Understanding the Function and Impact of Protease Enzymes
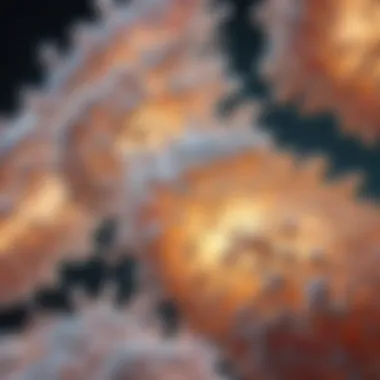
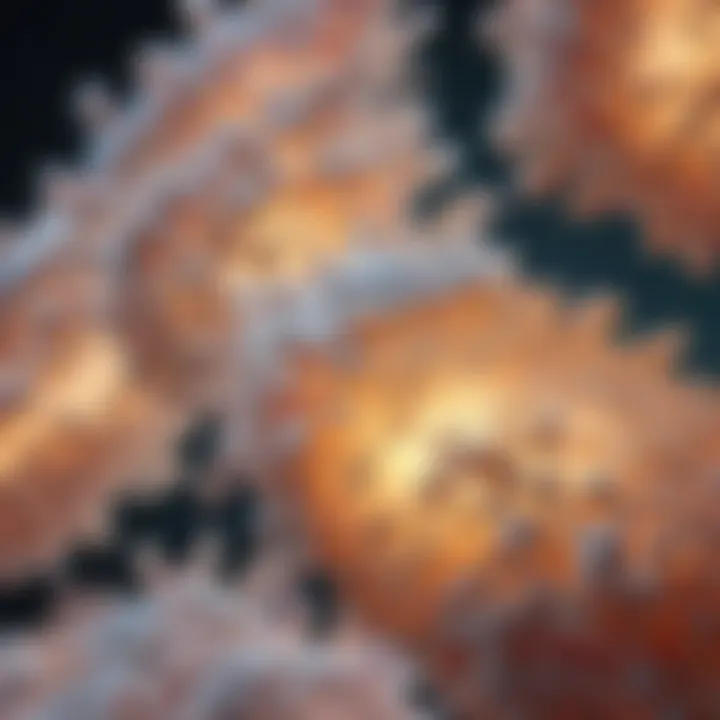
Intro
Protease enzymes are vital biological catalysts that perform essential functions in various living organisms. These enzymes break down proteins into smaller peptides or amino acids, a process known as proteolysis. The role of proteases extends from digestion to cell signaling, regulation of cell cycles, and immune responses. Understanding their mechanisms can illuminate pathways that are critical for health, influencing the development of therapies for numerous diseases.
This article will explore protease enzymes in detail. We will discuss their types, regulatory mechanisms, and applications in industries like biotechnology and pharmaceuticals. By examining both recent advances and traditional understanding, we aim to shed light on the complexities of protease functions in biological systems.
Recent Advances
Latest Discoveries
Research into protease enzymes has unveiled remarkable findings, particularly regarding their functions in health and disease. For example, scientists have identified specific proteases that influence cancer progression. Certain proteases can degrade extracellular matrix proteins, facilitating tumor spread. Conversely, other proteases are being researched for their potential inhibitory roles in cancer metastasis. This duality points to the complex nature of protease action, which can both promote and inhibit disease processes.
Technological Innovations
Recent technological advancements have propelled the study of proteases. Techniques such as CRISPR genetic editing have been applied to create precise protease inhibitors. Additionally, high-throughput screening methods have enabled researchers to evaluate thousands of potential inhibitors rapidly. These tools enhance understanding of protease functions and pave the way for novel therapeutic approaches to combat diseases.
Mechanisms of Action
Proteases operate through various mechanisms, including serine, cysteine, aspartic, and metalloproteases. Each type has unique active sites that determine how they interact with specific substrates. For instance, serine proteases utilize a serine residue in their catalytic mechanism, while metalloproteases depend on metal ions to activate water molecules for hydrolysis.
Understanding these mechanisms is crucial for designing drugs that can either inhibit or enhance protease activity. Researchers are constantly examining interactions at the molecular level to develop targeted therapies.
Applications of Proteases
Proteases find applications in diverse fields, such as:
- Medicine: Inhibitors are explored for treating cancer, inflammation, and other conditions.
- Food Industry: Proteases are used in cheese making, meat tenderization, and as digestive aids.
- Biotechnology: They are essential in the production of biofuels and in bioremediation processes.
The adaptability of proteases across these sectors underscores their importance in both natural and industrial processes.
Finale
The exploration of protease enzymes reveals a multifaceted role in biological systems and practical applications. From their involvement in fundamental physiological processes to their innovative applications in various industries, proteases are an area of immense research and potential. As we continue to unravel their complexities, the implications for health and industry become increasingly significant.
Preface to Protease Enzymes
Protease enzymes are fundamental biological catalysts that facilitate the breakdown of proteins into smaller peptides or amino acids. Their importance cannot be overstated, as they are involved in various physiological processes that sustain life. In this article, we will explore the various dimensions of proteases, their functions, classifications, and implications in health and industrial applications. Understanding these aspects is critical for students, researchers, educators, and professionals in life sciences and biotechnology fields.
Definition of Proteases
Proteases, also known as proteinases or peptidases, are enzymes that catalyze the hydrolysis of peptide bonds in proteins. This activity is crucial for protein metabolism, allowing for the recycling of amino acids and the regulation of biochemical pathways. Proteases are classified based on their structure, catalytic mechanism, and specificity. This diversity allows them to fulfill distinct and essential roles within various biological systems.
The general mechanism of action involves the enzyme binding to a substrate protein, which is then cleaved at specific sites. The resulting fragments can have various fates, from further degradation to function as signaling molecules. Understanding proteases involves recognizing their specificity toward different substrates and the conditions under which they catalyze reactions.
Historical Context and Discovery
The study of proteases has a rich history that traces back to the 19th century. Early observations noted enzymatic activity in digestive processes, leading scientists to investigate the proteins involved. In this context, the discovery of pepsin by Theodor Schwann in 1836 marked a significant milestone. Pepsin was identified as a gastric enzyme involved in protein digestion.
Further advancements in biochemistry illuminated the complexities of these enzymes. In the early 20th century, researchers like Emil Fischer contributed to understanding enzyme specificity and the active site concept. The development of tools such as X-ray crystallography in the later half of the century allowed scientists to visualize enzyme structures, providing deeper insights into their functionality. Today, modern proteomics employs various techniques to study protease activity in complex biological systems, demonstrating their essential role in health and disease.
Biological Functions of Proteases
Protease enzymes hold paramount importance in various biological processes. Understanding their functions helps to comprehend the intricate mechanisms of life at a molecular level. These enzymes are primarily responsible for the catalysis of peptide bonds, facilitating the degradation of proteins into smaller peptides or amino acids. This catalytic efficiency is essential for several key physiological functions that range from metabolism to immune response. The versatility of proteases implies they play roles not only in healthy physiological states but also in various pathological conditions.
Protein Turnover and Regulation
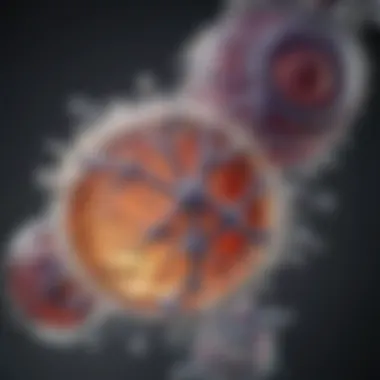
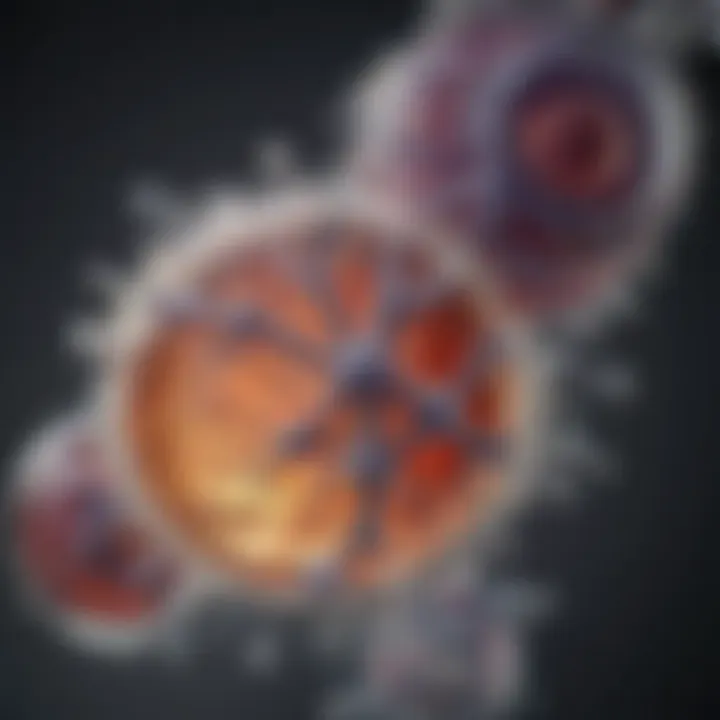
One of the most critical functions of proteases is protein turnover. This process involves the continuous synthesis and degradation of proteins, which is essential for cellular health. Proteins perform specific roles in cells, and their activity requires regulation. Protease enzymes are fundamental in maintaining the balance by removing damaged or misfolded proteins. This ability to regulate protein levels ensures that cellular functions operate optimally.
For instance, during periods of stress or nutrient deprivation, proteases can facilitate the degradation of cellular components to provide substrates for necessary metabolic pathways. This process is known as autophagy and is crucial for cellular homeostasis.
In addition, the activity of proteases is tightly regulated through various mechanisms including the presence of inhibitors and covalent modifications. This ensures that proteolysis occurs only when needed, thus preventing cellular damage that could result from uncontrolled protein degradation.
Role in Cellular Signaling
Proteases also play a significant role in cellular signaling pathways. They contribute to the activation and deactivation of signaling molecules such as hormones and growth factors. For example, many proteases are involved in the activation of proenzymes, turning them into their active forms.
Moreover, proteases can cleave receptors that are part of signaling pathways, thereby modulating cellular responses. This regulatory effect is crucial in various physiological processes such as cell proliferation, apoptosis, and immune responses. The dysregulation of this function can lead to diseases, notably cancer, where inappropriate signaling pathways can lead to uncontrolled cell growth.
"Proteases act like molecular switches, controlling crucial cellular processes through their activity."
Participation in Digestion
The digestive process heavily relies on proteases. These enzymes are secreted by glands in the digestive system to break down dietary proteins into peptides and amino acids. This breakdown is essential for nutrient absorption and plays a vital role in sustaining life. Specific types of proteases can be found at various points throughout the digestive tract, each tailored to act optimally under different conditions.
For instance, pepsin is a well-known protease active in the acidic environment of the stomach. It begins the digestion of proteins, leading to smaller peptides that can be further processed in the intestines by other proteases, such as trypsin and chymotrypsin. The efficiency of these enzymes ensures that nutrients are available for absorption and utilization by the body.
Types of Proteases
The classification of proteases is essential for understanding their diverse roles in biological systems. Each type of protease has unique characteristics, mechanisms, and functions, determining how they interact with substrates and contribute to various physiological processes. Knowing the specific types of proteases allows researchers, students, and professionals to better appreciate their relevance in enzyme activity, health implications, and industrial applications.
Classification Based on Catalytic Mechanism
Proteases can be classified by their catalytic mechanisms. This classification includes serine, cysteine, aspartic, and metalloproteases. Each group operates using distinct sites and modes of action that make them suitable for specific functions within biological systems. Understanding these mechanisms can help in the development of inhibitors and activators for therapeutic purposes and aid in the design of applications in biotechnology.
Serine Proteases
Serine proteases are characterized by their use of a serine residue in the active site for catalysis. Found in various organisms, they play critical roles in digestion, immune response, and blood coagulation. Examples include trypsin and chymotrypsin. These enzymes work efficiently to hydrolyze peptide bonds, illustrating the importance of their specificity and rate of reaction. Their role in physiological processes often attracts significant interest in drug design and therapeutic interventions.
Cysteine Proteases
Cysteine proteases utilize a cysteine residue in their active site, making them crucial in various biological functions. They are involved in protein degradation, cell signaling, and the progression of diseases, including cancer and neurodegeneration. Papain is a well-known example. Cysteine proteases can also be found in many pathogens, which makes them target candidates for pharmaceutical research.
Aspartic Proteases
Aspartic proteases, which use two aspartate residues for catalysis, are known for their role in digestion and protein turnover. Renin and pepsin are prominent members of this group. These enzymes are vital in pH-dependent environments, illustrating their versatility. Their involvement in disease mechanisms has led to an increase in the focus of research aimed at understanding their functions.
Metalloproteases
Metalloproteases require metal ions, typically zinc, for their activity, which facilitates the hydrolysis of peptide bonds. These enzymes participate in extracellular matrix remodeling and are of great interest in research related to cancer metastasis and tissue repair. Matrix metalloproteinases are key examples. The regulation of their activity presents both challenges and opportunities in therapeutic applications, particularly in cancer treatment and wound healing.
Be aware that understanding types of proteases provides a foundation for exploring their mechanisms and applications. Each type reveals insights into the nuanced roles these enzymes play across fields such as health and industry.
Mechanism of Action
The mechanism of action of protease enzymes is crucial for understanding how these proteins interact with substrate molecules to execute their biological roles. Proteases play an essential role in various physiological processes, from the digestion of nutrients to the regulation of cellular functions. This section elucidates the key elements of protease action, emphasizing the complex interactions that dictate their efficacy and context of use.
Enzyme-Substrate Interaction
Enzyme-substrate interaction is the first step in the proteolytic process. Proteases recognize specific peptide bonds within substrate proteins. This selectivity arises from the structural compatibility between the enzyme's active site and the substrate. The active site is a unique pocket shaped to fit the substrate molecule, akin to a key fitting into a lock.
Once the substrate binds to the enzyme, it forms an enzyme-substrate complex. This complex stabilizes the interaction, allowing the enzymatic reaction to occur. Several factors influence this interaction, such as the pH level, the presence of cofactors, and temperature.
Catalytic Cycle of Proteases
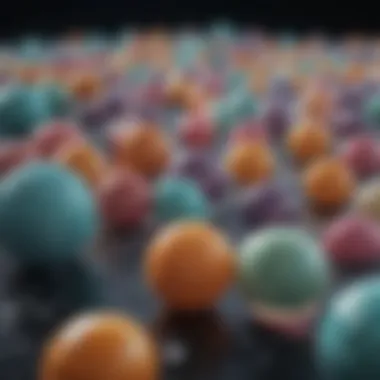
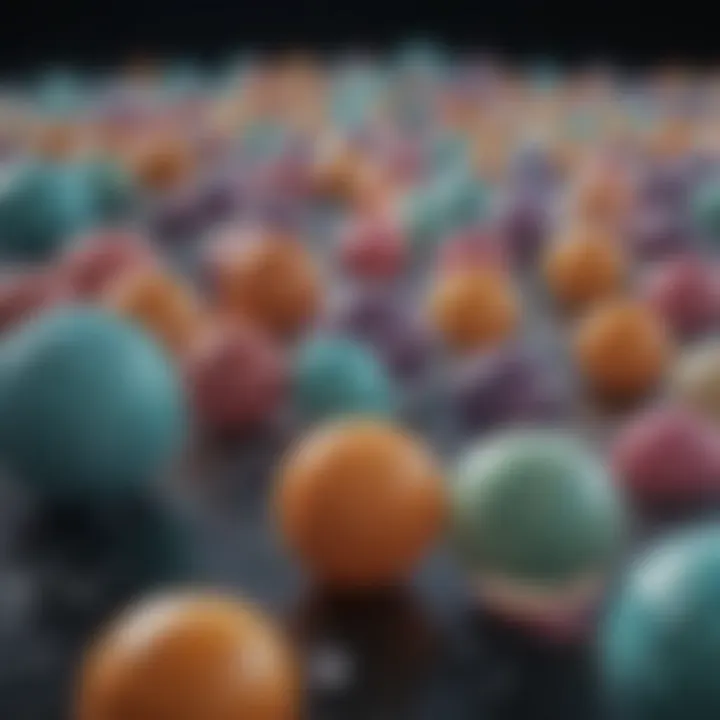
The catalytic cycle refers to the series of steps that proteases undergo to catalyze the hydrolysis of peptide bonds. After the formation of the enzyme-substrate complex, the protease facilitates the breaking of the peptide bonds by adding water molecules, a process known as hydrolysis. This transformation results in the release of smaller peptide fragments or individual amino acids.
Typically, the catalytic cycle can be summarized in these stages:
- Substrate Binding: The substrate fits into the active site of the enzyme.
- Transition State Formation: The enzyme stabilizes the transition state, facilitating bond cleavage.
- Hydrolysis: Water molecules play a significant role in breaking peptide bonds.
- Product Release: The resulting peptide fragments are released from the enzyme, which is then free to interact with new substrates.
This cyclic process illustrates the efficiency and speed of proteases, often catalyzing reactions in milliseconds.
Factors Affecting Protease Activity
Multiple factors can significantly impact protease activity, altering how effectively they function in biological systems. Key elements include:
- pH Levels: Each protease has an optimal pH that maximizes its activity. Deviations can lead to reduced enzymatic efficiency.
- Temperature: Enzymes generally have a temperature range where they operate best. Extreme temperatures can denature proteins, resulting in loss of function.
- Cofactors and Inhibitors: Certain ions or small molecules may enhance or inhibit activity. For instance, calcium ions are known to assist the catalytic action of specific proteases, while inhibitors can block active sites, preventing substrate binding.
Understanding these factors is essential, particularly in research and industry, where controlling protease conditions is vital for desired outcomes.
Overall, the mechanism of action reveals the intricate nature of proteases. Their ability to recognize substrates, catalyze reactions, and respond to environmental factors underpins their roles across various biological processes and applications.
Proteases in Health and Disease
Proteases play a pivotal role in human health and disease processes. Their activity affects many physiological functions, which include digestion, immune response, and cell signaling. Understanding how proteases impact health is essential for identifying potential therapeutic strategies and improving disease management. Proteases can have both beneficial and detrimental effects in biological contexts, highlighting the need for careful regulation of their activity.
Proteases and Immune Response
Proteases are critical to the immune response. They contribute to the processing of antigens, which are foreign substances that trigger an immune response. Proteolytic enzymes, such as caspases, play a role in apoptosis, or programmed cell death, a process crucial for eliminating infected or damaged cells.
In addition, certain proteases can modulate cytokine activity, influencing inflammation and immune cell recruitment. The balance of activity between different proteases can determine the outcome of immune responses. Overactivity of specific proteases can lead to excessive inflammation, while underactivity might result in insufficient immune activation. Vigilance in understanding this balance is essential for developing interventions in autoimmune disorders and allergies.
Role in Cancer Biology
In cancer biology, proteases are involved in various stages, from tumor initiation to metastasis. Tumor cells can secrete proteases, such as matrix metalloproteinases, to degrade extracellular matrix components. This degradation enables the invasion of surrounding tissues and facilitates metastasis to distant sites in the body.
Moreover, certain proteases regulate the bioavailability of growth factors within the tumor microenvironment, which can further promote tumor growth. The understanding of these mechanisms can lead to innovative therapeutic approaches, including the development of protease inhibitors. Targeting specific proteases may slow down tumor progression and reduce metastasis, offering hope for more effective cancer treatment strategies.
Proteases in Infectious Diseases
Proteases also have a significant role in infectious diseases. Many pathogens, including viruses and bacteria, exploit proteases to facilitate their life cycles. For instance, the human immunodeficiency virus (HIV) relies on its own protease for the maturation of viral particles. Inhibition of this protease is a critical part of antiretroviral therapies aimed at managing HIV infection.
In addition to viral infections, bacterial proteases can modulate host immune responses. They may disrupt signaling pathways, allowing pathogens to evade detection and promote their survival within the host. Understanding these interactions provides insights for potential therapeutic approaches in infectious disease management, helping to inform vaccine and drug development efforts.
"The intricate relationship between proteases and health highlights the need for ongoing research to delineate their roles in various biological systems. Their dual nature can result in both therapeutic opportunities and challenges in managing diseases."
The multifaceted roles of proteases in health and disease underline their relevance. Further research into the specific mechanisms by which proteases act in these contexts is essential for advancing medical science and improving treatment outcomes.
Industrial and Biotechnological Applications
Protease enzymes have significant relevance across various industries, serving as essential catalysts in biochemical processes. Their versatility and efficiency make them valuable in fields such as food production, pharmaceuticals, and waste management. This section delves deeper into these avenues, highlighting the impact and considerations of protease usage in industrial and biotechnological contexts.
Use in Food Industry
Proteases find extensive application in the food industry. They are pivotal in many processes like protein hydrolysis, which converts food proteins into smaller peptides and amino acids. This conversion enhances flavor, texture, and digestibility of food products.
For example, in cheese production, specific proteases are responsible for curd formation by breaking down casein proteins. Additionally, in meat tenderization, proteases like papain or bromelain contribute to the softness of meat by cleaving tough muscle fibers.
Key benefits of utilizing proteases in food production include:
- Improved taste and aroma of food products.
- Enhanced nutritional value through better amino acid availability.
- Cost-effective methods to produce high-quality foods.
- Increased shelf life of products due to modified proteins.
However, considerations such as optimal temperature and pH for enzyme activity must be taken into account to ensure efficiency and quality control in food manufacturing.
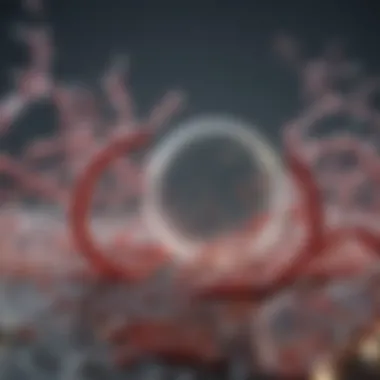
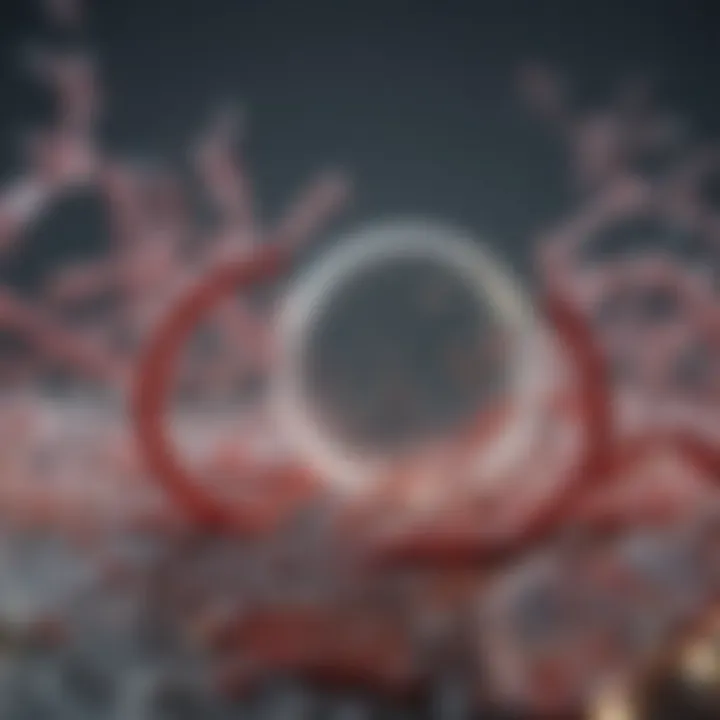
Application in Pharmaceuticals
In the pharmaceutical sector, proteases play critical roles in drug development and therapeutic interventions. They are utilized in designing drugs aimed at specific proteolytic pathways involved in diseases. Moreover, protease inhibitors can serve as vital treatments for conditions such as HIV and cancer.
Proteases are also important in drug delivery systems, where they can facilitate the release of active pharmaceutical ingredients.
Some prominent applications include:
- Utilization in cancer therapy to target proteolytic enzymes overexpressed in tumor cells.
- Development of antiviral medications that inhibit proteases essential for viral replication.
- Engineering of enzyme-based diagnostics that improve disease detection methods.
Despite the therapeutic potential, careful research is necessary. Not all protease interactions are beneficial, and unregulated inhibitors can cause adverse effects, emphasizing the need for ongoing studies in this field.
Proteases in Waste Management
Protease enzymes also play a role in waste management, particularly in the degradation of organic materials. Their ability to break down proteins makes them useful in various waste treatment processes, including the decomposition of organic waste in landfills and wastewater treatment plants.
By facilitating the breakdown of proteins, proteases contribute to:
- Reduction of waste volume by accelerating the decomposition process.
- Mitigation of foul odors associated with decomposing organic matter.
- Improvement of nutrient recovery from bio-waste, supporting composting efforts.
The integration of proteases into waste management practices supports sustainable development and environmental conservation strategies.
Overall, the multifaceted applications of proteases illustrate their importance in various industrial processes. It is essential to leverage their capabilities while being mindful of the conditions that regulate their activity. This balance will enhance efficacy and sustainability across sectors.
Regulation of Protease Activity
The regulation of protease activity plays an essential role in various biological processes. Maintaining the balance of protease enzymes is critical for cellular functions, preventing dysregulation that can lead to diseases. The activity of proteases must be finely controlled to ensure they function appropriately in physiological contexts. Differences in the regulation mechanisms can determine the specific roles proteases play in processes such as digestion, immune response, and cell signaling.
Inhibitors and Activators
Protease activity is modulated by a variety of inhibitors and activators. These molecules can either suppress or enhance the activity of proteases, thus directing the pathways in which they are involved.
Inhibitors can be classified into several categories:
- Competitive inhibitors: These bind to the active site of proteases, preventing substrate binding.
- Non-competitive inhibitors: These attach to other parts of the enzyme, changing its shape and function without directly blocking the active site.
- Reversible inhibitors: These can detach from the enzyme, allowing activity to resume.
- Irreversible inhibitors: These form permanent bonds with the enzyme, leading to a lasting decrease in activity.
On the other hand, activators serve to enhance the activity of proteases. They may increase the availability of substrates or alter the conformation of the protease, making it more effective in catalyzing reactions. Both inhibitors and activators are critical for maintaining homeostasis, as their balance determines the functional state of protease activity in living organisms. As a result, understanding these regulatory interactions is vital for developing therapeutic strategies that target specific proteases in diseases.
"Understanding the balance of proteases between their activated and inhibited states provides insight into their functional versatility in biological systems."
Impact of Cellular Conditions
Cellular conditions significantly affect protease activity. Factors such as pH, temperature, and ion concentrations can alter the stability and function of proteases. For example, most proteases are sensitive to pH changes; they often function optimally within a narrow pH range. Enzymes such as pepsin, active in the acidic environment of the stomach, differ in pH preference from those operating in more neutral conditions in the intestine.
Temperature can also play a crucial role. Elevated temperatures may enhance kinetic energy, increasing the reaction rates of proteases, but excessive heat can denature enzymes, leading to loss of function. Likewise, ionic strength and the presence of specific substrates or cofactors can influence protease activity and stability.
These cellular conditions intertwine with protease regulation, as cells adapt to their environment by shifting the balance of activators and inhibitors in response to changing physiological needs. Therefore, examining these impacts contributes to our understanding of protease dynamics and their roles in health and disease.
Research Trends and Future Directions
Research focused on protease enzymes has witnessed significant growth in recent years, reflecting their crucial role in both biological and industrial applications. The exploration of novel proteases and their diverse functionalities is essential for advancing our understanding of various physiological processes. Furthermore, identifying how these enzymes interact with other biomolecules can lead to breakthroughs in treatment and prevention of diseases.
Innovations in Protease Research
Innovations in protease research are unfolding across various scientific disciplines. One key area of development involves the use of genomics and proteomics technologies. These approaches enable scientists to uncover previously uncharacterized proteases and understand their interactions at the molecular level. Advanced sequencing techniques facilitate the identification of new protease genes, which can be particularly advantageous in agricultural and biotechnological contexts.
Additionally, structural biology is providing insights into the intricate mechanisms by which proteases operate. Techniques such as X-ray crystallography and cryo-electron microscopy allow researchers to visualize protease structures at an atomic resolution. Understanding these structures is vital to designing specific inhibitors that can modulate protease activity, which has implications for various therapeutic applications.
Moreover, bioinformatics tools are emerging as a powerful ally in protease research. These tools can analyze large datasets, predicting protease functions based on sequence homology, and help map the dynamic roles of proteases in complex cellular environments. Such innovations are pivotal in deciphering the multifaceted roles of proteases in pathways implicated in diseases.
Potential for Therapeutic Interventions
The potential for therapeutic interventions involving proteases is vast and represents a promising frontier in medical science. Proteases have been implicated in many disease processes, including cancer, cardiovascular diseases, and neurodegenerative disorders. By precisely targeting specific proteases, novel treatment strategies can be developed to combat these conditions more effectively.
For instance, the role of proteases in cancer biology, particularly in tumor progression and metastasis, presents an opportunity for intervention. Inhibitors designed to target these enzymes could effectively halt the spread of cancerous cells, improving patient outcomes. Similarly, addressing the dysregulation of proteases in inflammatory diseases could lead to new anti-inflammatory drugs.
Additionally, proteases are being explored as potential therapeutic agents in managing infectious diseases. For example, proteases from pathogens can be targeted to prevent their replication, offering a new approach to treating bacterial and viral infections.
The future of protease research holds promise, with ongoing studies expanding our knowledge and opening avenues for innovative treatments.