Understanding Innovations in Electron Microscopy
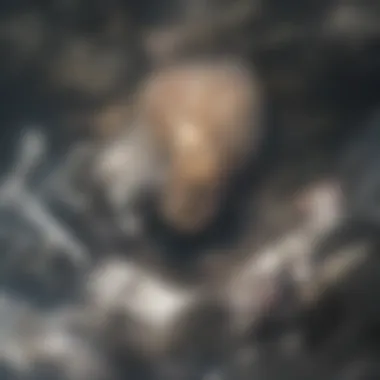
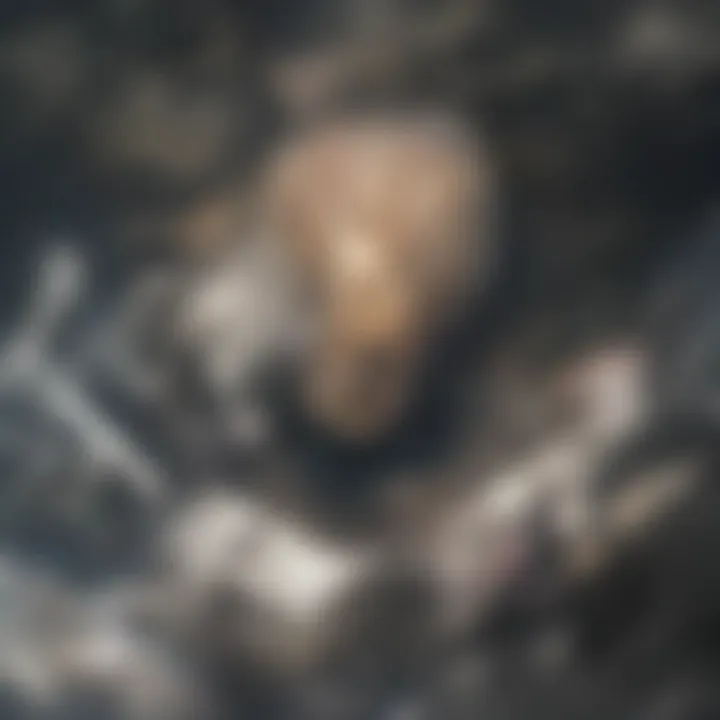
Intro
The electron microscope signifies a pivotal shift in contemporary imaging technology. In a field that has undergone substantial transformations over the last few decades, the latest versions of this instrument push boundaries further than before. This technology now enables scientists to explore the nanoscale realm with unprecedented resolution. As such, various scientific domains are seeing a revolution in how research is conducted, ranging from biology to materials science and nanotechnology.
This discussion aims to elaborate on the workings of the new electron microscope. We will dissect its technical specifications, operational principles, and a range of practical applications. Moreover, the article will consider implications of the advancements this technology presents for future research, allowing a deeper insight for individuals from all background.
Recent Advances
Latest Discoveries
The new electron microscope has already led to significant breakthroughs across multiple disciplines. For instance, in biology, researchers are now able to visualize cellular structures in intricate detail. This capability enhances our understanding of fundamental biological processes, potentially leading to advanced medical treatments and new diagnostic techniques. Key discoveries include:
- Detailed imaging of cellular organelles, improving our grasp on cellular functions.
- Precise analysis of biomolecular structures, empowering drug design processes.
Technological Innovations
The instrumentation itself has evolved dramatically. Modern electron microscopes now feature super-resolution capabilities that allow them to observe phenomena previously deemed unobservable. Some noteworthy innovations include:
- Field Emission Gun (FEG) technology, which provides a brighter electron source for improved contrast.
- Cryo-electron microscopy, allowing samples to be examined in their natural hydrated state, preserving native structures.
Emerging technologies within electron microscopy continue to reshape methodologies. Adaptive optics, combined with enhanced detectors, now facilitate clearer imaging and three-dimensional reconstructions.
Modern electron microscopy not only enhances the resolution but also reduces the time required for image acquisition.
Methodology
Research Design
The operational blueprint of new electron microscopes incorporates a network of meticulously calibrated components. It employs electron optics to focus a beam of electrons, manipulating it with high precision. This design supports advanced imaging techniques that allow researchers to distinguish features at the atomic level.
Data Collection Techniques
Data collection in electron microscopy has also been fine-tuned. The most common method involves:
- Sample Preparation: Samples must be thin enough for electrons to pass through, often requiring intricate preparation techniques.
- Imaging Conditions: Optimization of electron beams and environmental conditions is crucial to obtaining clear images.
- Post-Processing: The captured data undergo extensive analysis using dedicated software, allowing for visualization enhancement.
Understanding these methodologies is essential for researchers aiming to leverage this advanced technology effectively. Improved data gathering techniques lead to a more accurate representation of materials and biological specimens.
Overall, the evolution of the electron microscope exemplifies how technology can drive scientific discovery. By staying abreast of these advancements, professionals and students alike can better navigate the growing complexity of the nanoscale universe.
Prolusion to Electron Microscopes
The electron microscope represents a pivotal development in imaging technology. Its importance lies in its ability to explore the nanoscale world, revealing structures that traditional optical microscopes cannot capture. This section provides an overview of the principles and background essential for grasping the innovations in electron microscopy presented in this article.
Understanding electron microscopes is vital for students, researchers, educators, and professionals. The enhancements and innovations within this field change how various sciences approach research and observation. From biological studies to materials science, the impacts are profound.
Basic Principles of Electron Microscopy
Electron microscopy utilizes a beam of electrons to illuminate a specimen. Unlike light, which has a longer wavelength, electrons have much shorter wavelengths, allowing for a much higher resolution. This fundamental principle enables scientists to resolve details at the atomic level.
When the electron beam hits the sample, it interacts with the atoms, leading to the emission of various signals. These signals can be detected and translated into images. There are two main types of electron microscopes: Transmission Electron Microscopes (TEM) and Scanning Electron Microscopes (SEM). TEM provides information about internal structures, while SEM offers detailed images of the surface.
Key factors such as lens design, vacuum systems, and sample preparation play a crucial role in determining the quality of the images produced. Moreover, the introduced innovations in these areas have significantly improved the capabilities of modern instruments.
Historical Context and Evolution
The development of electron microscopes began in the early 20th century, following the discovery of the electron by J.J. Thomson in 1897. The first operational electron microscope was built in 1931 by Ernst Ruska and Max Knoll. Their invention marked the beginning of a new era in microscopy, enabling scientists to visualize structures smaller than what light could reveal.
Over the decades, advancements have continued to evolve. The introduction of new forms of electron microscopy, such as field-emission guns and spherical aberration correctors, has led to phenomenal increases in resolution and versatility.
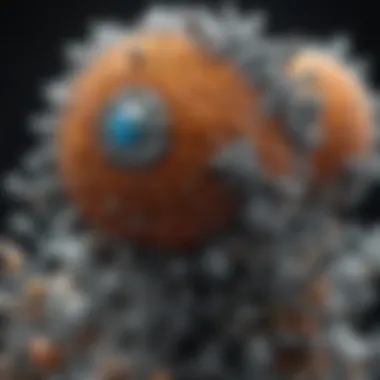
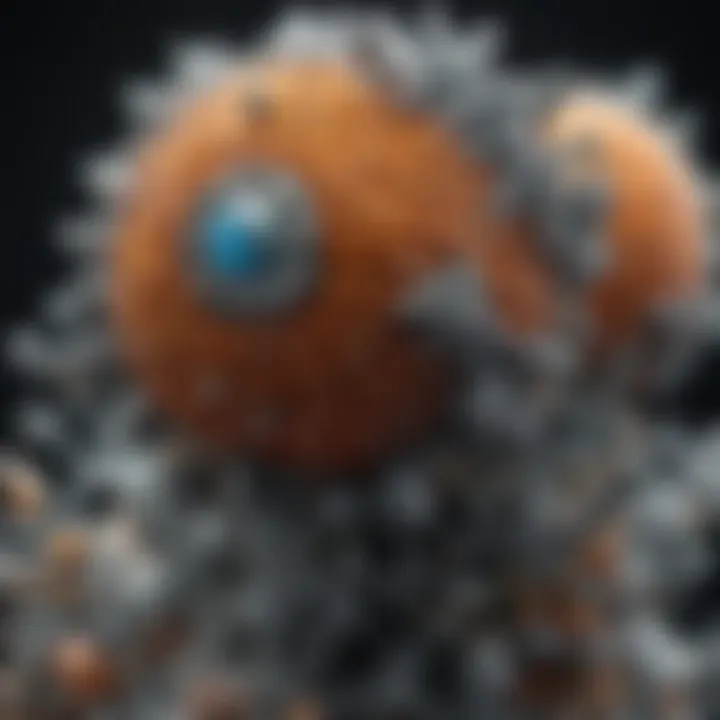
Focusing on the timeline, from the emergence of the initial prototype to today’s sophisticated instruments, it becomes clear how the field has advanced. These developments have enabled a deeper understanding of materials and biological systems, opening avenues for groundbreaking research.
"The electron microscope has changed the way we see the world at the smallest scales, providing insights that were previously unimaginable."
Through exploring these historical milestones, it’s possible to appreciate the significance of the new electron microscope in contemporary research and its implications for future studies. This evolving technology will continue to shape scientific inquiry for years to come.
The New Electron Microscope: Features and Innovations
The introduction of the new electron microscope heralds a significant shift in imaging technology, particularly in how we observe materials at the nanoscale. This segment will examine the essential features and innovations of this technological advancement. Understanding these aspects is crucial as they pave the way for enhanced research capabilities across various scientific fields. The innovations not only improve image resolution but also streamline the entire microscopy experience, from sample preparation to data analysis. This is a pivotal moment for researchers, educators and professionals alike as these innovations redefine methods of investigation and application.
Advanced Resolution Capabilities
One of the standout features of the new electron microscope is its advanced resolution capabilities. Traditional electron microscopes have limitations regarding the smallest details they can observe. However, new models can now achieve resolutions approaching atomic dimensions. This leap in capability allows scientists to visualize previously inaccessible structures with remarkable clarity. For instance, in biological research, it becomes possible to observe cellular components like ribosomes or mitochondria in unprecedented detail.
Furthermore, utilizing advanced electron optics and improved detector technology contributes to this enhancement. The result is a more precise imaging system, which facilitates accurate measurements and analysis.
Improved Sample Preparation Techniques
Sample preparation is often regarded as one of the most challenging aspects of electron microscopy. The introduction of the new electron microscope has brought improvements that simplify and enhance this process. New methods and equipment have been developed, allowing for thinner and more uniform samples. Such advancements ensure that researchers can obtain high-quality images without the artifacts that often come from inadequate sample preparation.
For example, some new electron microscopes employ focused ion beam milling, which allows for better sample thinning whilst maintaining the integrity of the specimen. This improved technique significantly reduces the time spent on preparation and increases the potential for high-fidelity imaging.
Incorporation of Machine Learning
The integration of machine learning into the operations of the new electron microscope represents a paradigm shift for both data analysis and imaging. Machine learning algorithms are capable of processing vast amounts of data generated during microscopy sessions quickly. With the assistance of AI, researchers can identify patterns and features in images that may have been previously overlooked.
This incorporation enables more efficient data handling and enhances the microscope's predictive capabilities. For instance, an AI-enhanced system may predict the conditions required for specific imaging results based on historical data. This level of integration not only increases the productivity of researchers but also helps in extracting more meaningful insights from experiments.
"With the new electron microscope, the integration of machine learning transcends traditional microscopy, enabling a deeper comprehension of materials at the nanoscale."
Operational Aspects of the New Electron Microscope
The operational aspects of the new electron microscope are critical in understanding its overall functions and capabilities. This section explores the key components, comparisons with traditional instruments, and user training needs. Understanding these operational elements helps to appreciate the advancements made with this technology and its overall impact on scientific research.
Key Components and Functionality
The new electron microscope incorporates a variety of components that enhance its functionality. Key elements include the electron source, which produces a beam of electrons; the electromagnetic lenses, which focus the electrons; and the detectors, which capture the information from the sample. Each component works collaboratively to achieve high-resolution imaging.
- Electron Source: Generally, a field emission gun is used. It provides a stable and intense source of electrons, resulting in better image quality.
- Lenses: These lenses are pivotal. They manipulate the electron beam to spotlight the sample, enhancing depth of field and resolution.
- Detectors: Advanced detectors, like the CMOS-based systems, are capable of gathering detailed data quickly. This is essential for both imaging and analysis.
The interplay between these components is vital. Improvements in these technologies help scientists visualize nanoscale structures that were previously inaccessible, paving the way for new discoveries.
Comparison with Traditional Electron Microscopes
When comparing the new electron microscope to traditional models, several distinctions become apparent. Traditional electron microscopes, while effective, have limitations that the new models address.
- Resolution: The new electron microscope achieves superior resolution, allowing for imaging at sub-nanometer levels. Traditional scopes often struggle with resolution, limiting the depth of insights available.
- Sample Preparation: The novel techniques in sample preparation simplify the process. In contrast, traditional methods often demand labor-intensive protocols, thus delaying results.
- User Interface: The new models feature more intuitive interfaces and software. This contrasts with older systems that relied heavily on manual input and extensive training.
These enhancements in technology create a landscape where research can progress at an accelerated pace, offering researchers new tools to examine materials and biological specimens in unparalleled detail.
User Training and Accessibility Issues
While the new electron microscope presents advancements, it also introduces challenges concerning user training and accessibility. As the technology evolves, it is essential for users to familiarize themselves with the operational aspects to utilize it effectively.
- User Training: Comprehensive training programs must be developed. This training should cover both the practical use and an understanding of the underlying principles.
- Accessibility: Access to these advanced microscopes often remains limited due to high costs and infrastructure needs. This can create a gap in capabilities, especially in educational institutions or smaller research facilities.
- Technical Support: Ongoing technical support will be necessary, ensuring that users can troubleshoot and optimize the equipment. This aspect is especially important for new users less familiar with electron microscopy.
Addressing these training and accessibility issues will be paramount in maximizing the potential of the new electron microscope, ensuring that a wide array of researchers can benefit from its capabilities.
Applications of the New Electron Microscope
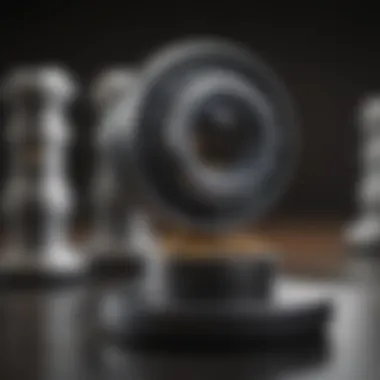
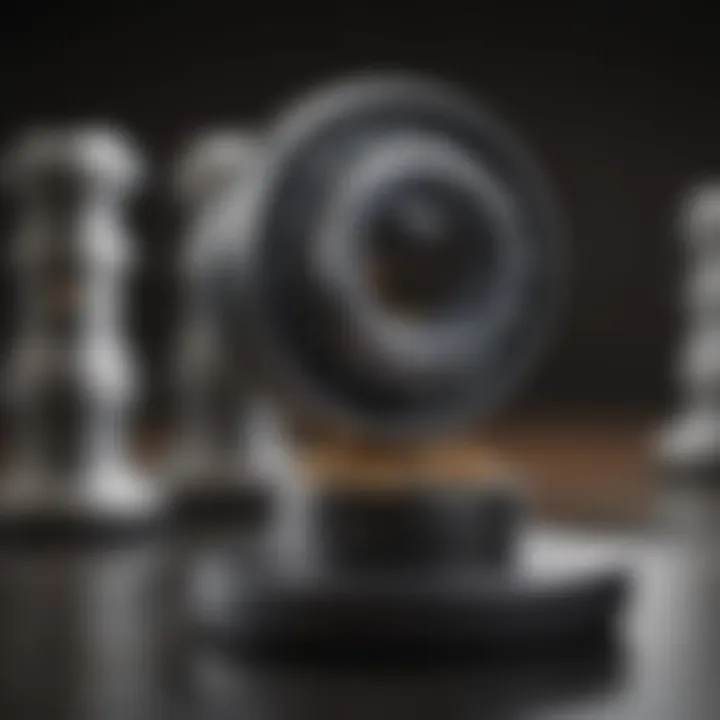
The applications of the new electron microscope are vast and varied, significantly impacting multiple fields including biological research, materials science, and nanotechnology. Understanding these applications illuminates their importance in advancing scientific knowledge and developing technologies. Each application shows how the enhanced features of the new electron microscope foster innovations that can lead to groundbreaking discoveries.
Biological Research Applications
Cellular Imaging
Cellular imaging is a prominent application of the new electron microscope, which provides unprecedented insights into the cellular structure and functions. This aspect is crucial for understanding how cells behave in health and disease. The key characteristic of cellular imaging is its exceptional resolution, allowing scientists to observe organelles and other cellular components in detail. This makes it a beneficial choice when studying complex biological systems.
The unique feature of cellular imaging lies in its ability to generate high-contrast images, capturing the texture and architecture of cellular environments. Its advantage is the detailed level of information it provides, which can lead to new hypotheses in cell biology. However, a disadvantage might be the complexity of sample preparation, often requiring sophisticated techniques to preserve cells without damaging them.
Virus Structure Analysis
Virus structure analysis serves as another pivotal application of the new tool. This facet focuses on elucidating the morphology of viruses at the nanoscale. A prominent characteristic of virus structure analysis is its ability to visualize intricate viral features that are often invisible to traditional microscopy. It is widely recognized for enhancing our understanding of viral infections and developing effective vaccines.
The unique feature of this analysis includes the capacity to reveal how viruses interact with host cells. Its advantages are significant in virology and can potentially lead to therapeutic advancements. Yet, the precision required in sample preparation may also pose a limitation, making standard practices essential for reproducibility of results.
Materials Science Applications
Nanomaterials Characterization
Nanomaterials characterization is crucial in evaluating the properties and behaviors of materials at the nanoscale. This aspect significantly contributes to the fields of engineering and material science. The key characteristic of nanomaterials characterization is that it gives detailed compositional and structural information. This makes it a vital tool for researchers looking to innovate in material properties and applications.
The unique feature is the ability to conduct atomic-level analysis, providing insights into how nanomaterials can be used in technology or medicine. The primary advantage is that such characterization helps in optimizing material performance for specific applications. In contrast, the limitation is the significant expertise required to interpret the data derived from these high-resolution techniques.
Defect Analysis in Crystals
Defect analysis in crystals becomes essential for understanding material imperfections that may affect their functionality. This aspect is crucial for synthesizing high-quality materials and improving their applications in various technologies. The key characteristic of defect analysis is its capacity to pinpoint minute defects within crystal lattices, enhancing our understanding of material reliability.
The unique feature of this analysis is the detailed mapping of defects, which helps researchers correlate these imperfections with material properties. The advantages include improving the performance and durability of materials in engineering applications. Nevertheless, complexities in the detection methods may limit routine usage in less specialized settings.
Nanotechnology Implications
Nanoparticle Analysis
Nanoparticle analysis stands out in the realm of nanotechnology, focusing on understanding the behaviors and properties of nanoscale particles. This aspect contributes significantly to advancements in drug delivery systems and nanomaterials. A notable characteristic of nanoparticle analysis is the ability to measure particles' size, shape, and distribution with high accuracy. This capability renders it beneficial in research that explores the therapeutic applications of nanoparticles in medicine.
The unique feature here is the rapid feedback loop that allows for immediate adjustments in experimental setups. The advantages are manifold, including enhanced targeting capabilities in treatments. The drawback includes potential challenges in ensuring consistency across varied sample types, necessitating careful calibration of techniques used.
Nanoscale Device Development
Nanoscale device development is an emerging and exciting application associated with the new electron microscope. This aspect provides insights into the design and functions of devices that operate at the nanoscale. The key characteristic of nanoscale device development is its focus on the integration of various nanoscale components, facilitating innovations like improved sensors and transistors.
The unique feature is its ability to visualize how components interact at incredibly small scales, driving technological advancements. Its advantages lie in paving the way for new applications across fields such as consumer electronics and biotechnology. However, the challenges of scalability and cost of materials can pose significant barriers to practical implementation, which remains a point of contention for researchers in the field.
"The new electron microscope not only enhances our understanding but also paves the way for next-generation technological solutions across disciplines."
In summary, the applications of the new electron microscope underscore its transformative role in research and technology development. By examining different fields where this advanced instrument can be applied, we see how it fosters innovation and facilitates deeper exploration into both biological and material sciences.
Implications for Future Research
The introduction of the new electron microscope fundamentally shifts the landscape of scientific research. Its advanced capabilities not only enhance the resolution of imaging but also expand our ability to observe and analyze phenomena at the nanoscale. This transformation brings significant implications for future research endeavors across multiple disciplines.
Potential for New Discoveries
The new electron microscope empowers researchers to delve deeper into material structures and biological entities.
- Enhanced Resolution: The microscope's ability to discern finer details allows for observations that were previously unattainable. This could lead to groundbreaking discoveries in fields like biochemistry and nanotechnology.
- Exploration of Unknowns: With this technology, scientists can investigate complex systems and morphologies. New findings in areas such as virus structure are conceivable, as researchers can now visualize morphological changes and interactions.
- Interdisciplinary Collaboration: As capabilities improve, collaborations across disciplines to understand materials and biological processes should grow. Researchers in various fields can share insights, driving innovative research outcomes.
Impact on Educational Practices
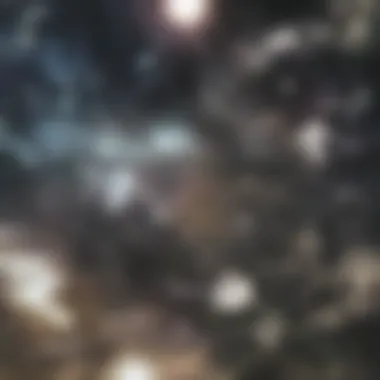
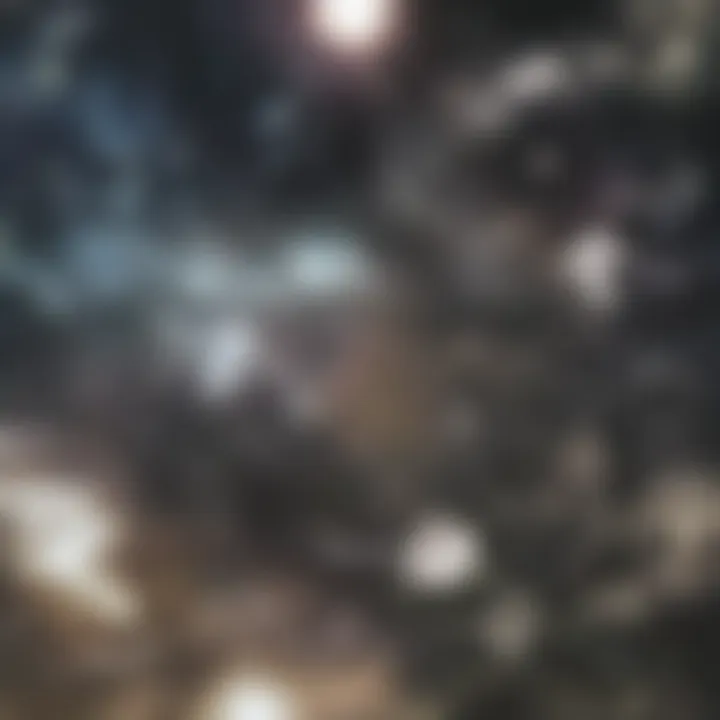
The integration of the new electron microscope in academic institutions carries several implications for educational practices.
- Curriculum Development: It necessitates updates in curriculum to include advanced techniques and technologies. Educators must adapt to ensure students are prepared for research environments where these tools are commonplace.
- Hands-on Learning: This instrument enables practical teaching methods through direct student engagement with cutting-edge technology. Experiential learning opportunities can enhance understanding of complex scientific concepts.
- Research Exposure: Students gain exposure to high-level research practices early in their education. This can spark interest in scientific careers and promote a deeper understanding of modern scientific inquiry.
As these implications unfold, they signify not just enhancements in technology, but a significant evolution in how research is conducted and understood. The new electron microscope thus stands as a critical tool, setting the stage for countless research breakthroughs.
Challenges and Limitations
The exploration of the new electron microscope unravels a mix of opportunities and hurdles. Understanding these challenges and limitations is crucial to fully realize the potential of this sophisticated imaging technology. In this section, we will discuss two primary concerns: the technical limitations inherent in the design and operation of the new microscope and the significant cost associated with its implementation.
Technical Limitations
Despite its remarkable advancements, the new electron microscope is not without its technical constraints. One notable limitation is the requirement for a vacuum environment. Electron beams are easily scattered in air. Therefore, samples must be placed in a vacuum chamber. This need poses logistical challenges in sample handling and can restrict experiments involving living organisms or liquids, as they may not survive under vacuum conditions.
Additionally, resolution enhancements often come with trade-offs in terms of field of view and scanning speed. While the new models can achieve atomic resolution, they may take longer to scan larger areas, which complicates applications in structural biology or materials science where broad area imaging is relevant.
Moreover, there can be adverse interactions between the electron beam and the sample material. Electron beams may cause damage to delicate samples, leading to changes in their structure or properties. This effect must be carefully managed to ensure that the images captured are representative of the true sample state.
High Cost of Implementation
The financial implications of acquiring and maintaining new electron microscopes also present significant barriers. The initial investment required for state-of-the-art equipment is substantial. Instruments like the FEI Titan Krios and Zeiss Sigma require not only a significant capital outlay but also advanced infrastructure, such as specialized laboratories and climate control systems.
Ongoing maintenance costs add another layer of financial burden. Regular servicing and calibration are necessary to maintain the high performance associated with advanced electron microscopes. Furthermore, training personnel to operate these sophisticated tools involves additional costs and time, potentially deterring institutions from adopting the technology promptly.
"Access to advanced electron microscopy is often limited by the high expenses related to acquisition and upkeep. This reality shapes the landscape of research capabilities across various scientific disciplines."
The End
The conclusion section serves a crucial role in summarizing the key themes and insights gained from the exploration of the new electron microscope. It allows readers to reflect on the significant innovations and their implications that have been discussed throughout the article. This summarization is important because it reinforces the understanding of the remarkable technological advancements in electron microscopy.
Recap of Key Insights
In revisiting the essential points of the article, several highlights emerge:
- Unprecedented Resolution: The advancements in resolution capabilities push the boundaries of what is observable at the nanoscale, offering scientists new avenues for discovery.
- Sample Preparation: Improved techniques streamline processes and enhance the reliability of results, which is particularly vital for precise research in various fields.
- Role of Machine Learning: The integration of machine learning enables more efficient data analysis, allowing researchers to extract meaningful information from complex datasets promptly.
- Broad Applications: The new electron microscope has significant implications across several domains, from biological studies to materials science, enabling unprecedented explorations into structures and behaviors at minuscule scales.
- Challenges: Nonetheless, the challenges including high costs and technical limitations still present hurdles that the scientific community needs to address for more widespread adoption.
Future Directions in Electron Microscopy
Looking ahead, the future of electron microscopy appears bright yet complex. Here are some potential directions to consider:
- Enhancement of Imaging Techniques: Ongoing research aims to refine imaging methodologies further, possibly leading to real-time imaging of dynamic processes at the molecular level.
- Broader Accessibility: There is a desire to make these advanced tools accessible to a wider range of researchers and educators, which could democratize scientific inquiry and foster innovation.
- Collaborative Approaches: Increased interdisciplinary collaboration could lead to novel applications and advancements, blending expertise from different fields.
- Sustainability Concerns: Addressing environmental impacts and promoting sustainable practices in the production and operation of electron microscopes is expected to become more prominent.
- Integration with Other Technologies: Further synergizing electron microscopy with technologies like cryo-electron microscopy and atomic force microscopy may expand the scope and impact of imaging capabilities.
"The new electron microscope opens doors to a world of atomic precision, allowing scientists to observe previously inaccessible phenomena."
The evolving landscape of electron microscopy holds great promise. The potential for new discoveries in several scientific disciplines will be paramount in the years ahead. As research in this area continues to flourish, it will be crucial for professionals and students alike to stay informed about these advancements and their implications.
Importance of References
References serve several key purposes. First, they validate the information presented, enabling researchers and professionals to trust the content. Enhanced accuracy is achieved when references are drawn from reputable sources such as peer-reviewed journals, books by established authors, and credible encyclopedias like Britannica or Wikipedia.
Second, they facilitate further exploration. Students and researchers can follow the references to delve deeper into specific aspects of electron microscopy. This can lead to broader comprehension and context for their ongoing studies or projects.
Elements of Good Referencing
When compiling references, it is crucial to consider the following elements:
- Quality of Source: Prioritize peer-reviewed articles and publications from respected institutions.
- Relevance: Ensure that the references directly relate to the subject matter at hand, allowing clear connections between the text and the source.
- Currency: Use the most recent studies and findings, particularly in rapidly advancing fields like microscopy. This ensures that readers access the latest advancements in technology.
- Diversity of Sources: Incorporate a range of resources including articles, books, and reputable online content. This enriches the discussion and presents various perspectives on the topic.
Benefits of Proper Referencing
Properly referencing the new electron microscope contributes to several benefits:
- Increased Credibility: Strong references enhance the trustworthiness of the article, making it a valuable resource for the audience.
- Academic Rigor: Supports a structured and scientific approach to the discussion, essential for readers aiming for advanced understanding.
- Interdisciplinary Engagement: References can span multiple disciplines—biochemistry, materials science, and nanotechnology—appealing to a diverse readership.
Considerations about References
Managing references requires vigilance. It is vital to maintain accuracy in citations, ensuring that all sources are correctly attributed. Misattribution can lead to confusion or misinformation, undermining the article's goals. A well-crafted reference section not only supports the content but also helps the reader in critical analysis of the data presented.