Understanding the Genetic Roots of Disease
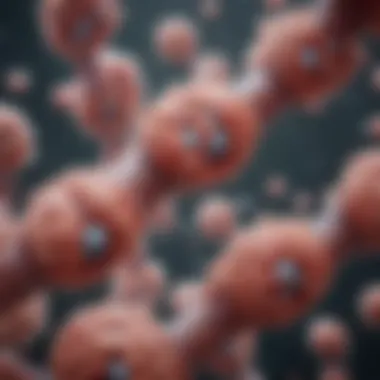
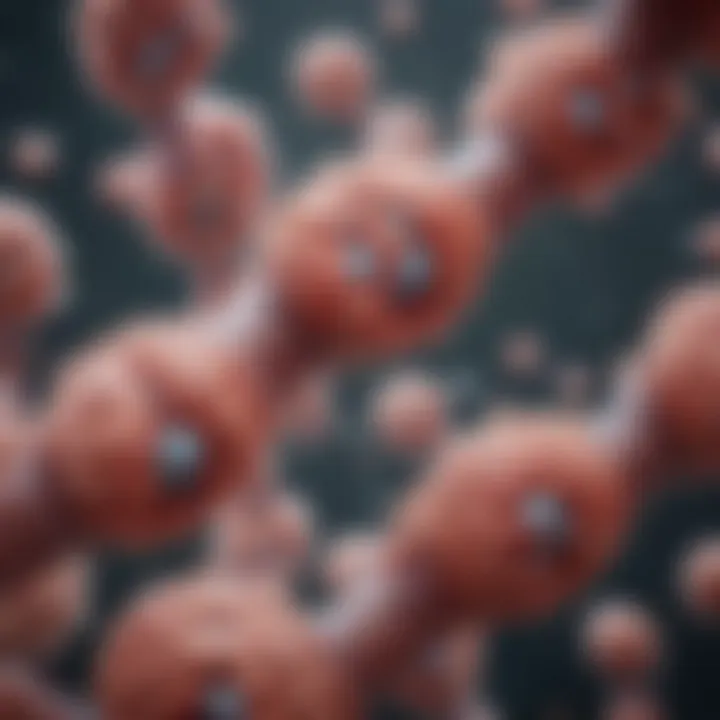
Intro
Genetic diseases represent a significant area of study in modern biology and medicine. Understanding the genetic basis of these disorders is critical for developing effective treatments and prevention strategies. This article examines the intricate mechanisms at play in genetic diseases, emphasizing the contributions of mutations, inherited traits, and chromosomal abnormalities. By investigating various examples, from cystic fibrosis to Down syndrome, readers will gain insights into how genetic factors shape health outcomes.
Recent Advances
Latest Discoveries
Recent advancements in genomic medicine have shed light on the underlying causes of numerous genetic disorders. Thanks to next-generation sequencing technologies, researchers can now identify mutations at an unprecedented scale. For instance, the discovery of the CFTR gene mutation linked to cystic fibrosis has revolutionized our understanding of this condition and paved the way for targeted therapies.
Moreover, researchers are uncovering the polygenic nature of various diseases. Complex conditions such as diabetes and schizophrenia have shown intricate interactions among multiple genes, highlighting the need for a broader approach to genetic research.
Technological Innovations
Technological developments, particularly CRISPR, have transformed genetic research. This powerful tool allows for precise editing of DNA, providing researchers the means to study gene functions directly. By manipulating specific genes, scientists can create disease models to observe effects and test potential treatments.
Furthermore, advancements in bioinformatics have enhanced our ability to analyze vast amounts of genetic data. These innovations facilitate the analysis of genetic variants associated with diseases, leading to more tailored therapeutic approaches. The integration of machine learning algorithms has also become increasingly relevant in predicting disease risk based on genetic profiles.
Methodology
Research Design
Research in genetics often employs a mix of observational and experimental methodologies. Large cohort studies help identify associations between genetic markers and diseases, while controlled laboratory experiments clarify the functional consequences of specific mutations.
Data Collection Techniques
Data collection involves various methods, including:
- DNA Sequencing: This technique allows for the identification of mutations in specific genes.
- Family Studies: These studies assess the inheritance patterns of diseases, providing insights into genetic risk factors.
- Population Genetics: Analyzing genetic diversity within populations helps identify common variants associated with disease susceptibility.
With ongoing advancements, the methodology in genetic research continually adapts, ensuring a comprehensive understanding of genetic disorders.
Genetic Foundations of Disease
The study of genetic foundations of disease is crucial for understanding the mechanisms that underlie numerous health conditions. This foundation lays the groundwork for the entire field of genetics and offers insights into how genetic and environmental factors coexist to shape health outcomes. Understanding these genetic elements can aid in early diagnosis, personalized treatment plans, and potential preventive measures against diseases.
When we talk about genetic diseases, we primarily focus on how different mutations and chromosomal aberrations manifest into visible symptoms. This understanding guides medical professionals in determining effective treatments and empowers researchers to develop new therapies. Thus, grasping the basics of genetic diseases opens doors to innovations that could change the way we approach healthcare.
Preface to Genetic Diseases
Genetic diseases arise from variations in genes. These variations can be as simple as point mutations, which involve a single nucleotide change, or as complex as large chromosomal rearrangements. Some genetic diseases are clearly inherited, while others may happen due to new mutations in an individual's DNA. This complexity is essential to understand as it influences how these diseases are diagnosed and treated.
Furthermore, genetic diseases can affect any organ or system, causing a wide array of symptoms, which makes them significant in medical practice and research. Raising awareness and understanding can improve medical responses and individual support networks for those affected.
Types of Genetic Diseases
Understanding the types of genetic diseases can clarify how they differ in origin and impact. Broadly, these can be categorized into monogenic, polygenic, and chromosomal disorders.
Monogenic Disorders
Monogenic disorders result from mutations in a single gene. This simplicity allows for targeted approaches in both diagnosis and treatment. The key characteristic of monogenic disorders is that they often follow clear inheritance patterns, making it easier for genetic counselors to inform patients about risks.
A well-known example is cystic fibrosis, caused by mutations in the CFTR gene. The unique aspect of monogenic disorders is their relatively straightforward genetic basis. However, they can have severe manifestations, leading to significant morbidity. The advantage of focusing on these disorders in genetic research is the potential for precise therapies, such as gene replacement or editing.
Polygenic Disorders
Unlike monogenic disorders, polygenic disorders arise from the cumulative effect of variations in multiple genes. This complexity makes them more challenging to study, as they do not follow simple inheritance patterns. Common examples include conditions like diabetes and heart disease.
The distinct characteristic of polygenic disorders is the interplay of environmental factors with genetic predispositions. This creates a multifaceted picture of disease risk that enriches the field of epidemiology and public health. However, the disadvantage lies in the difficulties of genetic testing, as many contributing genes may only slightly increase the risk.
Chromosomal Disorders
Chromosomal disorders exemplify problems caused by structural changes in chromosomes. These may involve deletions, duplications, or translocations, resulting in disorders like Down syndrome or Turner syndrome. The key feature of chromosomal disorders is their potential to impact a large number of genes at once, often leading to various developmental and health issues.
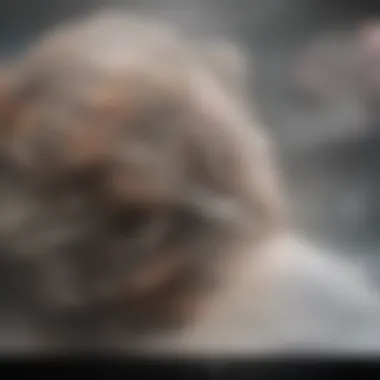
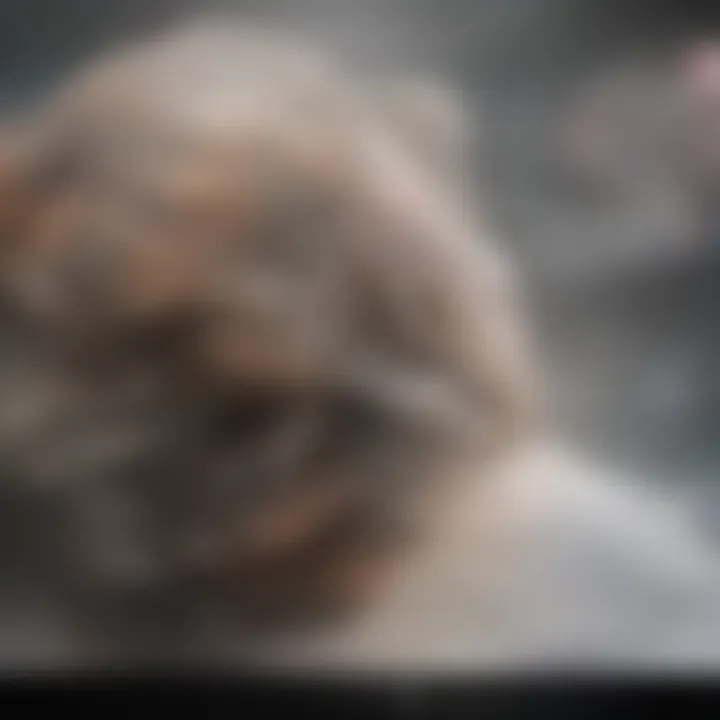
These disorders provide a broader context about genetic abnormalities and their varied effects on development and health. Their complex nature presents unique challenges in detection and management. Understanding these issues informs initiatives aimed at improving diagnostic techniques and patient outcomes.
"Genetic diseases reflect a spectrum of conditions ranging from straightforward Mendelian inheritance to complex multigenic and environmental interactions."
Mechanisms of Genetic Disease
The importance of understanding the mechanisms of genetic disease lies in its potential to illuminate how genetic abnormalities lead to specific health conditions. By examining these underlying mechanisms, we gain valuable insights into the causes of diseases, which can inform prevention strategies and treatment options. Knowledge of genetic mechanisms can also enhance our understanding of how diseases may present differently among individuals, influenced by factors such as gene-gene interactions, environmental conditions, and lifestyle choices.
Molecular Basis of Genetic Mutations
Point Mutations
Point mutations represent a subtle yet significant variation in the DNA sequence, where a single nucleotide is altered. This type of mutation can lead to changes in amino acids within proteins, potentially modifying their function. Point mutations are significantly important when discussing genetic diseases because they can trigger a chain reaction that results in serious health conditions.
The key characteristic of point mutations is their simplicity; they involve a single base pair change. This makes them a beneficial choice for study since the impacts can often be traced more easily than with larger genetic alterations. However, their interpretation may vary. While some point mutations could be harmful, others may not have any noticeable effect on the organism, distinguishing them as a unique feature in genetic research. Understanding point mutations assists in delineating the molecular pathogenesis behind many hereditary diseases.
Insertions and Deletions
Insertions and deletions, often referred to as indels, occur when nucleotides are added or removed from the DNA sequence. This can lead to a shift in the reading frame during the translation of genes, altering the resulting protein structure and function. The significance of indels cannot be understated, especially in their contribution to genetic disorders like cystic fibrosis and muscular dystrophy.
The key characteristic of insertions and deletions lies in their potential for causing significant changes in downstream protein products. This makes them a popular topic for genetic studies as they often correlate with observable phenotypic variations. The unique feature of indels is they can range in size from a few nucleotides to larger sections of DNA. The downside is that larger deletions can result in more severe diseases, while smaller alterations may only have subtle effects, making interpretations intricate in certain cases.
Copy Number Variants
Copy number variants (CNVs) consist of larger segments of the genome that are duplicated or deleted, offering a distinct approach to understanding genetic complexity. CNVs can encompass various lengths and may involve whole genes or non-coding regions. Their contributions to genetic diseases are critical since these variants have been associated with conditions like schizophrenia and autism spectrum disorders.
A key characteristic of CNVs is their ability to affect gene dosage — the number of copies of a gene present in the genome. This makes CNVs an interesting area of focus in genetic research as they reflect a broader spectrum of genetic variation. The advantage of studying CNVs lies in their potential role in evolutionary adaptations. However, their association with disease phenotypes emphasizes the precarious balance between genome flexibility and stability.
Inheritance Patterns
Autosomal Dominant
Autosomal dominant inheritance is characterized by the presence of one mutated copy of a gene being sufficient to cause the manifestation of a disease. This aspect significantly contributes to understanding diseases such as Huntington's disease and Marfan syndrome. The defining characteristic of this pattern is that affected individuals usually have an affected parent, which makes tracking inheritance more straightforward in families.
The benefit of focusing on autosomal dominant disorders is the relative simplicity of inheritance that aids in both diagnosis and genetic counseling. The unique feature here is the potential for incomplete penetrance, where not all individuals with the mutation exhibit symptoms. This complexity can pose challenges in predicting disease occurrence within families.
Autosomal Recessive
In contrast to autosomal dominant patterns, autosomal recessive disorders arise when two copies of a mutated gene are needed to express the disease phenotype. This is crucial for understanding conditions like cystic fibrosis and sickle cell anemia. The key characteristic is that carriers, possessing only one copy of the mutation, do not exhibit symptoms but can pass the mutation to offspring.
The benefits of autosomal recessive studies are clear as they offer insight into carrier prevalence and population genetics. The unique challenge is identifying carriers, as they are often asymptomatic. This makes education on genetic risks essential for disease prevention.
X-Linked Inheritance
X-linked inheritance pertains to genes located on the X chromosome and reveals unique patterns in genetic disease transmission, especially impacting males more than females. This characteristic makes X-linked disorders, such as hemophilia and Duchenne muscular dystrophy, essential for understanding gender-specific disease risks.
The critical aspect of X-linked inheritance is that males are more likely to express the phenotype due to having a single X chromosome, while females can be carriers with one mutant allele. This differential impact highlights the importance of studying X-linked patterns in genetic research. The uniqueness lies in potential female carriers often being asymptomatic, raising considerations for genetic counseling and family planning.
Environmental Influences on Genetic Expression
Understanding environmental influences on genetic expression is crucial for a thorough comprehension of genetic diseases. Genetic predispositions exist, but they interact with various external factors. These factors can range from lifestyle choices to exposure to pollutants, and they can significantly alter how genes are expressed. This interaction between genes and environment underlies many health conditions and diseases, such as asthma or certain cancers.
There are important components of these interactions that we can observe:
- Lifestyle Factors: Diet, exercise, and habits such as smoking can change gene expression. A balanced diet might enhance the expression of beneficial genes.
- Toxins: Chemicals in the environment may lead to mutations. For example, heavy metals can interfere with DNA repair processes.
- Microbiome Influence: The gut microbiota has been shown to affect the host's gene expression. This can impact everything from immunity to metabolism.
By addressing these elements, we can identify both preventative measures and potential treatment strategies for genetic diseases. This section lays the groundwork for understanding how genetics is not solely determined by inherited DNA but is also impacted by a vast array of environmental factors.
Gene-Environment Interactions
Gene-environment interactions refer to how environmental factors influence the traits associated with genetic predispositions. For example, individuals with a genetic predisposition for Type 2 diabetes may not develop the disease if they maintain a healthy lifestyle. Meanwhile, those who do not manage their environment might trigger the condition.
This interaction can complicate disease prevention and treatment. Understanding these interactions can lead to more personalized medicine and targeted interventions. Additionally, analyzing these interactions can help in identifying at-risk populations based on their environments and backgrounds.
Epigenetic Factors
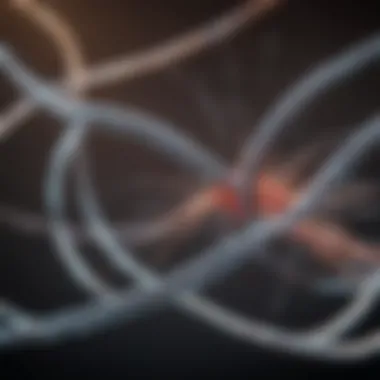
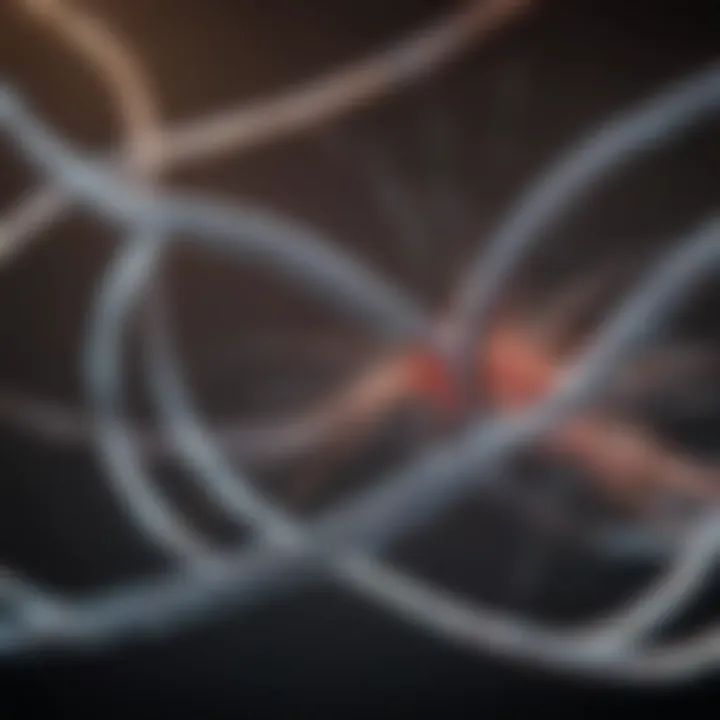
Epigenetic factors play a significant role in determining how genes are expressed without altering the DNA sequence itself. These factors include chemical modifications that can turn genes on or off, impacting how cells function.
DNA Methylation
DNA methylation is a process where methyl groups attach to DNA, affecting gene expression. This modification can silence a gene, reducing its activity. It is important because it provides a mechanism through which environmental factors can influence gene activity.
Key characteristics of DNA methylation include:
- It is reversible, which allows for dynamic changes in gene expression without altering the genetic code.
- It can help explain phenomena such as the development of diseases over a person's life, based on early life conditions and exposures.
The benefit of including DNA methylation in this article lies in its role as a modifiable factor in health. Understanding how environmental influences can adjust methylation patterns offers potential pathways for therapeutic interventions.
Histone Modification
Histone modification involves the alteration of histone proteins, which help package DNA in the cell nucleus. These modifications can either facilitate or hinder the access of transcription machinery to DNA, directly affecting gene expression.
One key characteristic of histone modification is its diversity. Many types exist, including acetylation and phosphorylation, each influencing gene activity differently. This variety allows histone modification to play a crucial role in controlling genetic expression in response to various stimuli such as stress or diet.
While providing numerous advantages, histone modification also poses challenges in understanding disease mechanisms completely. Improper modifications could lead to issues including cancer. Recognizing the significance of histone modifications can enhance understanding of the complex interplay between genetics and environment.
Examples of Genetic Diseases
In the study of genetic diseases, examining specific examples is crucial. These examples illuminate various mechanisms by which genetic disorders manifest. Understanding these diseases provides insight into their genetic basis, effects on individuals, and implications for healthcare and society. Cystic fibrosis, sickle cell disease, Down syndrome, and Huntington's disease exemplify diverse genetic conditions. Each of these cases highlights unique genetic mutations, inheritance patterns, and the influence of environmental factors. Moreover, they inform ongoing research and the development of therapeutic strategies.
Discussing these diseases not only raises awareness but also emphasizes the importance of genetic counseling and screening. Early diagnosis can lead to better management and improved life quality for affected individuals. This narrative explores these conditions in detail, contributing to a holistic understanding of genetic diseases.
Cystic Fibrosis
Cystic fibrosis is a severe genetic disorder caused by mutations in the CFTR gene. This gene plays a vital role in the production of a protein that regulates salt and water movement across cell membranes. Mutations result in thick, sticky mucus accumulation in various organs, particularly the lungs and digestive system. The impact on pulmonary function is significant, leading to frequent respiratory infections, persistent cough, and reduced lung capacity.
The current life expectancy of individuals with cystic fibrosis has improved due to advances in treatment, including enzyme replacement therapy and inhaled medications. However, challenges remain as individuals navigate chronic health issues. Genetic testing has become essential in identifying carriers and confirming diagnoses.
Sickle Cell Disease
Sickle cell disease is another notable genetic disorder characterized by abnormal hemoglobin. The mutation in the HBB gene leads to the production of sickle-shaped red blood cells. These abnormal cells cause pain, anemia, and increased susceptibility to infections. Episodes of pain, commonly known as sickle cell crises, can severely affect the quality of life.
Management strategies focus on relieving symptoms and preventing complications. Hydroxyurea, a medication that increases fetal hemoglobin production, proves beneficial in reducing crisis frequency. Blood transfusions and other supportive therapies are common components of care.
Down Syndrome
Down syndrome, or trisomy 21, arises from the presence of an extra copy of chromosome 21. This genetic anomaly is associated with distinct physical features and cognitive challenges. Individuals with Down syndrome often face developmental delays along with health issues like heart defects and gastrointestinal problems.
Early intervention services, including therapies and educational support, significantly enhance outcomes for individuals with Down syndrome. Awareness of this condition fosters inclusivity and understanding, which is crucial for social acceptance and support.
Huntington's Disease
Huntington's disease is an inherited neurodegenerative disorder caused by mutations in the HTT gene. This disease affects movement, cognition, and behavior, often appearing in mid-adulthood. The progressive nature of Huntington's disease leads to substantial loss of function over time, impacting not only the individual but also their families, who often bear the emotional and practical burdens of care.
Genetic testing has major implications for at-risk individuals. While there is currently no cure, therapies aim to alleviate symptoms and improve quality of life. Awareness and research are vital for understanding the complex genetics underlying Huntington's disease.
Advancements in Genetic Research
Advancements in genetic research have significantly transformed our understanding of genetic diseases. As science progresses, methodologies evolve, leading to improved diagnostic, preventative, and therapeutic strategies. This section focuses on two prominent areas: CRISPR technology and various genetic screening techniques. Together, they represent critical components that enhance our ability to combat genetic disorders and address their underlying causes.
CRISPR and Gene Editing
CRISPR technology revolutionized genetic research by introducing precise gene editing capabilities. It allows scientists to target specific sequences within the genome, enabling accurate modifications. This method can be used to correct genetic mutations that lead to diseases, making it a potential game-changer in treatment approaches.
Some benefits of CRISPR include its cost-effectiveness and efficiency compared to previous techniques. Researchers can achieve results faster, making it feasible for widespread application in clinical settings. However, ethical considerations complicate gene editing. The risks of unintended consequences from gene modifications must be thoroughly evaluated before widespread use.
Genetic Screening Techniques
Genetic screening techniques have become essential in identifying predispositions to genetic conditions before symptoms manifest. They allow for informed decision-making regarding treatment and management strategies. Two notable methods are Preimplantation Genetic Testing and Carrier Testing.
Preimplantation Genetic Testing
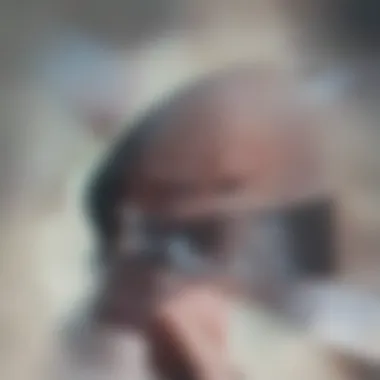
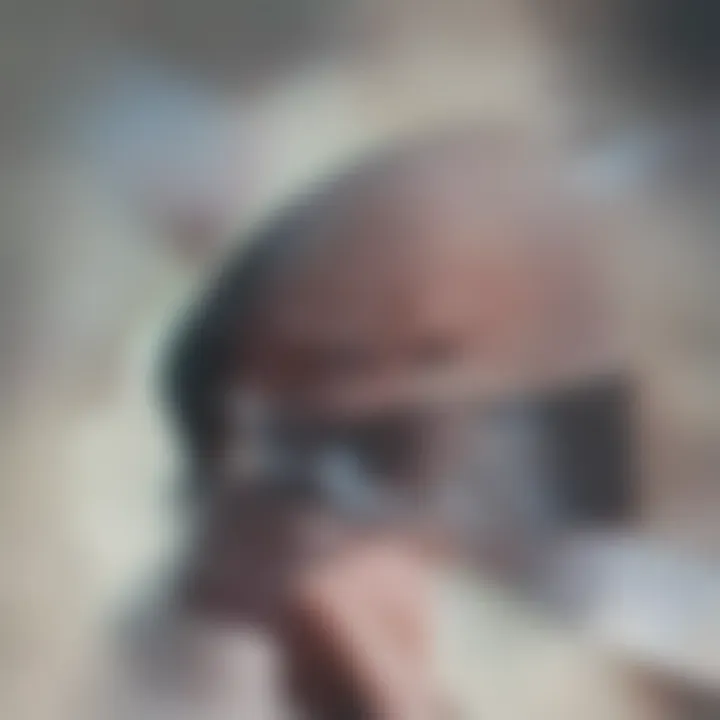
Preimplantation Genetic Testing (PGT) is a procedure used during in vitro fertilization. It enables the detection of specific genetic abnormalities in embryos before implantation. This aspect of PGT is crucial as it can prevent the transmission of genetic disorders to offspring.
The key characteristic of PGT is its ability to screen multiple embryos, increasing the chances of selecting a healthy embryo for implantation. This makes it a popular choice among those at risk of hereditary diseases. However, PGT is not without issues. Its costs can be prohibitive, and there is a potential for ethical dilemmas surrounding embryo selection.
Carrier Testing
Carrier Testing is a genetic test that determines whether an individual carries a one-copy mutation for a recessive genetic disorder. It plays a vital role in family planning, providing essential information to prospective parents about the risks of passing a genetic condition to their children.
A major advantage of Carrier Testing is its broad applicability to numerous conditions. This accessibility enhances awareness of genetic risks in families. Nevertheless, like PGT, Carrier Testing presents certain challenges. Some individuals may experience anxiety regarding the results, and the implications of being identified as a carrier can be complicated.
"Advancements in genetic research not only promise to improve treatment options but also raise significant ethical and social considerations that require careful navigation."
In summary, the advancements in genetic research, particularly in CRISPR and genetic screening techniques, have reshaped the landscape of genetic disease understanding and management. These methodologies provide powerful tools, although they come with ethical dilemmas and other considerations that ongoing discussions must address.
Ethical Considerations in Genetic Research
Genetic research represents a frontier of scientific inquiry that has the potential to dramatically alter our understanding of health and disease. However, examining the ethical considerations involved is essential to ensure that progress does not come at the expense of individual rights and societal norms. The significance of ethical concerns cannot be overstated as they influence public trust, regulatory policies, and the course of research itself.
When discussing the ethical implications in genetic research, several key factors arise:
- Respect for Persons: This principle emphasizes informed consent. Individuals participating in genetic studies must have a clear understanding of the purpose, risks, and benefits of the research. Ensuring that participants can make informed decisions is fundamental in maintaining ethical standards.
- Beneficence: Genetic research should aim to minimize harm while maximizing potential benefits. As researchers explore gene editing and therapies, weighing the potential moral and physical consequences on individuals and future generations is crucial.
- Justice: The distribution of benefits and burdens in genetic research must be equitable. Vulnerable populations should not bear the risks associated with research without receiving commensurate benefits. This aspect calls for careful consideration in the design of studies and genetic interventions.
"The advancement of genetic techniques such as CRISPR must be paired with ethical considerations to protect individuals and communities."
Implications of Gene Editing
Gene editing techniques, particularly CRISPR-Cas9, open up extraordinary capabilities in treating genetic diseases. These technologies allow for precise modifications at the DNA level, potentially correcting mutations that lead to disorders. However, they come with a set of ethical dilemmas that demand rigorous discourse.
Key implications include:
- Germline Modification: Editing genes in embryos can prevent inherited diseases. However, modifications can also be passed on to future generations, leading to concerns regarding unintended consequences and the concept of eugenics.
- Access and Inequality: There is a risk that gene editing technologies will be available only to those who can afford them, exacerbating existing health disparities. Ensuring equitable access is a critical ethical challenge.
In addressing these implications, ongoing dialogue among scientists, ethicists, and the public is vital to navigate the societal ramifications of gene editing safely.
Privacy Concerns in Genetic Testing
With the rise of genetic testing for various purposes, including health screening, ancestry research, and more, privacy becomes a prominent issue. Genetic information is uniquely personal and sensitive. Its handling requires a careful approach to maintain confidentiality and prevent misuse.
Several pressing privacy concerns include:
- Data Security: Genetic data is often stored digitally. Without robust security measures, there is a significant risk of breaches, which can expose sensitive information.
- Informed Consent: Participants must understand who will have access to their genetic data and how it will be used. This transparency is crucial to foster trust in genetic research and testing.
- Potential for Discrimination: There is a fear that genetic information could be used against individuals in employment or insurance contexts. Preventing genetic discrimination is essential in protecting individuals’ rights.
Future Directions in Genetic Disease Research
The field of genetic disease research is rapidly advancing. This section focuses on the future directions that this research may take. Understanding these advancements is vital for a range of stakeholders, including patients, healthcare providers, and researchers. With new technologies emerging, there are significant prospects for improving prevention, diagnosis, and treatment of genetic disorders.
Potential for Gene Therapy
Gene therapy represents one of the most promising areas in genetic disease research. This technique involves altering the genes inside a person’s cells to treat or prevent disease.
- Mechanism of Action:
- Current Applications:
- Future Considerations:
- Gene therapy works by correcting defective genes or by introducing new genes that can help treat a disease.
- This can involve replacing a harmful gene with a healthy copy or inactivating a malfunctioning gene.
- Conditions like severe combined immunodeficiency and certain inherited forms of blindness have shown successful responses to gene therapy.
- Ongoing clinical trials explore the efficiency of gene therapy in treating other conditions, including cystic fibrosis and muscular dystrophy.
- As research progresses, we can expect advancements that improve the precision and delivery of gene therapies.
- Ethical issues will also become more prominent, as decision-makers need to address concerns about accessibility and long-term effects on patients.
"The journey of gene therapy is just beginning, and its potential is boundless."
New Horizons in Genetic Counseling
Genetic counseling is evolving in significant ways. As the understanding of genetic diseases improves, so does the role of genetic counselors. They provide information and support to families at risk of inherited conditions.
- Enhanced Genetic Testing:
- Integration of Technology:
- Patient-Centric Approaches:
- The future holds promise for more sophisticated genetic tests that can provide insights into a wider range of conditions.
- This will help individuals make informed decisions about their health and the health of their offspring.
- Telehealth and online resources are changing how genetic counseling is delivered, making it more accessible to patients in remote areas.
- Integration of artificial intelligence could help in analyzing genetic data, leading to better risk assessments.
- There will be a shift toward personalized genetic counseling that considers patient preferences and values.
- Counselors will need to communicate complex genetic information in a comprehensible way, ensuring that patients feel supported in making choices about their health.