necessitating a careful approach in experimental conditions.
pH and Ionic Strength Effects
The effectiveness of EDTA within cell cultures can be heavily influenced by the pH and ionic strength of the solution. These factors determine how EDTA interacts with metal ions in the solution, subsequently influencing cell growth and survival.
At different pH levels, the effectiveness of EDTA can vary; for instance, it can become more effective at chelating certain metals in a more basic environment, while in an acidic milieu, its efficiency might wane. Therefore, the tuning of pH is often tailored in protocols to optimize the chelation process for specific cell types.
Moreover, the ionic strength of a solution—remarkably influenced by the presence of other salts—can impact EDTA's activity. Increased ionic strength can lead to competition for binding sites, which may result in reduced efficacy. Thus, maintaining appropriate ionic strength helps to ensure that EDTA performs its role effectively, providing researchers the stability they need in their experimental frameworks.
In summary, the chemical properties of EDTA, from its chelating efficiency to how it reacts under varying conditions, create a nuanced balance that researchers must navigate to ensure successful cell culture practices. Understanding these intricacies can be the difference between a thriving culture and one that faces detrimental setbacks.
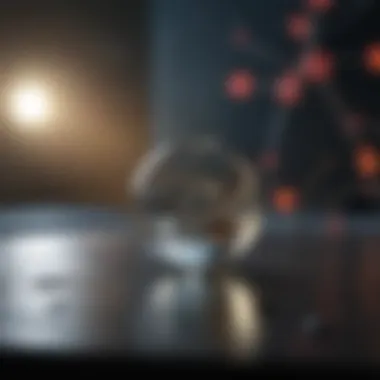
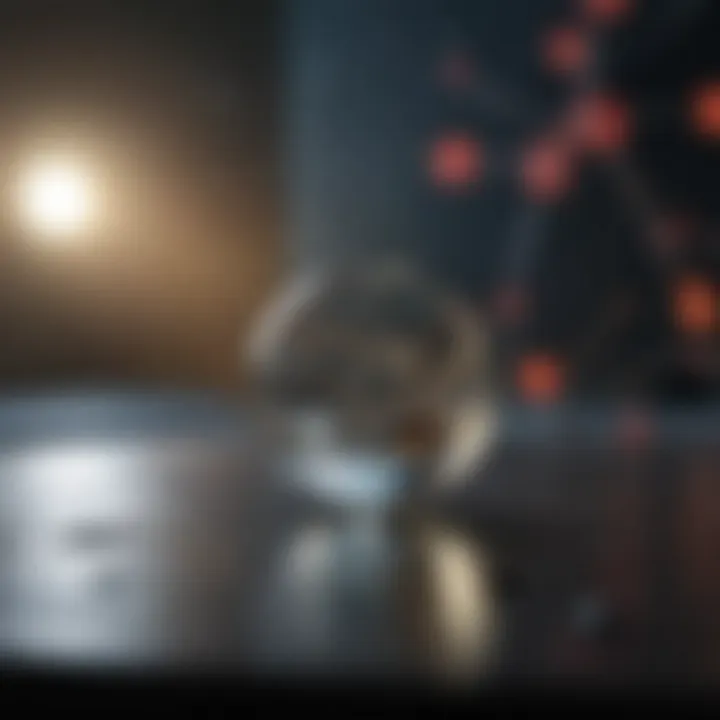
Applications of EDTA in Cell Culture
The applications of EDTA in cell culture stand as a cornerstone in modern biological sciences. This versatile chelating agent not only plays a critical role in the preservation and viability of cells but also profoundly influences cell growth and apoptosis studies. Its significance is widely acknowledged across various research domains, making an understanding of these applications necessary for both novices and seasoned professionals in the field.
Cell Preservation and Viability
One of the primary applications of EDTA is in the preservation of cells during culture processes. By chelating divalent metal ions, EDTA helps prevent the harmful effects that free metal ions can have on cell health. These ions, often present in growth media, can lead to oxidative stress and subsequent cell damage.
Using EDTA effectively promotes cell viability by creating a more stable and protective environment. In this context, researchers have become aware of how EDTA can enhance the storage of cell lines. For instance, when primary cells are cryopreserved, the right formulation containing EDTA can greatly influence survival rates post-thaw. This attribute makes brainstorming the right EDTA concentration crucial for protocols, especially for sensitive cell types.
"A robust cell culture environment is not just a lab bench activity; it’s an art that blends science with nuanced understanding."
Enhancing Cell Growth
Enhancing cell growth is another pivotal application of EDTA in cell culture. The effects of EDTA on cell proliferation and metabolic activity largely hinge on its chelating capabilities. This agent facilitates the availability of essential nutrients while simultaneously restricting the availability of potentially toxic metal ions.
Synergistic Effects with Other Compounds
The synergistic effects of EDTA when paired with other compounds deserve attention. For example, when mixed with various amino acids or vitamins, EDTA can amplify their efficacy, resulting in superior cell growth. What stands out is how certain combinations can bolster nutrient uptake by cells, leading to a more substantial proliferation rate.
Such mixtures can optimize medium formulations not commonly seen in traditional setup; think about integrating EDTA with L-glutamine or ascorbic acid. This becomes a point of significance for researchers aiming to elevate their culture techniques. However, care must be taken since not all combinations yield positive results; empirical testing is essential to find the sweet spot between enhancing growth and causing inhibitory outcomes.
Growth Factors and Serum Free Medium
Another relevant aspect is the role of growth factors in serum-free mediums alongside EDTA. Serum, often deemed essential for cell survival, poses challenges such as variability and ethical concerns. Using growth factors in serum-free mediums, combined with EDTA, opens doors to more controlled and defined environments.
The flexibility of this approach is quite appealing, as different cell types require specific growth conditions. Researchers can tailor the medium, incorporating essential growth factors while benefiting from EDTA's protective qualities. This synergy can enhance cell behaviors, thereby contributing to the overall viability and health of the cultured cells. Nevertheless, acquiring and standardizing these growth factors can sometimes be resource-intensive.
Apoptosis and Cell Cycle Studies
EDTA's involvement doesn’t stop at promoting well-being in cell cultures; its utility transcends into the realms of apoptosis and cell cycle studies. By modulating ionic conditions, EDTA has been shown to influence apoptotic pathways, presenting an interesting avenue for analyzing cell fate more precisely. Researchers are exploring how the chelating process might induce apoptosis under certain conditions, providing a dual perspective on its role in cell culture practices. This creates a deeper understanding of cellular responses and opens new pathways for therapeutic explorations.
EDTA and Cellular Response
The interaction of Ethylenediaminetetraacetic acid, commonly known as EDTA, with cells is a crucial aspect of its role in cell culture. Understanding how EDTA influences various cell types can provide invaluable insights, especially for researchers keen on maximizing experimental outcomes. EDTA’s capacity to chelate metal ions exerts significant effects on cellular responses, from proliferation to apoptosis. This section will focus on how cell type differences affect these responses, illuminating the importance of adapting methodologies to cater to distinct cellular requirements.
Influence on Cell Types
Adherent vs. Suspension Cells
Adherent cells, as the name suggests, are known for their tendency to anchor themselves to a substrate. These cells generally require a solid support system for optimal growth. In contrast, suspension cells thrive in a liquid medium, floating freely. The resilience of adherent cells can be a significant asset, particularly in experiments aiming at cell differentiation or responding to chemical stimuli like EDTA. Their attachment to the surface tends to enable better nutrient absorption and waste product removal, providing a stable environment for growth.
One key advantage of using adherent cells in conjunction with EDTA is their responsiveness to treatment; they typically exhibit a more pronounced morphological change following exposure. However, this comes with a trade-off. These cells may develop a dependency on their substrate, potentially skewing results if the attachment is disrupted.
On the other hand, suspension cells enjoy the freedom of movement, which can facilitate studies on cell signaling and interactions with soluble factors. For example, the opening up of a new avenue for research could be noted here as these cells can provide a more agile response to chelating agents. Nevertheless, their growth can be less predictable, often requiring more nuanced handling. Thus, choosing between adherent and suspension cells can shape the approach you take when incorporating EDTA into your experiments.
Primary Cells vs. Cell Lines
The différentiation between primary cells and established cell lines serves as another layer in the exploration of EDTA's role in cell culture. Primary cells are directly harvested from living tissues, providing a wide representation of the cellular features and behavior found in their natural environment. They often mirror in vivo biology closely but come with a limited lifespan and varying responsiveness to EDTA exposure, which can complicate experiments.
Conversely, cell lines are cultivated from primary cells, often immortalized to permit extended growth. Their consistency can be appealing for many researchers. However, this can result in gene expression changes that may not fully represent their primary counterparts, leading to results that might be misleading. In relation to EDTA, established cell lines may exhibit a more pronounced adjustment in viability and growth, often demonstrating more resistance due to pre-adaptations in vitro. Their uniformity may simplify assays but could potentially mask the nuanced effects of EDTA seen in more variable primary cell populations.
The selection between these two types hinges on the specific research goals and desired outcomes.
Mechanistic Insights into Cell Behavior
Delving deeper into the mechanistic insights into how EDTA affects cell behavior unveils a complex web of biochemical interactions. EDTA impacts cellular signaling pathways, often linked with calcium dynamics, and can mitigate the effects of metal ion toxicity. Such chelation is vital in environments where heavy metals may introduce unwanted variables.
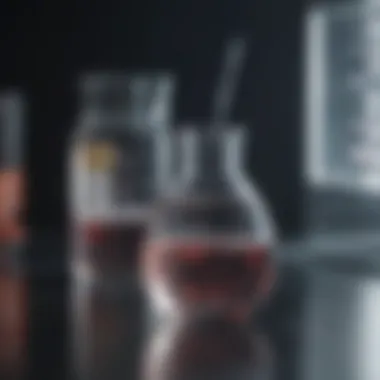
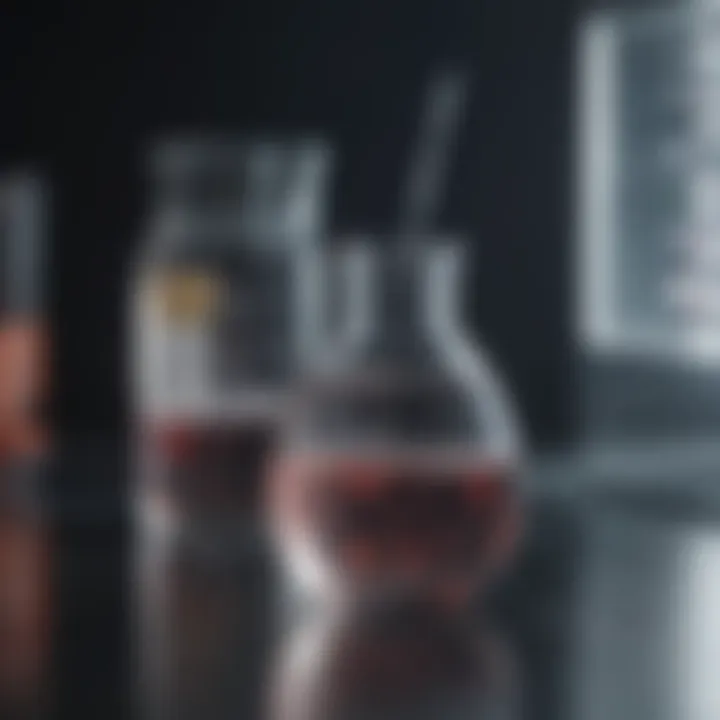
Furthermore, EDTA’s influence extends to mediating apoptosis and influencing proliferation rates, highlighting its multifaceted nature. Understanding these details is crucial for optimizing cell culture practices. Researchers can tailor their outlines based on how individual cell types respond differently to the presence of EDTA, facilitating better experimental designs.
"Choosing the right cell type and chelating agent can very well make or break an experiment."
This summary drives home the necessity of adapting experimental setups to align with the cellular responses elicited by EDTA, ensuring that research findings are both robust and meaningful.
In wrapping up this section on cellular response, it is apparent that the choice of cell type interacts intricately with the way EDTA functions. Armed with this knowledge, researchers can navigate the complexities of cell culture with much greater efficacy.
Practical Considerations in Using EDTA
When navigating the complexities of cell culture, the incorporation of EDTA requires thoughtful deliberation. Understanding the specific practical considerations surrounding its use not only enhances experimental outcomes but also minimizes the risk of undesired effects. In this section, we will dive into optimal concentrations for different applications, timing of exposure, and potential cytotoxicity associated with EDTA. Each of these elements contributes significantly to the role EDTA plays in cell culture and how researchers can wield it for maximal benefit.
Optimal Concentrations for Different Applications
Getting the concentration of EDTA just right can feel like trying to balance on a tightrope—too little, and it might not perform its chelating function effectively; too much, and you could end up compromising cell health. The ideal concentration varies widely depending on the cell type and the specific application at hand.
Typically, a common range used in cell culture is between 0.1 mM to 5 mM. For instance, in the context of trypsinization, a lower concentration of around 0.5 mM may be sufficient to detach adherent cells without causing undue harm. However, when it comes to certain storage solutions or protocols requiring the mitigation of metal ion-induced toxicity, concentrations could go much higher, often up to the 5 mM mark.
A careful dose-response analysis presents a great case study here, illustrating the critical dependence on empirical data to guide the effective use of EDTA.
Time of Exposure
The duration for which cells are exposed to EDTA can have a profound influence on their viability. Prolonged exposure, while sometimes necessary for effective chelation, often results in unintended cytotoxic effects. Understanding the balance here is pivotal for researchers.
For general practice, a time frame of 5 to 15 minutes often suffices in detaching cells or facilitating desirable chemical reactions without leading to cellular compromise. Longer periods may be needed in specialized cases, such as during the treatment of metal toxicity, but these should not be the norm. Scientists should have a keen eye on the cellular response, as each cell type may react differently; thus a one-size-fits-all approach doesn't work here.
Potential Cytotoxicity
Concentration-Dependent Effects
The relationship between the concentration of EDTA and its cytotoxic effects can be described as a double-edged sword. At appropriate concentrations, EDTA provides much-needed protection and supports cell growth; however, as the concentration escalates, this same compound can initiate detrimental effects—quite a paradox.
A key characteristic of concentration-dependent effects is the nonlinear behavior observed, wherein slight adjustments in dosage result in marked changes in cell viability. Research often indicates that while concentrations around 1 mM seem safe for many cell lines, pushing it to 10 mM may start to cause significant cellular distress, including signs of apoptosis. Thus, monitoring and careful adjustment is crucial.
Cell Type Sensitivity
When it comes to cell type sensitivity, not all cell lines are created equal. This notion is crucial; some cell types tolerate EDTA better than others. For example, various adherent cell lines may show resistance to higher concentrations due to robust cell membranes, while many primary cells might be more susceptible to the cytotoxic effects.
The unique feature of cell type sensitivity is essential for tailoring experimental procedures. Understanding which cell lines thrive under specific conditions allowed by EDTA can streamline protocols and minimize waste. It is wise to pilot studies with varied cell types to discern their responses, never underestimating the diversity inherent in cell behavior.
Achieving a delicate equilibrium between effective metal chelation and maintaining cell health speaks to the broader narrative of optimizing cell culture protocols—an endeavor requiring both skill and diligence.
Effective utilization of EDTA in cell culture is akin to fine-tuning an intricate instrument. One must pay close attention to the delicate balance of concentration and exposure time to avoid falling into the traps of cytotoxicity.
Alternatives to EDTA in Cell Culture
In the world of cell culture, Ethylenediaminetetraacetic acid—commonly known as EDTA—has solidified its role as a prominent chelating agent. However, while EDTA boasts a host of advantages, it’s also crucial to consider alternatives. Various scenarios might call for substituting EDTA or employing different methodologies that could yield improved outcomes or mitigate specific drawbacks. This section aims to explore viable alternatives to EDTA, emphasizing not only their chemical properties but also practical benefits and considerations in cell culture applications.
Other Chelating Agents
Among the alternatives to EDTA, several other chelating agents can serve similar functions in cell culture. These alternatives often come with unique mechanisms of action and specific applications:
- Citric Acid: Known for its mild chelating properties, citric acid is effective in binding metal ions. Its use can enhance the bioavailability of nutrients within culture media, promoting cell growth without the potential cytotoxic effects sometimes associated with EDTA.
- NTA (Nitrilotriacetic Acid): NTA presents a different binding profile compared to EDTA; its affinity for certain metal ions can be higher, making it a favored choice in specific laboratory settings. Moreover, NTA's application is often aligned with engineering bioprocesses, enhancing the efficiency of metal ion removal.
- DTPA (Diethylenetriaminepentaacetic Acid): Like EDTA, DTPA binds metal ions but offers greater stability over a wider range of pH levels. Neutralizing specific trace elements can be beneficial, especially in studies demanding high reproducibility.
- Malic Acid: This organic acid can chelate certain metal ions and is less toxic to cells compared to more robust chelators. It can also assist in maintaining cellular metabolism and energy production.
These chelating alternatives typically exhibit lower toxicity profiles and could theoretically alleviate some of the concerns associated with extended EDTA exposure. As your exact application evolves, weighing the advantages and potential downsides of such substitutes will help ensure optimal conditions for cultured cells.
Non-Chelating Alternatives
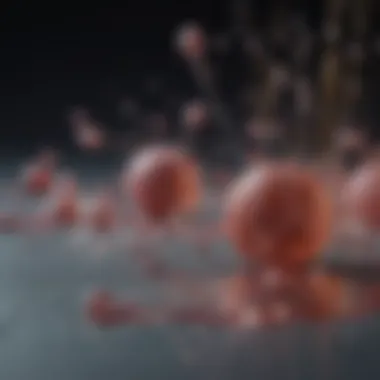
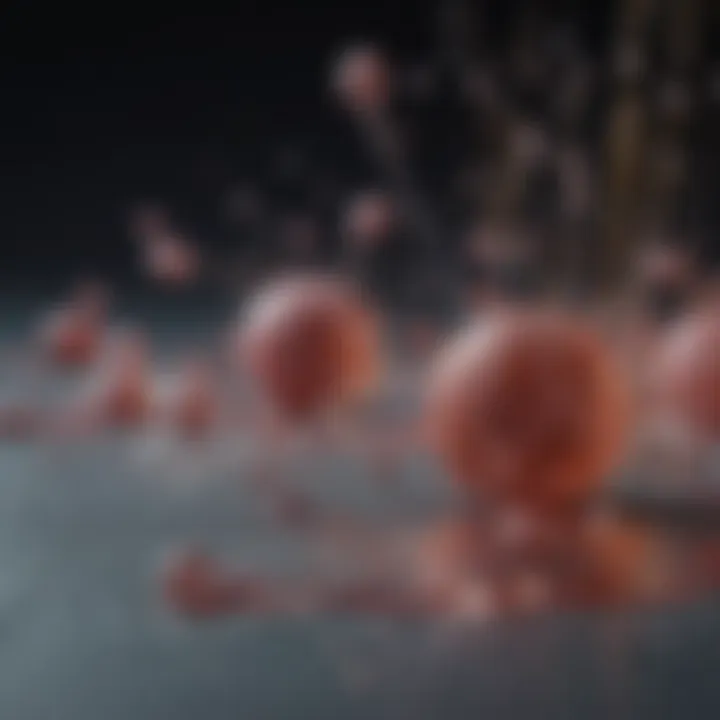
Some scenarios may not warrant any chelating agents at all. Non-chelating alternatives can be employed effectively in cell culture, providing either similar or even improved outcomes:
- Buffering Agents: Substitutes like HEPES or MOPS can control pH without potentially adverse interactions with metal ions found in culture media. These agents facilitate maintaining a steady environment essential for cellular processes without the metal-binding effect of chelators.
- Antimicrobial Agents: Introducing non-chelating antimicrobial options can help ward off contamination issues without the drawbacks of a chelating agent. For instance, using penicillin-streptomycin combinations may effectively reduce microbial threats without impacting metal ion levels.
- Nutrient Supplements: Instead of relying on chelators, the introduction of supplements such as amino acids or vitamins may enhance cell viability and growth. This approach focuses on addressing the nutritional needs of the cells, circumventing potential drawbacks from chelation.
In summary, while EDTA holds a reputable position in cell culture practices, assessing alternatives—both chelating and non-chelating—opens doors to innovative methodologies in research. The choice among these alternatives should be dictated by specific experimental design, cell type requirements, and desired outcomes.
Future Directions in EDTA Research
As we march forward into a more complex landscape of cell culture methodologies, the examination of EDTA continues to be of significant importance. Understanding where this exploration could lead is paramount for researchers, educators, and professionals alike. Right off the bat, the relevance of this topic cannot be overstated—one might say EDTA is a kind of unsung hero in the lab, quietly making a huge impact in cellular experiments. What comes next for EDTA can open doors to a realm of possibilities and innovations.
Technological Advances in Cell Culture
Technology is marking its footprint everywhere, and cell culture is no exception. Our understanding and application of EDTA is also undergoing a transformation thanks to advancements in technology. Techniques like high-throughput screening and genomic editing are at the forefront. These modern strategies necessitate a deeper comprehension of how EDTA interacts with cells at a molecular level. With the leap in imaging technologies, researchers can now visualize cellular responses in real-time, observing firsthand how EDTA influences physiological behaviors.
- Automation: Enhanced automation in laboratories optimizes the application of EDTA, allowing for standardized and precise dosing, improving consistency in results.
- Microfluidics: This cutting-edge technology makes it possible to study cellular responses to EDTA under a variety of conditions with minimal sample sizes.
By leveraging these advancements, researchers can refine experimental designs, leading to new methodologies that capitalize on EDTA's properties, ultimately augmenting its utility in research.
Environmental Considerations
As research turns increasingly toward sustainability, the environmental footprint of chemical agents like EDTA is under scrutiny. Traditionally, EDTA has been viewed as a benign chelator, but its persistence in the environment raises questions. It’s essential to scrutinize how its widespread use might affect ecological systems.
Understanding the breakdown products of EDTA and their potential toxicity is vital. Considerations include:
- Biodegradability: Researching how EDTA can be modified or supplemented with alternatives that reduce its environmental impact.
- Recycling and Reuse: Pushing for systems that could allow for the recycling of EDTA solutions in lab practices.
In essence, future research could pivot toward creating greener alternatives while maintaining the effectiveness of cellular experiments.
Regulatory Implications
As the scientific community unravels more discoveries concerning EDTA, regulatory bodies will inevitably respond. This leads us into a multifaceted discussion about compliance and usage regulations.
- Safety Data: The collection of comprehensive safety and toxicity data will likely become paramount for enforcing better regulations.
- Ethical Guidelines: The exploration of new applications may lead to the formulation of new ethical guidelines related to biochemistry and pharmacology.
Regulatory implications often affect research dynamics. As scientists navigate these waters, they must amalgamate solid data with proper ethical considerations. EDTA's multifaceted role in cellular studies necessitates that researchers stay updated with the evolving regulatory landscape not just locally, but globally.
The future of EDTA research holds both promise and responsibility; the need to innovate and comply only grows with time.
In closing, the future directions in EDTA research indicate an intersection of technological progress, environmental consciousness, and regulatory scrutiny that could redefine its role in cell culture. Every bit of advancement will contribute to the broader understanding of what makes our cellular studies more effective while also being mindful of our environmental responsibilities.
Closure
In examining the various facets of Ethylenediaminetetraacetic acid, or EDTA, it becomes evident that its role in cell culture is not merely supplementary but rather fundamental. This section distills the essence of what has been discussed and highlights the pertinent aspects of EDTA’s application in scientific endeavors.
EDTA acts as a chelating agent, effectively binding metal ions that might otherwise interfere with biological processes within cultured cells. By maintaining specific environments essential for cell growth and stability, this compound not only enhances cell viability but also contributes significantly to experimental reproducibility. Moreover, the article has illustrated the importance of understanding concentration levels and time of exposure when utilizing EDTA, as these factors can affect the cytotoxic impact on various cell types.
Summary of Key Points
The discussion on EDTA has encompassed crucial points, including:
- Chemical Properties: Its unique capability to form complexes with metal ions, thus preventing detrimental effects in the cell culture medium.
- Applications: Importance in cell preservation and enhancing growth, proving particularly beneficial in various experimental contexts.
- Cellular Response: Distinct impacts on different cell types, emphasizing the need to adapt usage based on the specific characteristics of the cells in question.
- Practical Considerations: Detailed insights into optimal usage protocols, dosage, and potential cytotoxicity concerns, which are essential for successful application in laboratories.
These elements collectively underscore EDTA’s pivotal role in fostering a productive environment conducive for cell culture and research.
Implications for Scientific Research
The ramifications of EDTA's role in cell culture extend far beyond the laboratory. Scientific research thrives on consistency and reliability, and EDTA significantly enhances these qualities. Its ability to stabilize environments where cells thrive allows researchers to draw more accurate conclusions and facilitates the replication of experiments, a cornerstone of scientific integrity.
In addition, as technological advancements usher in more intricate cellular studies, understanding and manipulating cellular behavior with agents like EDTA can lead to breakthroughs in fields from regenerative medicine to toxicology.
Selecting appropriate alternatives when necessary remains a considerate choice as non-chelating agents may also hold valuable properties beneficial for particular experimental structures.
Ultimately, this article has aimed to weave a narrative that not only informs but also enlightens readers about the integral nature of EDTA in cellular studies, emphasizing its multifaceted contributions across a range of disciplines.