Understanding Magnetism Detectors: Principles and Trends
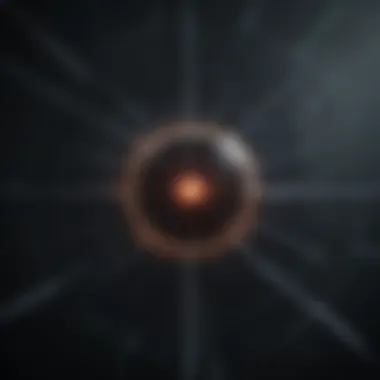
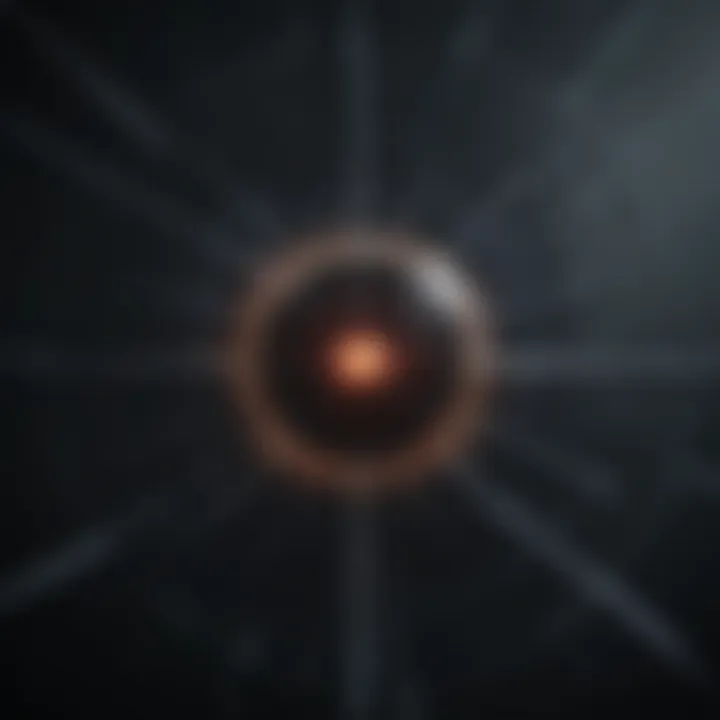
Intro
Magnetism detectors play a critical role across a wide spectrum of fields, from electronics to archaeology. This is not merely about measuring magnetic fields; it touches on principles that connect the very fabric of science itself. With the increasing complexity of technologies and methods to observe our world, understanding these devices has become not just important, but essential.
We live in a time where advancements in detection technologies are turning the once invisible forces of magnetism into understandable and measurable phenomena. As we trace the evolution of these detectors, we glimpse the future, an era where magnetic fields could be monitored with unprecedented accuracy.
In the sections that follow, we’ll delve into several key topics:
- The core principles of magnetism
- Variety of magnetism detectors and their applications in countless domains
- Recent advancements that push the boundaries of what we thought was possible.
By breaking down these intricate concepts into digestible pieces, we aim to provide not only clarity but also an appreciation for the nuanced world of magnetism and its detection.
Prologue to Magnetism
Magnetism encompasses a fascinating realm of physical phenomena that play an integral role in both our daily lives and advanced scientific explorations. Understanding magnetism is not merely an academic endeavor; it offers insights that are crucial in diverse fields ranging from geology to medical imaging. As we venture into the world of magnetism detectors, grasping the underlying principles becomes essential. These devices serve as tools that transform magnetic phenomena into measurable data that can be analyzed and utilized effectively.
Definition and Basic Concepts
Magnetism can be generally defined as the force of attraction or repulsion between objects caused by their magnetic fields. The most common examples are the simple magnets we encounter in fridges or toys. However, in the scientific context, magnetism is rooted in the behavior of electrons within atoms. When subjected to magnetic forces, certain materials exhibit unique properties.
To break it down:
- Magnetic Field: This is the area around a magnet where magnetic forces can be observed. It’s usually represented by lines, indicating the strength and direction of these forces.
- Magnetic Force: This is the actual push or pull that magnets exert on one another, along with any material that can become magnetic.
- Magnetization: The process by which a material acquires magnetic properties, due to the alignment of its atomic magnetic moments.
Adding further layers to these concepts, not all materials respond similarly to magnetic fields. They can be classified as ferromagnetic, paramagnetic, or diamagnetic, each exhibiting unique interactions with magnetic forces.
Historical Background
The journey into understanding magnetism has deep historical roots. Ancient civilizations, like the Greeks, first documented observations of magnetite—an iron ore that attracted small metal objects. Fast forward to the 19th century, where scientific giants such as Michael Faraday laid the foundation for electromagnetism, merging electricity and magnetism into a single framework.
It’s interesting to note that:
- In 1820, Hans Christian Ørsted discovered that an electric current creates a magnetic field, setting the stage for further exploration.
- James Clerk Maxwell later developed equations that describe how electric and magnetic fields interact, forming the bedrock of modern physics.
Understanding this history allows us to appreciate the monumental strides taken in magnetism research and how it influences contemporary innovations in magnetism detectors.
Fundamental Properties of Magnets
To really grasp how magnetism detectors work, it's important to get acquainted with the fundamental properties of magnets. Magnets have two poles—north and south—and they produce a magnetic field around them. The basic properties include:
- Attraction and Repulsion: Similar poles repel each other, while opposite poles attract.
- Field Lines: They emanate from the north pole and close loops at the south pole. The closer the lines are, the stronger the magnetic field.
- Magnetic Domains: In ferromagnetic materials, microscopic regions have aligned magnetic moments. When these domains align, the material can become magnetized.
"Magnets are not just about simple attraction; they embody the principles of forces and motion in a very tangible way."
These characteristics help in comprehending how various magnetism detectors are designed and function. By studying the properties above, researchers can create devices sensitive enough to detect minute changes in magnetic fields, paving the way for advancements in various fields including geology and medical diagnostics.
Types of Magnetism Detectors
Magnetism detectors are pivotal devices that measure magnetic fields, each utilizing distinctive principles and technologies. Understanding the various types of these detectors is critical in selecting the appropriate one for specific tasks. Their design and operational efficiencies can greatly impact scientific research, industrial practices, and even healthcare advancements. This section delves into five prominent types of magnetism detectors, shedding light on each one’s mechanisms, benefits, and considerations to bear in mind for effective application.
Fluxgate Magnetometers
Fluxgate magnetometers are renowned for their accuracy in measuring weak magnetic fields. These devices operate on the principle of magnetic induction and comprise two magnetic cores. When an alternating magnetic field is applied, a secondary magnetic field is induced in the core, which is then measured, providing insights into the original magnetic field's strength and direction.
This technology is especially valuable in geophysical surveys, where detecting subtle magnetic changes can lead to discoveries in mineral exploration or archaeological sites.
- Key Benefits:
- Highly sensitive, capable of detecting minute changes in magnetic fields.
- Robust design ideal for harsh environments.
Despite their high precision, fluxgate magnetometers can be sensitive to temperature fluctuations, hence requiring careful calibration to ensure accuracy in varying conditions.
Hall Effect Sensors
The Hall effect sensor is a classic in the world of magnetism detectors, operating on a straightforward principle first discovered in 1879 by Edwin Hall. When an electric current flows through a conductor in a magnetic field, a voltage—known as the Hall voltage—is generated perpendicular to both the current and the magnetic field. This phenomenon can be used to measure the intensity of magnetic fields accurately.
These sensors find applications ranging from automotive to consumer electronics. Usually compact and cost-effective, they prove beneficial in position detection and current sensing.
- Considerations:
- Limited sensitivity compared to some advanced detectors.
- Subject to saturation effects at strong magnetic fields.
Integration into smart devices has further expanded their reach, where they can help in navigation systems and magnetic switch applications.
Superconducting Quantum Interference Devices (SQUIDs)
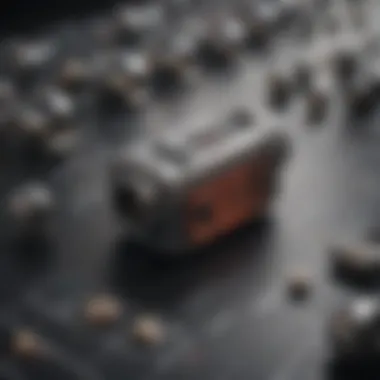
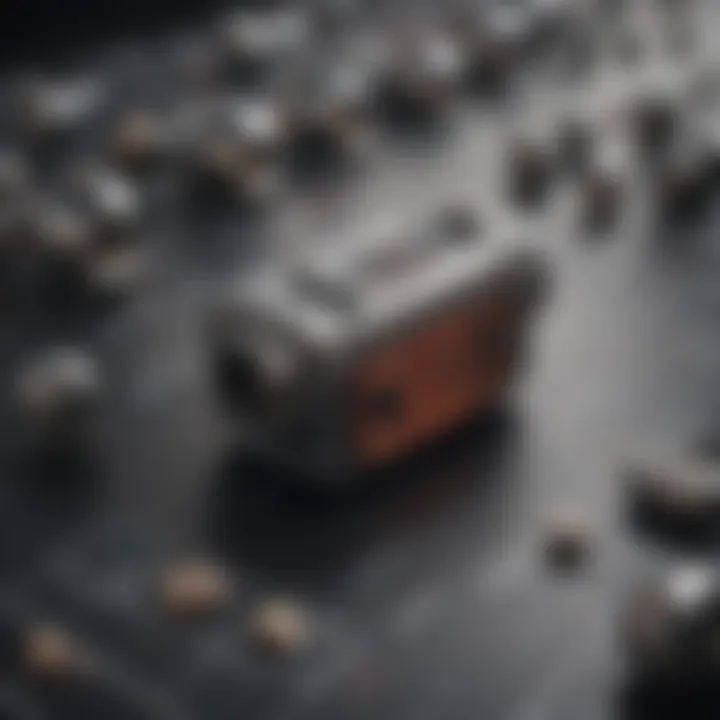
SQUIDs push the boundaries of sensitivity in magnetic field detection, being over a thousand times more sensitive than traditional sensors. Utilizing superconducting materials, these devices detect minute changes in magnetic fields through changes in electrical current due to the Josephson effect. They are ideal for applications that require extreme precision, such as in medical imaging and fundamental physics experiments.
- Noteworthy Applications:
- Magnetoencephalography (MEG) for brain imaging.
- Detection of magnetic anomalies in materials research.
A caveat is their need for cooling to maintain superconductivity, which can make them less practical for certain environments. To counteract this, significant advancements are on the horizon, aiming to create portable and more accessible SQUIDs.
Magnetoresistive Sensors
Magnetoresistive sensors have gained traction in various applications due to their adaptable nature. They exploit the magnetoresistive effect, where the electrical resistance of a material changes in response to an external magnetic field. They can be finely tuned for heightened sensitivity, making them invaluable in data storage devices and automotive applications, where detecting proximity is essential.
- Advantages:
- Compact size, providing design flexibility.
- Versatile across numerous applications, from consumer electronics to industrial machinery.
However, the sensitivity can lead these sensors to be affected by environmental factors, necessitating effective shielding in certain contexts.
Optically Pumped Magnetometers
Optically pumped magnetometers harness laser technology to achieve remarkable levels of precision in measuring magnetic fields. These devices use the principles of atomic physics, where atomic vapor is manipulated by polarized light to detect magnetic fields with hyper-sensitivity.
Ideal for applications in geophysical surveys and military sensing, optically pumped magnetometers can measure at various levels, making them indispensable in numerous scientific endeavors.
- Unique Features:
- Extremely high sensitivity, even in low field environments.
- Ability to measure over a wide frequency range.
However, the technology can be complex, requiring intricate setup and operation, which might not be feasible for all users.
In summary, each type of magnetism detector offers unique advantages and considerations that can influence their application, effectiveness, and operational demands. The careful selection of the appropriate technology is essential for achieving optimal results in varying contexts.
Principles of Operation
Understanding the principles of operation behind magnetism detectors is pivotal for grasping how these devices function in practical scenarios. At the core, these principles govern the devices' capabilities to sense and measure varying magnetic fields with precision. This section aims to shed light on the underlying mechanisms, pointing out their unique benefits and considerations that come into play when utilizing these detectors.
A firm grasp of how these devices operate can significantly influence the quality of the data one collects. This is especially true in industries where accuracy is critical, such as geophysics and medical imaging. When one learns about the principles each type of magnetism detector employs, they can better evaluate which device suits their needs best, factoring in efficiency, reliability, and cost.
How Fluxgate Magnetometers Work
Fluxgate magnetometers are renowned for their sensitivity and accuracy in measuring magnetic fields. They operate on the principle of magnetic flux, utilizing a core made of a ferromagnetic material. This core is wound with two coils. When an alternating current passes through one coil, it magnetizes the core, causing the magnetic flux to change in response to external magnetic fields.
Here’s a simplified breakdown of the process:
- The primary coil generates a magnetic field.
- External fields influence the core's saturation point.
- This interaction creates a measurable voltage in the secondary coil.
The voltages detected can be correlated to the strength and direction of the magnetic field. The beauty of fluxgate magnetometers lies in their ability to measure very small changes, making them ideal for applications in space exploration and geological surveys.
Hall Effect Phenomena Explained
The Hall effect is a fundamental principle used in many magnetism detectors today. Discovered by Edwin Hall in the late 19th century, this phenomenon occurs when a magnetic field is applied perpendicular to the flow of electric current in a conductive material.
To grasp the significance of the Hall effect:
- As charges move through the conductor, they experience a sideways force due to the magnetic field.
- This results in a voltage difference (the Hall voltage) across the material.
- The Hall voltage’s magnitude is directly proportional to the strength of the magnetic field.
This feature allows Hall effect sensors to detect magnetic fields in applications ranging from automotive to industrial environments, and they're valued for their simplicity and robustness.
Working Mechanism of SQUIDs
Superconducting Quantum Interference Devices, or SQUIDs, are remarkable tools for measuring extremely subtle magnetic fields. Their operation exploits the principles of superconductivity and quantum interference. Essentially, SQUIDs consist of two superconductors connected by a thin insulating barrier that allows for the formation of a Josephson junction.
Here’s how they work:
- When a magnetic field is applied, it influences the superconducting currents in the loop.
- This interaction results in a change in the phase of the quantum wavefunction.
- The phase shift can be translated into a change in the output voltage, allowing for precise measurements.
The incredible sensitivity of SQUIDs makes them indispensable in various scientific research areas, particularly in fields like neuroimaging and fundamental physics experiments.
Understanding Magnetoresistance
Magnetoresistance refers to the tendency of a material's electrical resistance to vary in response to an applied magnetic field. This principle is leveraged in a variety of sensors. When the material is exposed to a magnetic field, the electron trajectories experience a change, leading to a different resistance.
This effect is particularly useful in:
- Magnetic field sensing
- Memory devices (like MRAM)
Different mechanisms underlie magnetoresistance, ranging from the classical to the more intriguing giant magnetoresistance seen in certain layered materials, paving the way for novel applications in data storage and readout technologies.

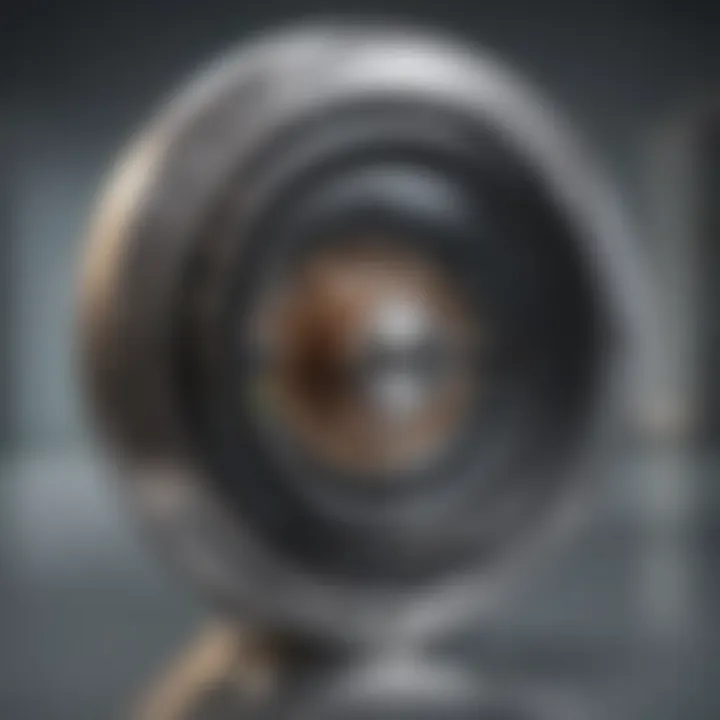
Principles Behind Optically Pumped Magnetometers
Optically pumped magnetometers operate using the principles of atomic physics and light. These devices utilize the interaction between laser light and alkali metal atoms to determine magnetic fields with high sensitivity. Here’s a closer look:
- Laser excitation: A laser is used to polarize the atoms in the vapor, aligning their spins.
- Field measurement: When exposed to a magnetic field, the precession of these spins provides data on the field strength.
The sensitivity of optically pumped magnetometers is remarkable, allowing for the detection of fields that are often below the noise level of traditional methods. Their applications range from fundamental physics to oil and gas exploration, showcasing the versatility of this technology.
In summary, the principles of operation in magnetism detectors lay down the bedrock on which this technology stands. Understanding these principles allows for better device selection and application, leading to advancements across numerous fields.
Applications of Magnetism Detectors
The applications of magnetism detectors span a wide variety of fields, each providing vital insights and capabilities. These detectors not only help in measuring magnetic fields but also enable various industries to derive meaningful data from invisible forces. Understanding the role magnets play in technology can enhance our grasp on their significance and the benefits they bring. In this exploration, we will delve into distinct applications, scrutinizing their importance and the challenges that accompany them.
Geophysical Surveys
In geophysical surveys, magnetism detectors are pivotal for mapping geological structures. Surveys done with magnetometers can trace the distribution of magnetic minerals in the earth. This method can hint at mineral deposits or even guide archaeological digs. For example, a fluxgate magnetometer allows geophysicists to measure minute magnetic fluctuations, revealing hidden features underground. Such insights not only assist in resource exploration but also mitigate the risks associated with mining operations.
- Key Benefits:
- Considerations:
- Accurate location of resources
- Detection of environmental hazards
- Requires proper calibration for accuracy
- Interpretation of data can be complex
Industrial Monitoring
In the arena of industrial monitoring, magnetism detectors contribute to ensuring safety and efficiency. By employing Hall Effect sensors or magnetoresistive sensors, industries can track machinery and equipment performance. These sensors monitor magnetic fields that can signal wear or failure in mechanical components. For example, a failing motor might exhibit a significant drop in magnetic field strength. This real-time data aids in predictive maintenance, reducing downtime and saving costs.
- Key Benefits:
- Considerations:
- Enhances operational safety
- Enables timely maintenance decisions
- Initial setup costs can be high
- Staff training might be necessary
Medical Imaging Techniques
Magnetism detectors also find essential roles in medical imaging, particularly in MRI technology. MRI machines utilize strong magnetic fields to provide detailed images of the body's internal structures. The precision of these detectors allows for high-resolution imaging, which can aid in the early diagnosis of diseases. Moreover, advancements in optically pumped magnetometers are pushing the frontiers of sensitivity, promising enhanced imaging capabilities in the future.
"The magnetic resonance imaging technology exemplifies how magnetism detection has made an indelible mark on healthcare."
- Key Benefits:
- Considerations:
- Non-invasive imaging
- Early detection of health issues
- Limited access in certain regions
- Safety concerns for patients with certain implants
Security Applications
In the realm of security, magnetism detectors help secure both public and private spaces. They can detect unauthorized movement of magnetic objects in sensitive areas, serving as a first line of defense against theft or tampering. For instance, magnetic sensors are often integrated into alarm systems to enhance surveillance measures. Their ability to provide real-time notifications can be a game changer in maintaining security.
- Key Benefits:
- Considerations:
- Immediate alert systems
- Versatile application in various settings
- False alarms can occur
- Requires regular maintenance for optimal operation
Research and Development
Lastly, the application of magnetism detectors in research and development cannot be overstated. Researchers utilize various detectors to conduct experiments in fields such as physics and materials science. These measurements help in the exploration of new materials with unique magnetic properties, paving the way for innovations like improved data storage devices or advanced medical treatments. The integration of superconducting quantum interference devices (SQUIDs) is particularly notable due to their sensitivity.
- Key Benefits:
- Considerations:
- Informs groundbreaking research
- Facilitates technological advancements
- High costs involved in technology development
- Need for specialized knowledge to operate effectively
The breadth of applications for magnetism detectors highlights their critical role across various sectors. By fully grasping their advantages and challenges, industry professionals and researchers can better harness this technology for future developments.
Challenges in Magnetism Detection
In the realm of magnetism detection, challenges are more than just speed bumps on the road to progress; they're significant hurdles that researchers and industry professionals must navigate. These obstacles can affect how detectors work, their reliability, and their applicability in various fields. Understanding these challenges is crucial not only for those developing magnetism detection technology but also for end-users who depend on accurate measurements in applications ranging from geophysical surveys to medical imaging. By identifying and addressing these challenges, the community can better harness the benefits of magnetism detection, making its virtues more apparent in harnessing the Earth's magnetic forces, enriching our technological innovations.
Sensitivity Limitations
One of the most pressing issues in magnetism detection is sensitivity. Sensors need to detect minute changes in magnetic fields, especially in applications like geological surveying or monitoring brain activity. Unfortunately, the sensitivity of some detectors can be compromised due to various factors, which could lead to inaccurate readings.
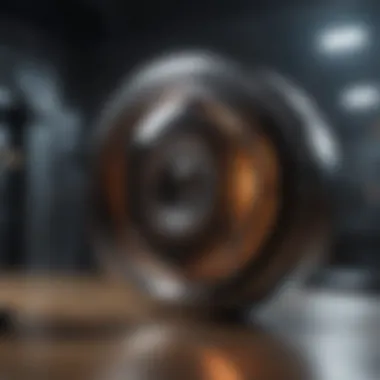
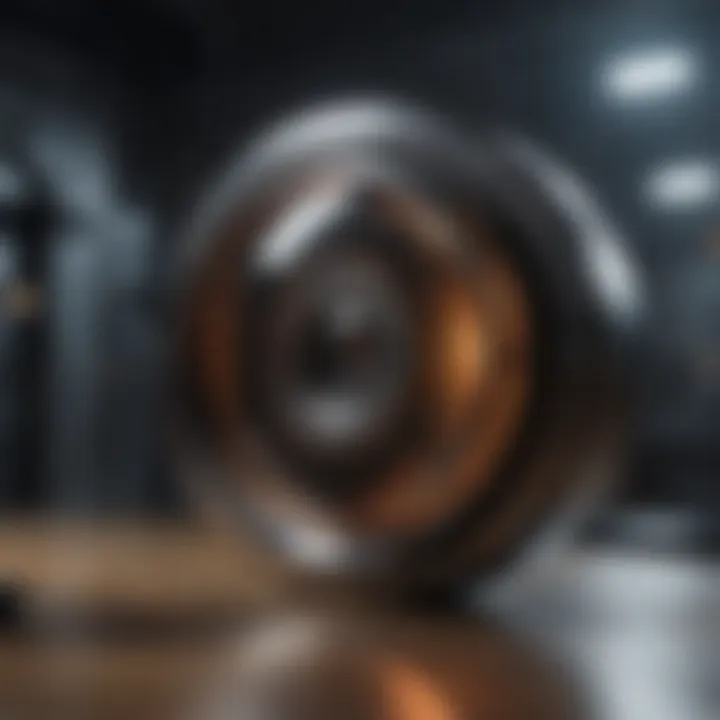
For instance, magnetoresistive sensors, though widely used, may not detect lower magnetic fields effectively. To illustrate, consider an example of a geophysicist trying to identify mineral deposits below the Earth's surface. If the detector lacks the appropriate sensitivity, vital information might remain undiscovered. To combat this, ongoing research has focused on enhancing the sensitivity of these devices, employing techniques such as advanced materials and calibration methods.
Environmental Interference
Another challenge faced by magnetism detectors is environmental interference. The presence of external magnetic fields—such as those generated by industrial machinery or even the Earth's natural magnetic fluctuations—can affect readings. Imagine a researcher conducting magnetic resonance imaging in a bustling urban hospital. The competing electronic devices and stray magnetic fields can skew results, leading to incorrect diagnoses.
Thus, it becomes imperative to develop techniques that can filter out this interference. Shielding technologies and advanced signal processing techniques are on the horizon, but they are not foolproof. Hence, balancing detector sensitivity with environmental robustness remains a puzzling but crucial endeavor.
Calibration and Accuracy Issues
Calibration is vital for ensuring the accuracy of magnetism detectors. Unfortunately, the process can be cumbersome and fraught with complexities. A poorly calibrated device can lead to results that are as useful as a screen door on a submarine. Achieving and maintaining precise calibration requires a series of checks, standards, and periodic maintenance.
For example, in medical applications, slight differences in calibration can mean the world of difference in patient care. Doctors rely on accurate imaging modalities to make diagnoses. If a detector malfunctions or drifts out of calibration, it may present a false reading, which could lead to misinterpretation of a patient's condition. Thus, ongoing research into simpler and more robust calibration techniques is crucial.
Cost and Accessibility Factors
Lastly, there are always cost factors to consider in any technology. High-precision magnetism detectors, especially advanced types like superconducting quantum interference devices, possess a hefty price tag, which can make them less accessible to smaller research institutions or developing countries.
In contexts where budget constraints exist, the challenge is to find affordable alternatives that do not compromise accuracy or reliability. As the competition in the market heightens, it's anticipated that manufacturers will innovate more cost-effective solutions without sacrificing performance—a tall order but necessary in making these powerful tools widely available.
"As technology evolves, addressing these challenges will determine how effectively we can use magnetism detection across various fields, including healthcare, geology, and industrial monitoring."
Navigating the challenges in magnetism detection demands not only technical ingenuity but also a collaborative effort across sectors to drive research and development toward surmounting these barriers.
Future Trends in Magnetism Detection
In today's rapidly evolving technological landscape, the future of magnetism detection is brimming with potential. This section highlights several noteworthy developments, addressing their significance and broader implications for various sectors. By focusing on advancements in sensor technology, cross-technology integration, novel applications, and the sustainability of magnetism detection methods, we get a glimpse into a more connected and efficient future.
Advancements in Sensor Technology
Sensor technology is consistently marching ahead, and magnetism detectors are no exception. Innovations in materials science, along with improvements in design and fabrication processes, are leading to enhanced sensitivity and miniaturization of detectors. For instance, researchers are exploring the potential of graphene-based sensors. Due to their exceptional properties, these sensors could lead to higher precision in detecting minute magnetic fields.
Moreover, new fabrication techniques are allowing for the incorporation of artificial intelligence in calibration processes, which dramatically improves the accuracy of readings from sensors. With applications in fields ranging from industrial applications to medical imaging, advanced sensors are crucial for the next wave of discoveries and efficiencies.
- Increased sensitivity offers:
- Detection of weaker magnetic fields
- Enhanced spatial resolution
- Greater accuracy in data interpretation
Integration with Other Technologies
As we progress, the fusion of magnetism detection technology with other fields appears inevitable. The intersection of magnetism detectors with Internet of Things (IoT) solutions exemplifies this trend. This integration enables real-time data collection and analysis, improving monitoring capabilities in various sectors, including logistics, healthcare, and environmental management.
Furthermore, implementing magnetism detection in robotics could revolutionize how these machines navigate and interact with their environment. Precision agriculture is another promising area, where magnetism detectors can be utilized to assess soil health and optimize resource use. Their role is growing alongside a surge in data-driven decision-making across industries.
Emerging Applications in Different Fields
The potential applications of magnetism detectors are expanding like a kid's imagination. Besides traditional fields like geophysics and industrial monitoring, novel uses are cropping up in unexpected areas. In healthcare, for instance, magnetism detectors can enhance magnetic resonance imaging (MRI) and improve drug delivery mechanisms.
In the realm of national security, these detectors can aid in detecting concealed objects, making them invaluable in enhancing safety measures at public events. As interdisciplinary collaboration becomes the norm, the applications could continue to grow and evolve, fostering new breakthroughs that push the boundaries of what's possible.
- Some emerging applications include:
- Monitoring environmental pollutants
- Enhancing navigation systems for autonomous vehicles
- Forensic science applications in crime scene investigations
Sustainability in Magnetism Detection
Sustainability remains at the forefront of technological discussions today. As the focus on reducing environmental impact intensifies, the magnetism detection sector is also vying to contribute positively. Research into sustainable materials for device fabrication is already underway. For example, developing eco-friendly magnetic materials can help mitigate some environmental costs associated with traditional manufacturing processes.
Moreover, the push for energy-efficient systems translates into lower operational costs and a reduced carbon footprint. With renewable energy solutions accessing magnetism detection for optimizing energy use and improving grid management, sustainability can intertwine seamlessly with magnetism technology.
"The future of magnetism detection is not solely about advancements; it’s about creating a sustainable and integrated approach to technology."
This narrative of innovation, integration, and sustainability paints a hopeful picture for the future of magnetism detection. Continuous exploration and adaptation will undoubtedly shape these tools into indispensable instruments across various fields, enriching both human lives and the environment.
Culmination
In the realm of science and technology, the understanding of magnetism detectors stands as a cornerstone for numerous applications. This article sheds light on the intricacies of magnetism, encompassing the principles, types, and technologies of these detectors. The importance of concluding this exploration cannot be understated, as it not only encapsulates the key findings but also paves the way for future discussions and advancements in the field.
Summary of Key Insights
Throughout the article, a variety of aspects were uncovered, each contributing to a well-rounded perspective on magnetism detectors. The following points serve as a synthesis of vital insights:
- Diverse Applications: Magnetism detectors stretch far beyond simple measurements. From geophysical surveys unearthing the secrets of our planet to medical imaging advancements that improve diagnostics, their utility is vast.
- Technological Evolution: The field is marked by rapid technological advancements. From traditional fluxgate magnetometers to cutting-edge SQUIDs, innovation drives efficiency and sensitivity.
- Current Challenges: Sensitivity, environmental factors, and calibration hurdles are ongoing challenges that necessitate continual refinement in detection methods.
This blend of historical context and modern application underscores the value of magnetism detectors, showcasing how they are integral to various scientific explorations and practical implementations.
The Role of Magnetism Detectors in the Future
Looking ahead, magnetism detectors are poised to play an even more significant role in multiple fields. The advent of new technologies and methodologies will likely lead to:
- Enhanced Sensitivity: As research continues, we can expect to see a marked improvement in the sensitivity and accuracy of detection methods. This will allow for more precise measurements even in challenging environments.
- Cross-discipline Integration: With the integration of AI and machine learning in data interpretation, the effectiveness of magnetism detectors will soar. Their role in automation could transform industries such as healthcare, defense, and environmental monitoring.
- Sustainable Practices: The commitment to sustainability will drive innovations in magnetism detection, with a focus on energy-efficient designs and eco-friendly materials.
In summary, the evolution and ongoing research into magnetism detectors hold promise not only for scientific inquiry but also for real-world applications that can benefit society at large. As we deepen our understanding and refine the technologies involved, the potential contributions of these tools can scarcely be overstated.