Understanding Gas Exchange: Mechanisms and Implications
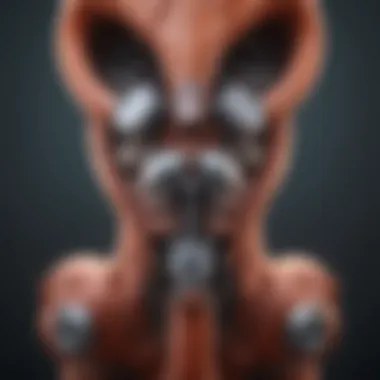
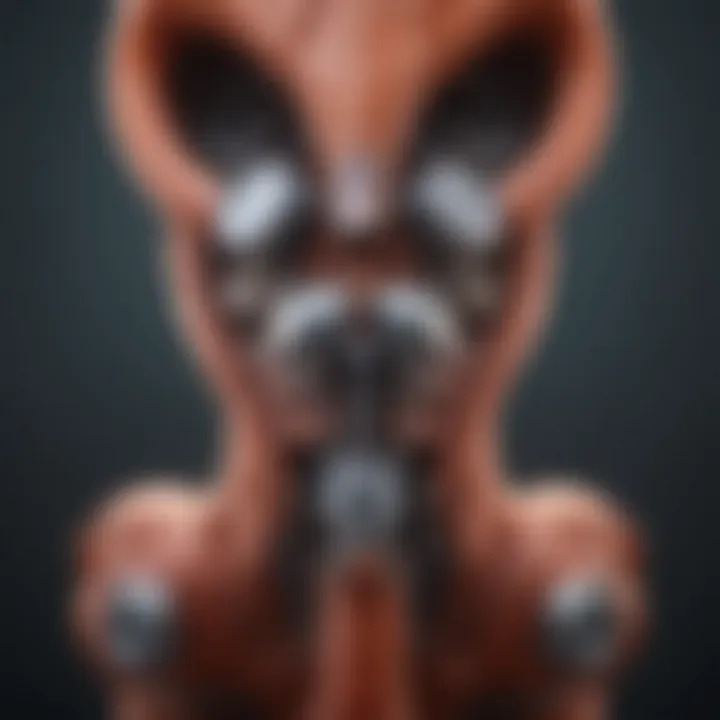
Intro
Gas exchange is a critical biological process that occurs in various living organisms. By allowing the transfer of oxygen and carbon dioxide, it plays a vital role in maintaining life. This article will explore the intricate mechanisms of gas exchange, as well as its importance in physiological contexts and its implications for environmental factors. Through examining gas exchange across different organisms such as mammals, plants, and aquatic life, this exploration underscores the fundamental nature of gas exchange in sustaining life on Earth. Furthermore, with recent advancements in research and technology, a fresh understanding of gas exchange can lead to innovative applications that may help address climate change.
Recent Advances
Latest Discoveries
In recent years, research on gas exchange has revealed complex interactions within biological systems. Scientists have made significant strides in understanding how certain environmental conditions impact gas exchange efficiency. For instance, studies have shown that increased atmospheric CO2 concentrations affect the stomatal behavior in plants, consequently altering their ability to uptake CO2 and release oxygen.
Similarly, investigations into mammalian respiratory systems have illuminated how various lung structures adapt to changes in altitude. This adaptability is vital for survival in distinct environments. These findings illustrate the dynamic nature of gas exchange processes and how organisms optimize these mechanisms based on their surroundings.
Technological Innovations
Technology also plays a pivotal role in unraveling the intricacies of gas exchange. Devices such as portable gas analyzers enable researchers to measure gas concentrations in real-time, bringing new insight into how organisms interact with their environment. For example, the use of satellite imagery is enhancing our understanding of photosynthetic activity and greenhouse gas emissions on a global scale. Advances in molecular imaging techniques are providing clarity on the cellular mechanisms that drive gas exchange.
"Understanding gas exchange will not only improve our knowledge of biological systems but also inform strategies to combat climate change."
Methodology
Research Design
When investigating the mechanisms of gas exchange, a multi-faceted research approach is essential. Comparative studies across species provide data on evolutionary adaptations. Each organism's unique gas exchange system offers valuable context for understanding general principles. This can include analyzing the anatomy of respiratory organs or the efficiency of gas diffusion across membranes.
Data Collection Techniques
Data collection methods in gas exchange research vary based on the focus area. For terrestrial organisms, field studies may include monitoring gas exchange rates with automated systems. In laboratory settings, controlled experiments allow for precise measurements of gas concentrations under different conditions. For aquatic organisms, direct measurements of dissolved gases in water are crucial. Such varied methodologies ensure comprehensive data that informs the overall understanding of gas exchange processes.
Overview of Gas Exchange
Gas exchange is a process essential to the survival of all aerobic organisms. This article addresses its mechanisms and implications, illustrating variations in gas exchange across different biological systems. The study of gas exchange facilitates a deeper understanding of the physiological and ecological aspects of life.
Definition and Importance
Gas exchange refers to the biological processes whereby organisms exchange gases with their environment, primarily involving the intake of oxygen and the release of carbon dioxide. This phenomenon is critical for cellular respiration, providing energy necessary for various metabolic activities. Oxygen serves as a key substrate for the production of adenosine triphosphate (ATP), the energy currency of cells. Conversely, the release of carbon dioxide is vital for maintaining acid-base balance in living organisms.
Moreover, gas exchange plays a pivotal role in ecosystem dynamics. It impacts plant photosynthesis, contributing to oxygen production and the carbon cycle. Understanding gas exchange is not just a matter of biological necessity; it also highlights the interplay between organisms and their environments, especially in the context of climate change and ecosystem health.
Historical Perspectives
The study of gas exchange has a rich history, signifying advancements in biology and physiology. The concept can be traced back to early observations of breathing; however, it gained scientific grounding in the 19th century with the works of notable figures such as Antoine Lavoisier, who identified oxygen as a crucial element for combustion and respiration.
As research progressed, scientists began to reveal the intricacies of gas exchange mechanisms. Pioneers like John Scott Haldane and Auguste Despiau made contributions that formulated the basis of our current understanding. The development of tools like the spirometer further facilitated quantitative studies in respiratory physiology, leading to better insights into how different species accomplish gas exchange.
Over the years, the application of physics to biology offered new perspectives, examining processes like diffusion and the relationship between surface area and gas exchange efficiency. Historical advancements lead to innovations in various fields, from medicine to environmental science, thereby enhancing the relevance of gas exchange study in contemporary research.
Physiological Mechanisms
Understanding physiological mechanisms is essential in comprehending how gas exchange functions in various organisms. The complexity of these mechanisms allows for efficient transfer of gases, ensuring that living beings can properly utilize oxygen and eliminate carbon dioxide. Physiological mechanisms encompass the structures and processes involved in breathing and cellular respiration. This section will delve deeper into respiratory systems and cellular respiration, two key components of gas exchange.
Respiratory Systems
Mammalian Lungs
Mammalian lungs are highly developed organs that serve as the primary means of gas exchange in mammals. The key characteristic of mammalian lungs lies in their ability to facilitate a large surface area for gas exchange due to their intricate structures, which includes the alveoli. Alveoli are small air sacs that maximize the contact area with blood, allowing for efficient oxygen uptake and carbon dioxide release. This structure makes mammalian lungs a prevalent choice for this article, as their efficiency plays a crucial role in sustaining energy levels necessary for daily functions.
A unique feature of mammalian lungs is the presence of surfactant, a substance that reduces surface tension in the alveoli. This prevents the collapse of these tiny structures and ensures that the lungs can expand effectively during inhalation. The advantage is clear: it improves gas exchange efficiency, especially during vigorous activities. However, the reliance on surfactant also presents a disadvantage; any disruption in its production can lead to respiratory complications.
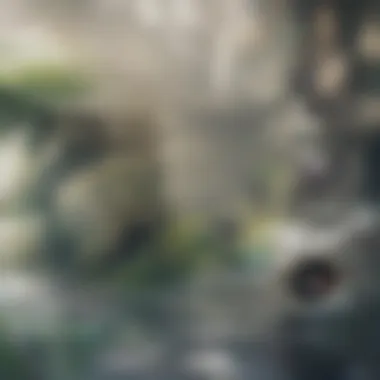
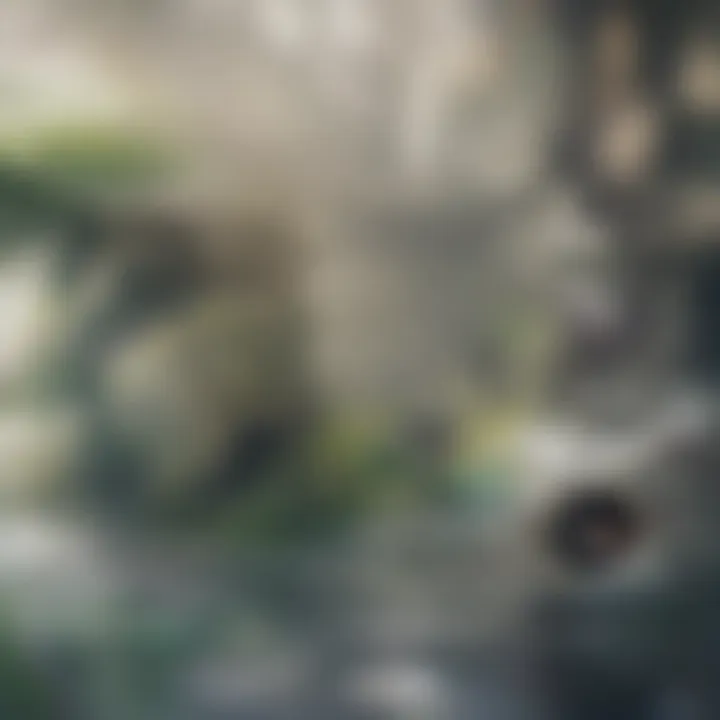
Bird Respiration
Bird respiration stands out through its unique system of air sacs, which allows for a continuous flow of air through the lungs. This is particularly beneficial for high-energy activities like flying, where constant oxygen supply is crucial. The key characteristic of bird respiration is its unidirectional airflow, contrasting with the bidirectional flow seen in mammalian lungs. This mechanism allows birds to extract more oxygen from the same volume of air, making it an efficient choice discussed in this article.
A notable feature of bird respiration is the high efficiency of gas exchange enabled by parabronchi within the lungs. These structures provide an extensive surface area for oxygen absorption. The disadvantage, however, may be the complex anatomical structure that requires more energy and adaptation compared to mammals.
Fish Gills
Fish gills are marvels of adaptive evolution, specifically designed to extract oxygen from water. The gills allow fish to extract dissolved oxygen, a process that is particularly crucial in aquatic environments where oxygen levels can vary. The key characteristic of fish gills is their large surface area combined with a sophisticated system of filaments, which maximizes the diffusion surface for gas exchange. This makes fish gills a vital choice for the context of gas exchange.
A unique feature of fish gills is the counter-current exchange mechanism, which optimizes oxygen absorption. Blood flows in the opposite direction to the water passing over the gills, maintaining a gradient that allows for maximum oxygen uptake. However, fish gills are limited by the requirement of water for breathing, which makes it impossible for these organisms to survive on land, thus highlighting a significant disadvantage.
Cellular Respiration
Cellular respiration is the process through which cells convert biochemical energy from nutrients into adenosine triphosphate (ATP), releasing waste products. This process is integral to understanding gas exchange since it defines how oxygen and glucose are utilized at the cellular level. In aerobic respiration, oxygen plays a crucial role, made possible by efficient gas exchange processes discussed previously.
Overall, physiological mechanisms are an essential aspect of gas exchange. The different respiratory systems and cellular respiration demonstrate how organisms have adapted to their environments while ensuring they meet their metabolic needs. Understanding these mechanisms provides insights into the broader implications of gas exchange on life sustainability.
Gas Exchange in Plants
Gas exchange in plants is essential for their survival and growth, as it directly impacts their ability to photosynthesize and respire. Plants utilize a series of sophisticated mechanisms to manage gas exchange, facilitating the essential processes of converting light energy into chemical energy. This section will explore the importance of gas exchange in plants and how it is intricately woven into their physiological functioning and adaptation to different environments.
Photosynthesis and Respiration
Photosynthesis is a critical process where plants convert sunlight into energy. During this phase, plants absorb carbon dioxide from the atmosphere through small openings called stomata. The absorbed carbon dioxide combines with water, converting it into glucose and oxygen. This oxygen is then released back into the atmosphere, contributing significantly to the planet's oxygen supply.
Respiration, on the other hand, is when plants utilize stored glucose, breaking it down to release energy necessary for growth and development. This dual requirement for both photosynthetic uptake of carbon dioxide and respiratory release of oxygen shows how gas exchange is fundamental in helping plants sustain life and maintain ecological balance.
Stomatal Function
Stomata are microscopic openings located on the surfaces of leaves and stems. They play a pivotal role in controlling gas exchange. The opening and closing of stomata are regulated by guard cells that respond to environmental conditions, such as humidity and light. When the conditions are optimal, stomata open to allow gas exchange; they close in dry or stressful environments to prevent water loss. This dynamic regulation highlights the stomata's dual function: facilitating necessary gas exchange while conserving water. The efficiency of stomatal function can directly affect a plant's growth and overall health.
Adaptations to Environment
Adapting to various environmental conditions is a critical aspect of plant survival. Different strategies for gas exchange have evolved to meet the challenges of varying climates.
Water-retaining Strategies
Water-retaining strategies include adaptations that help plants minimize water loss while maintaining essential gas exchange. A prominent characteristic of these strategies is thickened cuticles and reduced stomatal density, which limit moisture loss.
For instance, certain succulent plants store water in their tissues, allowing them to survive in arid conditions. These adaptations are beneficial in hot climates where drought is frequent. However, they may have disadvantages in environments where water is abundant, as these plants may be less efficient in gas exchange, potentially limiting their growth.
Temperature Regulation
Temperature regulation is crucial for optimizing the process of photosynthesis and respiration within plants. High temperatures can lead to increased transpiration rates, causing water loss and stress. To counteract this, some plants develop strategies such as orienting leaves, creating more surface area for cooling, and utilizing mechanisms like transpiration to regulate internal temperatures. This adaptability allows plants to maintain metabolic activity even when external conditions fluctuate. The unique feature of temperature regulation contributes significantly to a plant's resilience under varying climatic conditions, enhancing their overall survival.
"The ability of plants to regulate gas exchange and adapt to their environments plays a crucial role in their survival and ecological contributions."
In summary, gas exchange in plants is a multifaceted process that is vital for their growth and adaptation. Understanding how plants manage these exchanges can provide insights into their roles in ecosystems and their reactions to environmental changes.
Gas Exchange in Aquatic Organisms
Gas exchange in aquatic organisms is a critical aspect of biology that illustrates how life sustains itself in water. Unlike terrestrial organisms that primarily utilize lungs for breathing, many aquatic animals have evolved specialized structures, mainly gills, to facilitate gas exchange. Understanding the mechanisms behind gas exchange in aquatic environments helps to elucidate how these organisms interact with their surroundings, and it highlights the significance of water quality and oxygen availability in aquatic ecosystems.
Through this section, we will explore the differences between gills and lungs, the unique challenges posed by oxygen levels in water, and the adaptations aquatic organisms have developed to optimize gas exchange.
Gills vs. Lungs
Gills are specialized respiratory organs that extract dissolved oxygen from water. They are often found in fish and other aquatic animals. Unlike lungs, which are adapted for air breathing, gills have a large surface area in relation to their volume, maximizing efficiency in oxygen absorption. The structure of gills includes thin membranes, which allows for a quick transfer of gases.
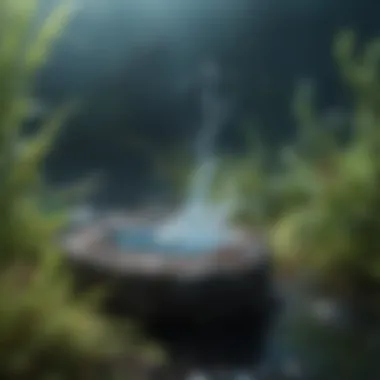
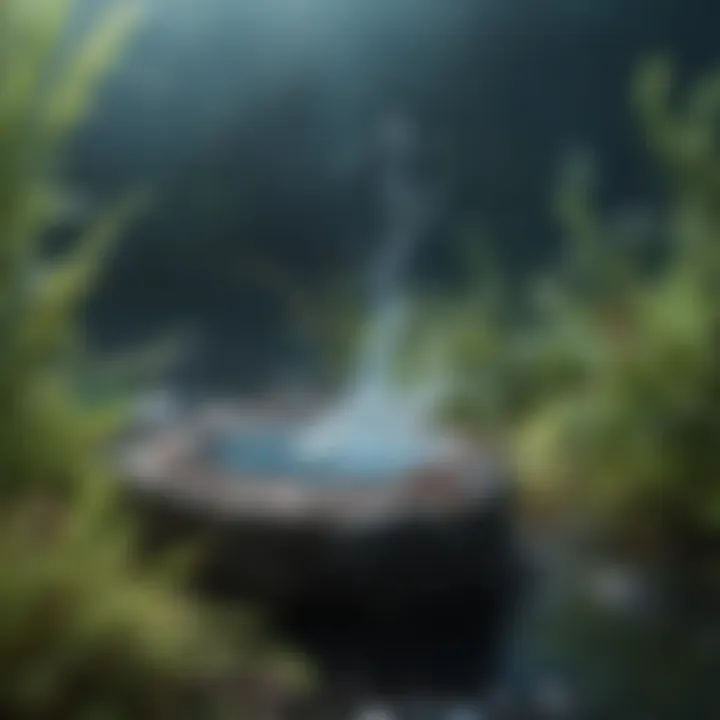
Key points about gills:
- Location: Gills are located on the sides of the head or in pharyngeal slits.
- Functionality: Water flows over the gill filaments, where oxygen is absorbed and carbon dioxide is expelled.
- Adaptations: Some species have developed more efficient gills for low-oxygen environments.
In contrast, lungs are not typical in aquatic organisms but do exist in some species, such as amphibians and certain fish species like the lungfish. Lungs allow these organisms to breathe air, which can be crucial in environments where water lacks sufficient oxygen.
Oxygen Levels in Water
Oxygen levels in aquatic environments can vary significantly due to temperature, salinity, and the presence of organic matter. Generally, water holds less oxygen than air, affecting how aquatic organisms adapt to their environments.
Factors influencing oxygen levels:
- Temperature: Warmer water temperatures lead to decreased oxygen solubility. As temperature increases, oxygen availability may drop, impacting organism survival and metabolism.
- Salinity: Changes in salinity can affect the density of water, influencing the amount of available oxygen. Freshwater organisms face different challenges compared to saltwater species due to these variations.
- Organic Matter: Decomposition of organic materials leads to oxygen depletion, commonly known as "dead zones". Such areas can result in serious ecological consequences, where many species struggle to survive.
Effective gas exchange in aquatic organisms is essential not just for individual survival but for the health of the entire aquatic ecosystem.
Understanding these relationships helps in conservation efforts and in predicting how climate change may impact aquatic life.
Factors Affecting Gas Exchange
Gas exchange is a complex process influenced by various factors. Understanding these factors is crucial for comprehending how organisms adapt and survive in different environments. In this section, we will explore both physical and biological elements that impact gas exchange, enabling better insights into its mechanisms.
Physical Factors
Temperature
Temperature significantly impacts gas exchange rates in organisms. An increase in temperature typically enhances the kinetic energy of gas molecules, resulting in faster diffusion rates. This is beneficial for organisms as it can improve oxygen uptake and carbon dioxide elimination in various environments.
However, the effects of temperature are not uniform across species. Cold-blooded animals, for example, may experience slower metabolic rates at lower temperatures, potentially affecting their gas exchange efficiency. A unique feature of temperature is that while it can enhance gas exchange, extreme heat can also lead to increased rates of evaporation, which might compromise the moisture necessary for effective gas exchange in organisms like amphibians.
Pressure
Pressure plays a pivotal role in gas exchange, particularly in aquatic environments. Higher pressure increases the solubility of gases in liquids, which is crucial for organisms that rely on dissolved oxygen. In terrestrial environments, atmospheric pressure influences the availability of oxygen for breathing.
A fundamental characteristic of pressure is that it varies with altitude, which can affect how efficiently organisms can exchange gases. Unique to this topic is the fact that while low pressure at high altitudes can result in reduced oxygen availability, adaptations such as increased hemoglobin affinity allow certain organisms to thrive despite these challenges.
Humidity
Humidity affects gas exchange primarily by influencing water vapor pressure. High humidity can facilitate gas exchange by reducing the moisture loss through evaporation, allowing for better retention of water in organisms like plants during photosynthesis. Alternatively, too much humidity can also create a saturation point where gas exchange is hindered due to the lack of a gradient for diffusion.
Moreover, a key characteristic of humidity is its direct relationship with temperature and air pressure, which can create unique environmental conditions. For instance, in excessively humid conditions, organisms might experience respiratory distress due to thickened mucus lining their airways, demonstrating how important this factor is in understanding gas exchange.
Biological Factors
Metabolic Rate
The metabolic rate of an organism directly influences gas exchange efficiency. Organisms with higher metabolic rates tend to require greater amounts of oxygen for cellular respiration. Consequently, they have developed specialized adaptations that enhance gas exchange, such as larger lungs or gills.
A significant characteristic of metabolic rate is its variability among species. Endothermic animals, for example, maintain high metabolic rates regardless of environmental temperature, which necessitates efficient gas exchange mechanisms. This raises interesting considerations on how evolutionary pressures shape these adaptations.
Surface Area
Surface area is critical to gas exchange, particularly in respiratory structures. A larger surface area allows for greater contact between circulating fluids and gases, facilitating higher exchange rates. For instance, the alveoli in mammalian lungs increase the surface area available for gas exchange massively compared to the body volume.
The unique feature of surface area is its adaptation in different organisms. Fish gills present a highly folded surface, allowing efficient oxygen extraction from water. However, there can be a disadvantage too; larger surface areas can also lead to increased water loss in terrestrial animals, showcasing the delicate balance in adapting to gas exchange needs across various environments.
Understanding these factors not only provides insights into the physiological processes involved in gas exchange but also sheds light on how organisms interact with their environments and the significance of these interactions in ecosystem dynamics.
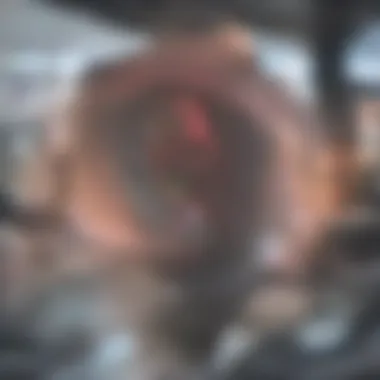
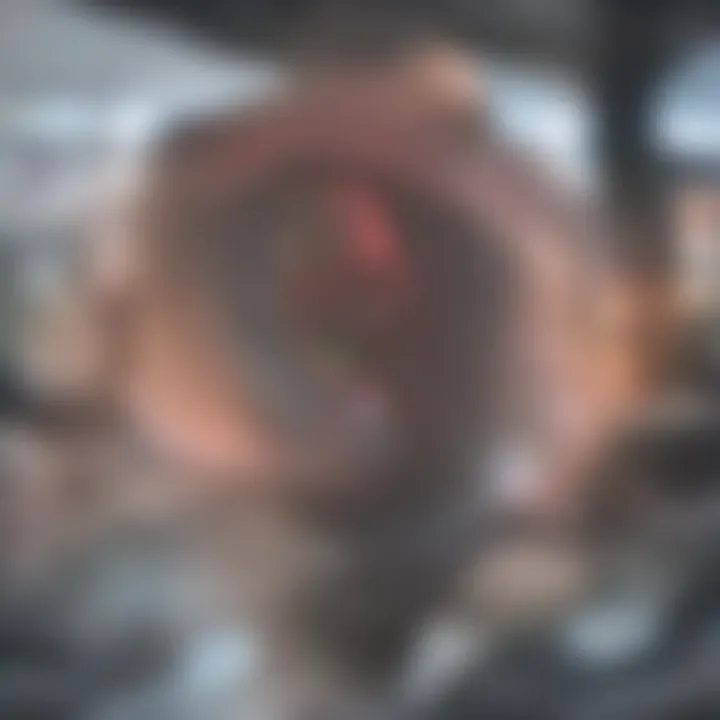
Ecological Implications
Gas exchange has profound ecological implications that extend beyond individual organisms to the interactions within ecosystems. Understanding these implications is vital for grasping the broader impact of gas exchange on ecological balance and health. Observing how different species interact through gas exchange reveals intricate relationships that are essential for sustaining life on Earth.
Impact on Ecosystems
Gas exchange is critical in the functioning of ecosystems. The mechanisms of gas exchange influence plant productivity, which in turn affects the entire food web. For example, in forest ecosystems, trees perform photosynthesis, absorbing carbon dioxide and releasing oxygen. This process plays a key role in maintaining atmospheric balance. Moreover, healthy vegetation supports a multitude of organisms, making this interplay essential for biodiversity.
The oxygen produced during gas exchange not only sustains terrestrial organisms but also supports aquatic life. Aquatic plants engage in gas exchange, releasing oxygen into the water. Fish and other aquatic organisms rely on this dissolved oxygen for survival.
Furthermore, carbon dioxide is a primary component in the biogeochemical cycles, fostering nutrient availability. A disruption in gas exchange can lead to ecological imbalances, such as increased levels of carbon dioxide leading to climate change, which can deteriorate habitat conditions.
"Healthy ecosystems depend on efficient gas exchange mechanisms that regulate the flow of gases, nutrients, and energy."
Climate Change Effects
The relationship between gas exchange and climate change cannot be overstated. Increased carbon dioxide emissions from human activities directly impact ecosystems by enhancing the greenhouse effect. As carbon levels rise, gas exchange processes in plants and oceans are affected. Plants may increase their photosynthetic rates initially, but as conditions change, their ability to sequester carbon could diminish.
Moreover, oceanic gas exchange is altered when temperature rises or when water becomes more acidic. Higher temperatures can reduce oxygen levels in water, directly affecting marine life.
In summary, ecological implications of gas exchange reveal intricate connections that influence the health of ecosystems. Addressing the challenges of climate change requires understanding these mechanisms and their effects on gas balance, biodiversity, and overall ecological integrity. A careful examination of these relationships offers crucial insights to aid conservation efforts and improve our responses to climate-related challenges.
Research and Innovations
Research and innovations play a critical role in understanding gas exchange and its implications across various biological systems. With the increasing emphasis on climate change and environmental sustainability, innovations in this field are essential. They provide insights that not only advance scientific knowledge but also inform practical applications in health, agriculture, and conservation. Research can reveal how organisms adapt their gas exchange mechanisms to changing environmental conditions, which can lead to broader implications for ecosystem health and resilience.
Advancements in Technology
The advancements in technology have significantly transformed our understanding of gas exchange processes. Tools such as mass spectrometry and imaging techniques have allowed researchers to study gas exchange at both the cellular and whole-organism levels. These technologies enable precise measurements of oxygen and carbon dioxide exchanges, providing clearer insights into metabolic rates and respiratory functions. For example, instrumentations that analyze gas concentrations can facilitate research in adaptation among different species.
Moreover, innovations in data analytics and modeling create opportunities to simulate gas exchange mechanisms. This allows scientists to predict how changes in environmental factors like temperature or pressure may impact these processes. The integration of real-time monitoring systems can lead to breakthroughs in understanding the dynamics of gas exchange, particularly in habitats undergoing rapid changes due to human activity.
Future Directions in Gas Exchange Studies
Future directions in gas exchange studies point toward greater interdisciplinary collaboration. This includes the integration of ecological, physiological, and technological insights. One significant focus could be on the relationship between gas exchange and climate adaptability. Researchers may explore how species with efficient gas exchange mechanisms can contribute positively to ecosystem stability.
Another promising avenue is the use of genomic and proteomic technologies to understand the molecular underpinnings of gas exchange. Investigating how certain genes and proteins are expressed in response to varying atmospheric conditions could yield vital knowledge. This may enhance our capacity to predict responses to climate change.
The potential for biotechnological solutions to enhance gas exchange efficiency in crops could lead to more sustainable agricultural practices, addressing food security while minimizing environmental impacts.
Overall, continued investment in research and innovations is crucial. As we face unprecedented challenges posed by climate change, a deeper understanding of gas exchange mechanisms will be fundamental in identifying solutions for ecological health and resilience.
End
The conclusion serves an essential role in reinforcing the significance of gas exchange, intricately weaving together the insights shared throughout this article. Understanding both the mechanisms and implications of gas exchange transcends mere academic interest; it holds real-world relevance in areas such as healthcare, environmental sustainability, and ecological balance.
Key elements of this conclusion include:
- Summary of fundamental processes: The physiological mechanisms of gas exchange in various organisms provide insights into how life sustains itself through the constant exchange of gases. This includes nuances in mammalian lungs, avian respiratory systems, and aquatic gills.
- Relevance to ecological health: The implications of gas exchange extend to understanding ecosystem dynamics. Changes in gas exchange processes can affect entire ecological communities, influencing species distribution and ecosystem functionality.
- Impact on human health: The understanding of how gas exchange operates remains vital for medical science. Pathologies such as Chronic Obstructive Pulmonary Disease (COPD) or asthma reveal the critical nature of proper gas exchange and its direct correlation to health and wellness.
- Adaptation to climate change: As we confront the urgent challenge of climate change, understanding gas exchange mechanisms can lead to innovative solutions in mitigating its effects. Evaluating how various organisms adapt their gas exchange processes in response to environmental stressors could inform strategies for conservation.
The consequences of our findings may extend into policy-making, particularly concerning environmental regulations and public health initiatives. Thus, the implications of gas exchange research are not merely academic; they resonate across various sectors, highlighting the need for continued inquiry and exploration.
Summary of Key Points
In summary, this article reinforces several crucial points:
- Critical Role of Gas Exchange: It is fundamental for all living organisms, facilitating the transfer of oxygen and carbon dioxide.
- Physiological Diversity: Different systems have evolved unique adaptations for effective gas exchange, such as mammalian lungs, bird respiration, and aquatic gills.
- Environmental Interdependencies: Factors like temperature, pressure, and humidity play significant roles.
- Impact on Ecosystems: Gas exchange is integral to ecological balance, influencing species interactions and biodiversity.
- Future Directions: Innovations in research can lead to advancements in both technology and our understanding of life processes in various environments.
Implications for Future Research
The study of gas exchange opens numerous avenues for further exploration. Future research might focus on:
- Advanced Technologies: Innovations such as machine learning and biotechnology can enhance our comprehension of gas exchange mechanisms.
- Climate Adaptations: Investigating how species adapt their gas exchange processes under changing environmental conditions provides insights necessary for conservation efforts.
- Interdisciplinary Approaches: Integrating knowledge from biology, environmental sciences, and medicine could lead to holistic understandings.
- Public Health Policies: Research findings can influence policy, emphasizing the need for improved air quality and its effects on human health.
- Biodiversity Conservation: Studies might examine the relationship between gas exchange efficiency and species resilience, aiding conservation strategies.
The dynamic nature of research in gas exchange not only enhances scientific understanding but also underscores its practical implications for global challenges such as climate change and biodiversity loss.